Abstract
Ligands are an imperative part of targeted drug delivery systems, and choosing a ligand with high affinity is a subject of considerable interest. In this study, we first synthesized a 12-residue peptide (TK) that interacts with integrin α6β1 overexpressed on colonic cancer cells. The molecular binding affinity assay indicated that TK had a high binding affinity for integrin α6β1. The results of cellular and tumor spheroid uptake suggested that TK peptide not only increases Caco-2 cells uptake, but also effectively increases penetration of the tumor spheroids. TK-conjugated PEG-PLA was synthesized to prepare a novel PEG-PLA micelles loading DOX or coumarin-6 (TK-MS/DOX or TK-MS/C6). The obtained TK-MS/DOX exhibited uniform, spherical shape with a size of 23.80 ± 0.32 nm and zeta potential of 12.21 ± 0.31 mV. The release behavior of DOX from micelles were observed no significant changes after TK modification, however, the release profile exhibited pH-sensitive properties. Compared with MS/DOX, TK-MS/DOX exhibited significantly stronger cytotoxicity for Caco-2. Confocal laser microscopy and flow cytometry data further indicated that the targeting micelles not only had higher uptake by Caco-2 cells, but also more effectively penetrated the tumor spheroids. Therefore, TK peptide appears to be suitable as a targeting ligand with potential applications in colonic targeted therapy.
Introduction
According to a WHO report, the number of cancer patients may increase by 50% to 15 million new patients by 2020 (World Health Organization, Citation2013). Colonic cancer is approximately 40% of the cancers diagnosed yearly. It is the third most common cancer and the fourth leading cause of mortality among cancer patients (Jemal et al., Citation2010). Surgery with adjunctive chemotherapy and/or radiotherapy is considered the gold standard treatment for colon cancer (Wong et al., Citation2011). However, nearly 40% of surgical patients develop recurrence or metastases and chemotherapy often has adverse effects and off-target actions. Therefore, efficient and site-specific delivery of therapeutic drugs is a critical challenge in the clinical treatment of cancer. Nanocarriers including liposomes, micelles and polymeric nanoparticles have been applied for tumor therapeutics by various mechanisms such as the enhanced permeability and retention (EPR) effect (Hirsjarvi et al., Citation2011). Conjugation of targeting ligands onto nanocarriers could further improve nanocarriers selective delivery to the tumor cell or the tumor vasculature (Zhang et al., Citation2010a,Citationb; Arias, Citation2011; Xu et al., Citation2012). It has been indicated that nanocarrier targeted delivery onto receptor improved therapeutic responses in vitro and in vivo (Khan et al., Citation2011).
Integrins are a large family of cell adhesion receptors (Humphries, Citation2000; Hynes, Citation2002; Johnson et al., Citation2009; Khan et al., Citation2011). All integrins are non-covalently linked heterodimeric molecules containing one α and one β subunit (Nagae et al., Citation2012). Integrins receptors that mediate cell–cell and cell–ECM interactions and it has been shown that these receptors directly or indirectly influence cell migration, cell behavior, cell proliferation, cell growth, angiogenesis, cell survival, invasion and tumorigenesis (Reddy & Mangale, Citation2003). Integrins have extracellular ligand-binding sites; thus, they are a promising therapeutic targets. Integrins have been targeted by allosteric inhibitor and by ligand-mimetic antagonists of their ligand-binding sites (Cox et al., Citation2010; Millard et al., Citation2011). They have been applied to targeted drug delivery in several forms. Integrin α5β1 and αv are used as cancer targeting (Desgrosellier & Cheresh, Citation2010); αvβ3 targets angiogenesis in tumors (Desgrosellier & Cheresh, Citation2010; Robinson & Hodivala-Dilke, Citation2011) and is used to treat arthritis (Wilder, Citation2002) and osteoporosis (Nakamura et al., Citation2007); and β7 is in Phase II trial for the treatment of ulcerative colitis (Stefanich et al., Citation2011). Therefore, integrins receptors are forward-looking therapeutic targets.
Cell surface receptors are dissimilar for different cancers from different diseases, which resulted in that improving targeting of tumor cells with high specificity is a complex and arduous task. Ligands are an imperative part of the specific drug delivery system and choosing a ligand with high affinity is a subject of considerable interest. Integrin α6β1 is a subtype of integrins that is up-regulated in human colon cancer cells (Chao et al., Citation1996; Ni et al., Citation2001; Zhang et al., Citation2010a,Citationb). Nakahara et al. (Citation1996) reported that a 12-residue peptide (TWYKIAFQRNRK), derived from the COOH-terminal globular domain of laminin-1 alpha1 chain, interacts with integrin α6β1 with high affinity. However, the application of this 12-residue peptide, termed TK, has not been reported in the field for any targeted drug delivery system. Thus, we hypothesis that TK peptide could target colonic cancer.
In this study, to verify our hypothesis that TK peptide could target colonic cancer, TK peptide was synthesized to investigate its targeting properties. Then, TK peptide was integrated into PEG-PLA micelles to further study potential applications of TK peptide as a targeting ligand in colonic targeted therapy. To the best of our knowledge, no study has been reported regarding the application of TK peptide in targeted drug delivery.
