Abstract
The Asn-Gly-Arg (NGR) motif has previously been demonstrated to specifically bind to CD13, which is selectively overexpressed in tumor vasculature and some tumor cells (e.g. HT1080). It was reported that NGR-modified stealth liposomes (NGR-SL) could be prepared with different lipid composition, such as 1,2-dipalmitoyl-sn-glycero-phosphatidylcholine (DPPC), hydrogenated soy posphatidylcholine (HSPC) and soy posphatidylcholine (SPC). In the present study, NGR-modified liposomes were prepared with DPPC, HSPC, SPC or the mixture of HSPC and SPC. The resultant liposomes with different lipid composition were compared in terms of cell uptake, antitumor efficacy and targeted drug delivery efficiency using HT1080 tumor model. It was found that NGR-SL composed of the mixture of HSPC and SPC was able to improve targeted drug delivery efficiency to tumor producing the most significant antitumor activity. Collectively, the NGR-modified liposomes composed of the mixture of HSPC and SPC are promising carriers for the treatment of tumor. Besides NGR ligand, lipid composition could also significantly affect the targeted delivery efficiency to the tumor.
Introduction
There is currently widespread interest in the development of active targeting strategies, which involve surface conjugation of recognition moieties that impart an affinity for cellular receptors within tumor tissue, for the treatment of tumor. In general, specific interactions between ligands and target molecules induce the receptor-mediated endocytosis of vesicles containing antitumor drugs. The application of various tumor-associated antigens for targeting tumor treatment is well documented. Among them, antigens expressed on angiogenic tumor vasculature are particularly attractive as tumor-associated targets.
Angiogenesis has an important role in tumor growth and metastasis. Therefore, anti-angiogenic therapy by targeting tumor endothelial cells has been an attractive and important strategy in tumor therapy. Antigens expressed on angiogenic tumor vasculature have intimate contact with the blood circulation. Thus, they are geographically accessible immediately following intravenous injection delivery of a targeted agent.
Endothelial cells in angiogenic vessels express several proteins that are absent or barely detectable in established vessels, such as aminopeptidase N (APN) also referred to as CD13 (Fukasawa et al., Citation2006). CD13 is a membrane-bound, zinc-dependent metalloproteinase that plays a key role in tumor invasion and angiogenesis. NGR (Asn-Gly-Arg) peptide motif is a tumor-homing peptide, which was identified by in vivo screening of peptidephage display libraries. The principal receptor for NGR peptide motif is CD13 isoform expressed on the endothelial cells of tumor blood vessels but not on the normal vasculature. Because of its good selectivity and low immunogenicity, NGR peptide has been identified as a potent targeting ligand for the targeted drug-containing liposomes to the tumor vascular antigen CD13 (Dunne et al., Citation2011; Luo et al., Citation2013; Xie et al., Citation2015). Moreover, the cyclic NGR peptide shows much higher stability and stronger binding activity than the liner NGR peptide (Negussie et al., Citation2010). Despite the higher affinity of cyclic NGR peptides, there has been a preference to use linear NGR-containing motifs to target liposomes to avoid the formation of disulfide bridges between adjacent peptides on the liposome surface that may render the ligand ineffective (Corti & Ponzoni, Citation2004).
It is well known that the phosphatidylcholine (PC) used to prepare targeted liposomes has significant effect on the properties of resulting liposomes. In the early 1980s, it had been demonstrated that liposomes obtained from PC with a high Tm were more stable in the blood than liposomes prepared with a low Tm (Senior, Citation1982). PC is an important consideration with liposome stability, and the state of liposomes at physiological temperature can be altered by inclusion of PC with different Tms. Gel-like structures of PC with Tm values higher than body temperature are more stable than those with short or unsaturated hydrocarbon chains and therefore low Tms (Chen et al., Citation2012). In addition, for stealth liposomes, it had been demonstrated that the choice of PC directed to a considerable extent the rate of cisplatin elimination from plasma and therapeutic effects (Alavizadeh et al., Citation2014). However, to the best of our knowledge, the effect of PC composition on the targeted delivery efficiency of NGR-modified liposomes is still unclear.
Consequently, PCs with different Tms were used to prepare NGR-modified liposomes, such as 1,2-dipalmitoyl-sn-glycero-3-phosphatidylcholine (DPPC) (Negussie et al., Citation2010; Dunne et al., Citation2011), hydrogenated soy posphatidylcholine (HSPC) (Pastorino et al., Citation2003; Garde et al., Citation2007; Takara et al., Citation2012) or soy posphatidylcholine (SPC) (Luo et al., Citation2013). The Tms of HSPC and DPPC are determined to be at 53 and 41 °C, respectively. However, the Tm of SPC is far below 0 °C.
In the present study, CD13 was employed as the target molecule, since it is a known tumor endothelial marker. The NGR-modified liposomes, in which the NGR motif is attached to the PEGylated liposomes, were prepared with different PC composition (HSPC, DPPC, SPC or the mixture of HSPC and SPC). In our previous study, the mixture of HSPC and SPC was applied for the preparation of liposomes containing antitumor drugs to obtain higher encapsulation efficiency (EE) (Chen et al., Citation2006, Citation2010).
Materials and methods
Materials
The PCs, soybean phosphatidylcholine (SPC), hydrogenated soybean phosphatidylcholine (HSPC) and 1,2-dipalmitoyl-sn-glycero-3-phosphatidylcholine (DPPC), 1,2-distearoyl-sn-glycero-3-phosphoethanolamine-N-(carbonyl-methoxypolyethyleneglycol-2000) (PEG-DSPE) were purchased from Lipoid Corp (Ludwigshafen, Germany). Cholesterol (CH) was purchased from Huixing Biochemistry Reagent Company (Shanghai, China). The NGR peptide, Lys