Materials and methods
Materials
Integrin α6β1 was purchased from R&D and chip CM5 was purchased from GE. Coumarin-6 and Sephadex G50 were purchased from Sigma (St. Louis, MO). Fluorescein-5-maleimide was purchased from Fanbo Biochemicals (Beijing, China). PEG-PLA was purchased from Advanced Polymer Materials Inc. (Montreal, Canada). DOX was obtained from ArK. Caco-2 cells were obtained from the Shanghai Institute of Cell Biology and were cultured in Dulbecco’s modified Eagle’s medium (DMEM) supplemented with 10% fetal bovine serum (FBS; Gibco), 100 U/mL penicillin and 100 μg/mL streptomycin at 37 °C under a humidified atmosphere containing 5% CO2.
Synthesis of TK and FITC-TK peptides
TK peptide was synthesized by solid phase peptide synthesis using active ester chemistry to Boc-protected amino acid to the deprotected resin. The Boc protecting group was removed with 100% trifluoroacetic acid, and dimethylformamide was used as both the coupling and flow wash solvent throughout the cycle. To prepare fluorescein-labeled peptides, an excess of Mal-FITC was dissolved in of PBS (0.1 M, pH 7.0) with TK-SH and was stirred for 1 h in the dark. The mixture was purified by preparative HPLC (Waters 600 E, Milford, MA) on a Symmetry 300 Å C-18 reversed column (300 mm ×19 mm) using a gradient method. TK-FITC was collected and measured with a mass spectrometer (Agilent 1100 series).
Molecular binding affinity study
To inspect the binding affinities of TK peptide with integrin α6β1, surface plasmon resonance (SPR, Biacore 3000, Sweden) analysis was used and a 7-residue peptide (TISWPPR) was used as a control. Briefly, human integrin α6β1 molecules were immobilized on a CM5 sensor chip via the amide-coupling method. The chip surface was activated by the introduction of a NHS/EDC mixture and integrin (in 10 mM acetate, pH 4.0) was pipetted over the activated surface. Finally, unreacted activated groups were blocked by ethanolamine. All experiments were performed at 25 °C with a continuous flow rate of 10 μL/min using HBS-EP buffer (10 mM HEPES, 150 mM NaCl, 3 mM EDTA and 0.05% Tween 20, pH 7.4). Samples concentrations of 0–20 μM in HBS-EP buffer were tested to evaluate the ligand-protein binding affinity by determining their equilibrium dissociation rate constants (KD). Biacore 3000 Evaluation Software version 4.5 (GE Healthcare, Piscataway, NJ) was used for the fitting of the curve to the entire material concentration series data set. During the assays, the signal was corrected against the control surface response to eliminate bulk refractive index changes and non-specific binding.
In vitro cell study of TK peptide
Cellular uptake
Caco-2 cells were seeded in confocal dishes or 12-well plates at a density of 105 cells per well in 0.5 mL DMEM with 10% FBS and were incubated overnight at 37 °C. After the 24 h incubation, the culture medium was replaced with 5 μM TK-FITC in DMEM supplemented with 10% FBS, and the cells were incubated at 37 °C for 4 h. The fluorescent intensity was captured by a laser scanning confocal microscope or flow cytometry. For the study of intracellular distribution, cells were fixed by formaldehyde and imaging of the fluorescent intensity was captured.
Tumor spheroid uptake
To further evaluate the tumor-penetrating ability of TK-FITC, a tumor spheroid model was prepared and used as previously described (Gao et al., Citation2012). Briefly, 150 μL of 2% low-melting temperature agarose was added into 48-well plates. After the agarose was set, Caco-2 cells were seeded in the 48-well plates (5 × 103 cells/400 μL per well) to prepare tumor spheroids. After seven days, TK-FITC molecules, at a final concentration of 5 μM, were added to the plate, and the plates were incubated for 4 h. The spheroids were rinsed with ice-cold PBS and fixed with 4% formaldehyde for 30 min and then observed with a laser scanning confocal microscope.
Synthesis of TK-PEG-PLA
TK-PEG-PLA molecules were synthesized through covalent conjugation of thiolated peptide with mal-PEG-PLA (Zhan et al., Citation2010a,Citationb). In brief, 20 mg of mal-PEG-PLA in 5 ml acetonitrile was rotary evaporated to form a thin film that was then hydrated with 3 ml PBS (pH 8.0, 0.2 M). Over 8 h, 10 mg of TK-SH was added, and the reaction was stirred overnight. The reaction was monitored by HPLC (Agilent 1100 series). The excessive TK-SH was removed by dialysis (MWCO 3.5 kDa) against distilled water and confirmed by the disappearance of the peak of TK-SH in HPLC spectrum. The mPEG-PLA and TK-PEG-PLA polymers were characterized by 1H NMR analysis.
Preparation of mPEG-PLA micelles and TK-PEG-PLA micelles
For the preparation of micelles (MS), mPEG-PLA or a mixture of mPEG-PLA/TK-PEG-PLA (95/5, by mol), was dissolved in chloroform. It was then rotary evaporated to form a thin film at 37 °C and, subsequently, hydrated with physiological saline (Zhan et al., Citation2010a,Citationb). For DOX encapsulated micelles, DOX·HCl was dissolved in purified water and NaHCO3 was added into this solution for to remove hydrochloride from DOX·HCl. Then chloroform was used to extract free DOX from the reaction mixture and CHCl3 was removed by rotary evaporation. DOX was dissolved in CHCl3 together with mPEG2000-PLA or a mixture of mPEG2000-PLA/TK-PEG-PLA to form the thin film, which was hydrated with saline and passed over a gel filtration column. For the loading of coumarin-6, fluorophores were dissolved in acetonitrile that was added into CHCl3 with mPEG2000-PLA or a mixture of mPEG2000-PLA/TK-PEG-PLA to form the thin film. Then the free dyes were removed by gel filtration column eluting with physiological saline.
Characterization of micelles
Drug-loading capacity and encapsulation efficiency
PEG-PLA Micelles were diluted in acidic isopropanol solution to release DOX. After precipitating the polymers with acidic isopropanol solution, samples were filtrated through a 0.45 µm nylon filter. The concentration of DOX in the filtrate was determined via a fluorescence spectrophotometer and detection wavelength was 480 nm. Drug-loading capacity (DLC) and encapsulation efficiency (EE) were calculated as indicated below, and the values were reported as mean ± SD (n = 3).
(1)
(2)
Particle size, Zeta potential and surface morphology
The hydrodynamic particle size and zeta potential of the micelles in water were measured using a Malvern Zetasizer Nano ZS (Malvern, Worcestershire, UK). All of the dynamic light scattering (DLS) measurements were performed at 25 °C. The surface morphology of the micelles was observed by transmission electron microscopy (TEM; JEM-1400, JEOL, Tokyo, Japan) after negative staining with 4% phosphotungstic acid and dried on carbon-coated grids for examination.
In vitro release of DOX from micelles
The in vitro release behavior of DOX from micelles was evaluated by a dialysis method. Briefly, 0.5 mL of purified micelles solution were put inside a dialysis bag (MWCO = 12 000–14 000 Da) and tightly sealed at both ends. The whole bag was immersed in 30 mL PBS (0.1 M, pH 7.4 and pH 5.8) and was placed in a shaking water bath at 37 °C. At pre-determined time points (0.25, 0.5, 1, 4, 6, 8, 10, 24 and 48 h), 0.5 mL sample were withdrawn and replaced with an equal volume of fresh release medium. The concentration of DOX in each time point was determined by using fluorescence spectrophotometer at 480 nm and the cumulative release was calculated and plotted against time. All data are the average points of determination (3 times).
In vitro cell study of micelles
Cellular uptake
Caco-2 cells were seeded in confocal dishes or 12-well plates at a density of 105 cells per well in 0.5 mL DMEM with 10% FBS and were incubated overnight at 37 °C. After the 24 h incubation, the culture medium was replaced with 20 ng/mL coumarin-6 loaded in micelles in DMEM supplemented with 10% FBS, and the cells were incubated at 37 °C for 1 h. The fluorescent intensity was captured by a laser scanning confocal microscope or flow cytometry. For the study of intracellular distribution, cells were fixed by formaldehyde and imaging of the fluorescent intensity was captured.
Tumor spheroid penetration
Caco-2 tumor spheroids were prepared to mimic the tumor parenchyma and observe penetration efficiency of targeting micelles. Micelles loading coumarin-6 at a final concentration of 20 ng/mL were added to the plate and incubated for 4 h. The spheroids were rinsed with ice-cold PBS and fixed with 4% formaldehyde for 30 min and then observed with a laser scanning confocal microscope.
Cytotoxicity assay
In vitro antiproliferative activities of DOX and DOX-loaded micelles against Caco-2 cells were estimated by MTT assay. The cells were seeded at a density of 5 × 103 cells per well in 96-well plates for 24 h. After 24 h incubation, cell culture medium was replaced with fresh DMEM medium containing various DOX concentrations of the different formulations. After additional 4 h incubation, the medium was removed and the cells were continuously incubated with fresh DMEM medium containing 10% FBS for 72 h. After treatment, the medium was replaced with 0.5 mg/mL MTT in DMEM and the cells were incubated for another 4 h. Then, the supernate was removed and 100 µL dimethyl sulfoxide was added to each well to dissolve the formazan crystals. Cell viability was quantitated by determining optical absorption at 492 nm and the negative control was as 100%. The viability percentage was calculated as the percentage of absorbance of the study group over the control group.
Statistical analysis
The data are expressed as the mean ± standard deviation (SD). The significance of the difference from the controls for each experimental test condition was assayed using Student’s t-test for each paired experiment. A p value of <0.05 was regarded as a significant difference.
Results
Characterization of TK and TK-FITC
TK was synthesized by a Fmoc-protected solid phase peptide synthesis method. The crude TK and TK-FITC peptides were analyzed and purified by HPLC. As shown in , the purity and molecular weight of the TK and TK-FITC peptides were determined by HPLC and ESI-MS spectrometry, respectively. The results of HPLC showed that the purity of TK and TK-FITC was more than 95%. The ESI-MS data shown in indicated that the TK and TK-FITC molecular ion peak was 1610.9 and 2113.56, respectively, which was consistent with their theoretical molecular weights.
Molecular binding affinity
The interactions of the TK peptide and control peptide were investigated with the immobilized integrin α6β1 on the CM5 sensor chip at a level of 23 000 response units (RU) by the standard amine coupling method. The chip was used to measure the binding affinity of TK peptide and control peptide at concentrations ranging from 0.625 to 20 μM. RU values evaluating TK peptide binding to the integrin α6β1 indicated an obvious concentration-dependent pattern (). The equilibrium dissociation constant (KD) of TK peptide to the integrin α6β1 was 2.79 × 10−5, which was greatly lower than the KD value (1.51 × 10−2) of the control peptide. Meanwhile, the chi-squared value of TK peptide was calculated to be 7.01 by Biacore 3000 Evaluation Software version 4.5, which demonstrated a good fit. This was in accordance with the finding of Baglia et al. (Citation2000) who chi-square values less than 10 indicate a good fit. These findings indicated that TK had a high binding affinity to integrin α6β1.
In vitro cell study of TK peptide
To further illustrate the binding affinity of TK peptide to integrin α6β1, Caco-2 cells were used to observe the cell uptake of TK-FITC. TK peptide was labeled with fluorescein by an interaction between the thiol group of the peptide and the maleimide group of fluoresein (fluorescein-5-maleimide). Caco-2 cells were incubated with 5 μM TK-FITC for 4 h at 37 °C and then imaged by confocal laser microscopy and analyzed by flow cytometry. As shown in , Caco-2 cells could uptake TK-FITC, and TK-FITC uptake by Caco-2 cells was significantly higher than that for free FITC. The results of flow cytometry shown in indicated that 66.59% of the cells containing TK-FITC, compared with only 4.43% of cells containing free FITC. These results show that TK-FITC could specifically recognize Caco-2 cells and has a high affinity for Caco-2 cells.
Figure 3. In vitro cellular uptake results of FITC and TK-FITC in Caco-2. Cells were incubated with 5 μM fluorescein-labeled peptide or FITC at 37 °C for 4 h, followed by DAPI staining and rinse with phosphate buffered saline. Intracellular fluorescence was detected by confocal laser scanning microscope (A) and flow cytometer (B).
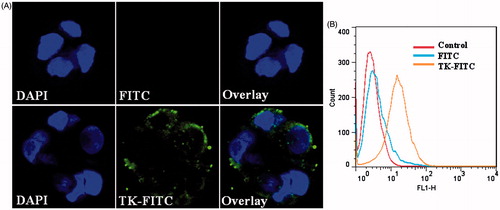
To imitate the in vivo status of solid tumors, tumor spheroids were applied to investigate the penetrating ability of TK via confocal microscopy. Tumor pheroid were incubated with 5 μM fluorescein-labeled peptide or FITC at 37 °C for 4 h, followed by being examined by confocal microscopy with a 5 μm interval between consecutive slides. As shown in , there was minimal fluorescence for the free FITC distributed in the tumor spheroids; in contrast, TK-treated tumor spheroids showed strong fluorescence. These results indicate that the TK-FITC peptide could not only increases uptake by Caco-2 cells, but also effectively increases uptake by the tumor spheroids.
Synthesis and characterization of TK-PEG-PLA
The NMR spectra of Mal-PEG-PLA and TK-PEG-PLA are shown in . The 1HNMR spectrum of Mal-PEG-PLA shows characteristic peaks for PEG at 3.6–3.8 ppm (CH2), for PLA at 1.6 ppm (CH3) and for MAL at 6.7 ppm, respectively. In contrast, the characteristic peaks of PEG and PLA remained in the NMR spectrum of TK-PEG-PLA, but the maleimide peak completely disappeared. This suggests that the TK peptide had been successfully conjugated to Mal-PEG-PLA.
Characterization of micelles
PEG-PLA micelles had a good capacity for DOX, with DLC of 10.15 ± 1.32% and 10.83 ± 1.67%, EE of 75.85 ± 2.27% and 77.96 ± 5.27% for MS/DOX or TK-MS/DOX, respectively, which indicated that conjugation of TK did not affect loading of DOX into the micelles.
DOX could be better accumulated in tumor tissues by EPR effect, if the size of micelles is small enough. lists the size of MS/DOX or TK-MS/DOX. The average size of MS/DOX or TK-MS/DOX was 21.70 ± 0.54 and 23.80 ± 0.32 nm, respectively (p < 0.05), and the size of TK-MS/DOX increased about 2 nm after TK conjugated. Although with an increase of 2 nm, the size of TK-MS/DOX was still approach 20 nm, which was better for delivery drugs to the tumor tissue (Perrault et al., Citation2009). Polydispersity index (PDI) of each was 0.036 ± 0.013 and 0.201 ± 0.018, respectively (p < 0.01). The conjugation of TK led to a slight increase of the size, probably because TK with MW of 1610.91 was a relatively macromolecule for 20 nm micelles after conjugation to its surface, which could cause a minor extension of the micelles ball (Wei et al., Citation2014). The zeta potential of MS/DOX or TK-MS/DOX was 14.85 ± 1.03 and 12.21 ± 0.31 mV (p < 0.01), respectively, which indicated that conjugation of TK partially neutralized the positive charge on the surface of the micelles.
Figure 6. Characterization of micelles. (A) Particle size and distribution of mPEG-PLA and TK-PEG-PLA micelles loading DOX by the DLS analysis. (B) Morphology of mPEG-PLA and TK-PEG-PLA micelles loading DOX by the TEM analysis negatively staining with 4% phosphotungstic acid solution. Bar is 20 nm. (C) In vitro DOX release profiles from blank micelles and TK modified micelles at neutral condition (pH 7.4) and acidic conditions (pH 5.8) at 37 °C. Each points represent the mean (n = 3).
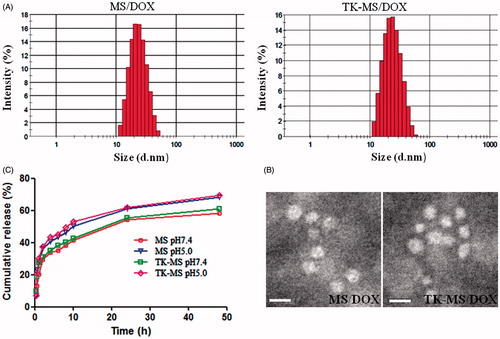
Transmission electron microscopy is a powerful tool to intuitively visualize the size and surface morphology of micelles. As shown in , TEM images exhibited that these micelles were nearly spherical shapes with uniform size. The size observed by TEM was smaller than that determined with DLS, which probably was due to the shrink of the micelles during the drying process of TEM sample preparation (Wei et al., Citation2014).
In vitro drug release
indicates the DOX release profile of MS/DOX and TK-MS/DOX in two different buffer solutions (pH 7.4 and 5.8). A typical two-phase-release profile was observed for MS/DOX and TK-MS/DOX in two different buffer solutions. It is a relatively rapid release in the first stage and it shows a sustained and slow release in the second stage. The rapid release stage was beneficial to suppress the growth of tumor cells in a short time, while the sustained release stage would provide the possibility to continually fight against tumor cells (Gong et al., Citation2010). As shown in , the in vitro release behaviors of MS/DOX and TK-MS/DOX was similar in pH 7.4 or 5.8 buffer solutions, which explained that conjugation of TK did not affect release of DOX in the micelles. However, in comparison with the release at pH 7.4, DOX release from micelles at pH 5.8 is much faster. This results was in agreement with Kataoka’s reporter who also observed the faster release of DOX in acidic conditions (Kataoka et al., Citation2000), and is due likely to the deprotonation of the amino group of DOX at lower pH. This pH-dependent releasing behavior is helpful to deliver more DOX to tumor tissue. Most DOX encapsulated in micelles will remain in the micelle cores for a considerable time period when the injected micelles stay in the plasma at normal physiological conditions (pH 7.4). However, a faster release will occur once the micelles reach the solid tumor site where local pH is reported to be lower than that of normal tissue (Gerweck & Seetharaman, Citation1996). Moreover, micelles are usually internalized into the cells by endocytosis (Nam et al., Citation2009), which resulted in a further accelerated release inside the endosome/lysosome of tumor cells due to low pH values.
In vitro cell study of micelles
To investigate whether TK peptide could increase the cellular uptake of PEG-PLA micelles, coumarin-6 was used as a fluorescence probe for mPEG-PLA and TK-PEG-PLA micelles, and cellular uptake was performed by incubating the micelles with Caco-2 cells at 37 °C for an hour. The laser scanning confocal microscope images () of Caco-2 cellular uptake showed that the accumulation of TK-PEG-PLA micelles was slightly higher than that of the mPEG-PLA micelles. In , the flow cytometry data indicated that the percentage of fluorescence-positive cells and the mean fluorescent intensity were 99.97% and 345.72 for PEG-PLA micelles, and 100.00% and 386.54 for TK-PEG-PLA micelles, respectively. The results of flow cytometry and laser scanning confocal microscopy suggest that affinity of targeting micelles to Caco-2 was similar to that of the targeting peptide.
Figure 7. In vitro cellular uptake results of MS and TK-MS in Caco-2. Cells were incubated with 20 ng coumarin-6 loaded in MS or TK-MS at 37 °C for 1 h, followed by DAPI staining and rinse with phosphate buffered saline. Intracellular fluorescence was detected by confocal laser scanning microscope (A) and flow cytometer (B).
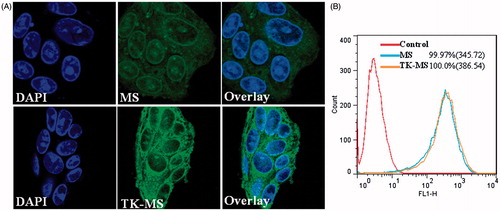
As targeting drug delivery system could delivery drug carriers deeper into solid cancer regions. Thus, tumor spheroid penetration experiments were carried out in addition to the cellular uptake tests. The confocal microscopy data indicated the penetration efficacy of the targeting micelles and non-targeting micelles. As shown in , TK-PEG-PLA micelles penetrated deeper and were distributed more extensively in the spheroids than mPEG-PLA micelles, which illustrates that TK modification could effectively enhance the tumor penetration of micelles.
Cytotoxicity assay
To evaluate the selectivity of TK-MS/DOX, the cytotoxicity of MS/DOX and TK-MS/DOX for Caco-2 cells were compared. The IC50 values of free DOX, MS/DOX and TK-MS/DOX for Caco-2 cells was 1.6 ± 0.31, 2.1 ± 0.64 and 1.6 ± 0.48 µg/ml, respectively.
After 72 h of incubation, IC50 values of free DOX for Caco-2 cells were the lowest compared with that of micelles. This might be because free DOX can be readily transported into cells and diffuse into cytosol by passive diffusion, however, the common micelles have to be internalized by endocytosis and then progressively release the loaded drugs, which usually delay the cytosolic and nuclear drug delivery and result in less efficacy in cancer cell inhibition (Sun et al., Citation2010). However, the IC50 values significantly decreased when the micelles were modified with TK peptides. These cytotoxicity results suggested that micelles modified TK could increase the selectivity for tumor cells.
Discussion
Colonic cancer is approximately 40% of the cancers diagnosed yearly. It is the third most common cancer and the fourth leading cause of mortality among cancer patients. Nanodrug delivery systems have been widely recognized as an emerging and promising field with the potential to offer solutions to the current obstacles in cancer therapies (Farokhzad & Langer, Citation2009; Zhao et al., Citation2013; Chen et al., Citation2014). Moreover, targeting nanocarriers could further improve nanocarriers selective delivery to the tumor tissue. Hence, the efficient and site-specific delivery of therapeutic drugs is a critical challenge in the clinical treatment of cancer. Ligands are an imperative part of the specific drug delivery system and choosing a ligand with high affinity is a subject of considerable interest. In our study, we synthesized 12-residue peptide (TK) with high affinity with integrin α6β1 that is over expressed in human colon cancer cells and exploited the feasibility of target of TK peptide for Caco-2 cells.
To investigate target of TK peptide for Caco-2 cells, we evaluate TK peptide in molecular and cells level. Surface plasmon resonance (SPR) is an optical method that is generally applied to investigate enzymatic reactions (Iwasaki et al., Citation2001), RNA-hybridization (Iguchi et al., Citation2013), antibody–antigen interactions (Karlsson & Fält, Citation1997; Weeraratne et al., Citation2013), etc. Therefore, SPR technology was used to study the binding affinity between TK peptide and integrin α6β1. The results indicated that TK had a high binding affinity to integrin α6β1.
To further illustrate the binding affinity of TK peptide to integrin α6β1, Caco-2 cells were used to observe the cell uptake of TK-FITC. Confocal laser microscopy and flow cytometry data indicated that TK-FITC could specifically recognize Caco-2 cells and has a high affinity for Caco-2 cells (). However, in vivo and in vitro environments are significantly different (Ziolkowska et al., Citation2011); thus, it is difficult to extrapolate data from in vitro experiments to possible effects in vivo (Yamada & Cukierman, Citation2007). Consequently, a mimicking in vivo circumstance technique is necessary to investigate the targeting of this ligand. Tumor spheroids have physiology and morphology similar to tumors in vivo (Sutherland, Citation1988) and act as a better cellular model of anticancer therapy testing than other in vitro models (Lin & Chang, Citation2008). Therefore, tumor spheroids were applied to imitate the in vivo status of solid tumors and investigate the penetrating ability of TK via confocal microscopy. TK-treated tumor spheroids showed stronger fluorescence than that of FITC (). These results indicate that the TK-FITC peptide could not only increases uptake by Caco-2 cells, but also effectively increases uptake by the tumor spheroids.
To investigate the feasibility of TK peptide as ligand of nanocarrier, TK was conjugated to PEG-LPA to prepare TK-PEG-LPA micelles. Coumarin-6 was used as a fluorescence probe to observe the cell uptake TK-PEG-LPA micelles. Confocal laser microscopy and flow cytometry data indicated that TK-PEG-LPA micelles has a higher affinity for Caco-2 cells than that of mPEG-LPA micelles (), which was similar to results of the targeting peptide. In general, nanocarriers are preliminarily evaluated using a two-dimensional monolayer cell (2D) model. However, 2D cell cultures lack a three-dimensional (3D) architecture and extracellular matrix, which are important characteristics of tumors in vivo (Goodman et al., Citation2007, Citation2008). Thus, the results using 2D cell models may not be similar to those observed in vivo. A 3D in vitro model is more accurate method for the optimization of nanodrug delivery systems in vitro (Minchinton & Tannock, Citation2006; Kim et al., Citation2010). As shown in , tumor spheroid penetration experiments illustrates that TK modification could effectively enhance the tumor penetration of micelles.
DOX is a widely chemotherapeutic agent used in the treatment of malignant tumors, but DOX has demonstrated severe side effects, such as dose-related acute cardiotoxicity, bone marrow suppression and multidrug resistance in the clinical use (Steinherz et al., Citation1991; Ray et al., Citation2000; Carvalho et al., Citation2009). Many drug delivery systems such as micelles have been developed to diminish the toxicity and increase the antitumor efficacy of DOX. Consequently, DOX was used as a model drug to be loaded into TK-PEG-LPA micelles. The results of characterization of micelles indicated that the conjugation of TK into the surface of micelles had no significant effect on the incorporation of DOX, only leading to a slight increase of size, which suggested that superficial modification of TK was feasible according to micelles structure. It is reported that the permeation of nanocarrier in the tumor tissue mainly depends on its size, that is, larger nanoparticles (∼100 nm) were mostly localized in the perivascular region, while smaller nanoparticles (∼20 nm) rapidly distributed throughout the tumor tissue (Perrault et al., Citation2009). Hence, the TK-MS/DOX with average size of 23.80 ± 0.32 nm is more suitable for targeting to tumor tissue. Compared with MS/DOX, TK-MS/DOX exhibited significantly stronger cytotoxicity for Caco-2, which further display that micelles modified TK could increase the selectivity for tumor cells.
Conclusions
In our study, a 12-residue peptide (TK) was first synthesized, which had a high binding affinity for integrin α6β1 overexpressed on colonic cancer cells. TK peptide not only increases uptake by Caco-2 cells, but also effectively increases the penetration of the tumor spheroids. TK-PEG-PLA was further synthesized to prepare targeting micelles. DOX was successfully loaded into TK-PEG-PLA micelles and the obtained drug-loaded micelles exhibited spherical shape and small particle sizes. TK conjugation showed no adverse effect on drug-loading capacities and in vitro release behavior of PEG-PLA micelles for DOX, only leading to a minor increase of size and a slight decrease of zeta potential. The release profile exhibited pH-sensitive properties. Compared with MS/DOX, TK-MS/DOX exhibited significantly stronger cytotoxicity to Caco-2 cells. The results of flow cytometry and laser scanning confocal microscope further confirmed that the modification of TK peptide enabled the penetration of micelles through cancer cells and into deep tumor tissues. Therefore, TK peptide is a promising targeting ligand and TK peptide modification is an effective strategy for improving the transport of micelles into colonic cancer tissue.
Declaration of interest
The authors report no conflicts of interest. This work was supported by the Wu Liande Fund for the Youth of Harbin Medical University (WLD-QN1111), Natural Science Foundation Project for the Youth of Heilongjiang province (QC2013C029) and Post-Doctoral Scientific Research Startup Fund (LBH-Q13131).
References
- Arias JL. (2011). Drug targeting strategies in cancer treatment: an overview. Mini Rev Med Chem 11:1–17
- Baglia FA, Badellino KO, Ho DH, et al. (2000). A binding site for the kringle II domain of prothrombin in the apple 1 domain of factor XI. J Biol Chem 41:31954–62
- Carvalho C, Santos RX, Cardoso S, et al. (2009). Doxorubicin: the good, the bad and the ugly effect. Curr Med Chem 16:3267–85
- Chao C, Lotz MM, Clarke AC, Mercurio AM. (1996). A function for the integrin alpha6beta4 in the invasive properties of colorectal carcinoma cells. Cancer Res 56:4811–19
- Chen D, Lian S, Sun J, et al. (2014). Design of novel multifunctional targeting nano-carrier drug delivery system based on CD44 receptor and tumor microenvironment pH condition. Drug Deliv 3:1–6
- Cox D, Brennan M, Moran N. (2010). Integrins as therapeutic targets: lessons and opportunities. Nat Rev Drug Discov 9:804–20
- Desgrosellier JS, Cheresh DA. (2010). Integrins in cancer: biological implications and therapeutic opportunities. Nat Rev Cancer 10:9–22
- Farokhzad OC, Langer R. (2009). Impact of nanotechnology on drug delivery. ACS Nano 3:16−20
- Gao H, Qian J, Yang Z, et al. (2012). Whole-cell SELE X aptamer-functionalised poly-(ethyleneglycol)-poly(ɛ-caprolactone) nanoparticles for enhanced targeted glioblastoma therapy. Biomaterials 33:6264–72
- Gerweck LE, Seetharaman K. (1996). Cellular pH gradient in tumor versus normal tissue: potential exploitation for the treatment of cancer. Cancer Res 56:1194–8
- Gong C, Wei X, Wang X, et al. (2010). Biodegradable self-assembled PEG-PCL-PEG micelles for hydrophobic Honokiol delivery: I. Preparation and characterization. Nanotechnology 21:215103
- Goodman TT, Olive PL, Pun SH. (2007). Increased nanoparticle penetration in collagenase-treated multicellullar spheroids. Int J Nanomed 2:265–74
- Goodman TT, Ng CP, Pun SH. (2008). 3-D tissue culture systems for the evaluation and optimization of nanoparticle-based drug carriers. Bioconjug Chem 19:1951–9
- Hirsjarvi S, Passirani C, Benoit JP. (2011). Passive and active tumour targeting with nanocarriers. Curr Drug Discov Technol 8:188–96
- Humphries MJ. (2000). Integrin structure. Biochem Soc Trans 28:311–39
- Hynes RO. (2002). Integrins: bidirectional, allosteric signaling machines. Cell 110:673–87
- Iguchi A, Fukuda N, Takahashi T, et al. (2013). RNA binding properties of novel gene silencingpyrrole-imidazole polyamides. Biol Pharm Bull 36:1152–8
- Iwasaki Y, Horiuchi T, Niwa O. (2001). Detection of electrochemical enzymatic reactions by surface plasmon resonance measurement. Anal Chem 73:1595–8
- Jemal A, Siegel R, Xu J, Ward E. (2010). Cancer statistics. CA Cancer J Clin 60:277–300
- Johnson MS, Lu N, Denessiouk K, et al. (2009). Integrins during evolution: evolutionary trees and model organisms. Biochim Biophys Acta 1788:779–89
- Karlsson R, Fält A. (1997). Experimental design for kinetic analysis of protein–protein interactions with surface plasmon resonance biosensors. J Immunol Methods 200:121–33
- Kataoka K, Matsumoto T, Yokoyama M, et al. (2000). Doxorubicin-loaded poly(ethylene glycol)-poly(beta-benzyl-l-aspartate) copolymer micelles: their pharmaceutical characteristics and biological significance. J Control Release 64:143–53
- Khan JA, Kudgus RA, Szabolcs A, et al. (2011). Designing nanoconjugates to effectively target pancreatic cancer cells in vitro and in vivo. PLoS One 6:e20347
- Kim B, Han G, Toley BJ, et al. (2010). Tuning payload delivery in tumour cylindroids using gold nanoparticles. Nat Nanotechnol 5:465–72
- Lin R, Chang H. (2008). Recent advances in three-dimensional multicellular spheroid culture for biomedical research. J Biotechnol 3:1172–84
- Millard M, Odde S, Neamati N. (2011). Integrin targeted therapeutics. Theranostics 1:154–88
- Minchinton AI, Tannock IF. (2006). Drug penetration in solid tumours. Nat Rev Cancer 6:583–92
- Nagae M, Re S, Mihara E, et al. (2012). Crystal structure of α5β1 integrin ectodomain: atomic details of the fibronectin receptor. J Cell Biol 197:131–40
- Nakahara H, Nomizu M, Akiyama SK, et al. (1996). A mechanism for regulation of melanoma invasion. Ligation of alpha6beta1 integrin by laminin G peptides. J Biol Chem 271:27221–4
- Nakamura I, Duong T, Rodan SB, Rodan GA. (2007). Involvement of alpha(v)beta3 integrins in osteoclast function. J Bone Miner Metab 25:337–44
- Nam HY, Kwon SM, Chung H, et al. (2009). Cellular uptake mechanism and intracellular fate of hydrophobically modified glycol chitosan nanoparticles. J Control Release 135:259–67
- Ni H, Gagne D, Basora N, Beaulieu JF. (2001). Up-regulation of the α6β4 integrin in human colon cancer cells. Gastroenterology 120:A61
- Perrault SD, Walkey C, Jennings T, et al. (2009). Mediating tumor targeting efficiency of nanoparticles through design. Nano Lett 9:1909–15
- Ray MR, Lakshmi C, Deb C, et al. (2000). Modulatory effect of dopamine on doxorubicin-induced myelosuppression. Comp Haematol Int 10:212–20
- Reddy KV, Mangale SS. (2003). Integrin receptors: the dynamic modulators of endometrial function. Tissue Cell 35:260–73
- Robinson SD, Hodivala-Dilke KM. (2011). The role of beta3-integrins in tumor angiogenesis: context is everything. Curr Opin Cell Biol 23:630–7
- Steinherz LJ, Steinherz PG, Tan CT, et al. (1991). Cardiac toxicity 4 to 20 years after completing anthracycline therapy. J Am Med Assoc 266:1672–7
- Stefanich EG, Danilenko DM, Wang H, et al. (2011). A humanized monoclonal antibody targeting the beta7 integrin selectively blocks intestinal homing of T lymphocytes. Br J Pharmacol 162:1855–70
- Sun Y, Yan X, Yuan T, et al. (2010). Disassemblable micelles based on reduction-degradable amphiphilic graft copolymers for intracellular delivery of doxorubicin. Biomaterials 31:7124–31
- Sutherland R. (1988). Cell and environment interactions in tumor microregions: the multicell spheroid model. Science 240:177–84
- Weeraratne DK, Lofgren J, Dinnogen S, et al. (2013). Development of a biosensor-based immunogenicity assay capable of blocking soluble drug target interference. J Immunol Methods 396:44–55
- Wei Y, Xu S, Wang F, et al. (2014). A novel combined micellar system of lapatinib and paclitaxel with enhanced antineoplastic effect against human epidermal growth factor receptor-2 positive breast tumor in vitro. J Pharm Sci 104:165–77
- Wilder RL. (2002). Integrin alphaV beta3 as a target for treatment of rheumatoid arthritis and related rheumatic diseases. Ann Rheum Dis 61:ii96–9
- World Health Organization. (2013). Global cancer rates could increase by 50% to 15 million by 2020. Available from: http://www.who.int/mediacentre/news/releases/2003/pr27/en/ [last accessed 9 Aug 2015]
- Wong TW, Colombo G, Sonvico F. (2011). Pectin matrix as oral drug delivery vehicle for colon cancer treatment. AAPS Pharm Sci Tech 12:201–14
- Xu W, Cui Y, Ling P, Li LB. (2012). Preparation and evaluation of folate-modified cationic pluronic micelles for poorly soluble anticancer drug. Drug Deliv 19:208–19
- Yamada K, Cukierman E. (2007). Modeling tissue morphogenesis and cancer in 3D. Cell 130:601–10
- Zhan C, Gu B, Xie C, et al. (2010a). Cyclic RGD conjugated poly (ethylene glycol)-co-poly (lactic acid) micelle enhances paclitaxel anti-glioblastoma effect. J Control Release 143:136–42
- Zhan C, Yan Z, Xie C, Lu W. (2010b). Loop 2 of Ophiophagus hannah toxin b binds with neuronal nicotinic acetylcholine receptors and enhances intracranial drug delivery. Mol Pharm 7:1940–7
- Zhang J, Gao J, Tan X, et al. (2010a). Effects of down-regulation of integrin-β1 expression on migration and hepatic metastasis of human colon carcinoma. J Huazhong Univ Sci Technol 30:464–9
- Zhang HZ, Li XM, Gao FP, et al. (2010b). Preparation of folate-modified pullulan acetate nanoparticles for tumor-targeted drug delivery. Drug Deliv 17:48–57
- Zhao M, Li A, Chang J, et al. (2013). Develop a novel superparamagnetic nano-carrier for drug delivery to brain glioma. Drug Deliv 20:95–101
- Ziolkowska K, Kwapiszewski R, Brzozka Z. (2011). Microfluidic devices as tools mimicking in vivo environment. New J Chem 35:979–90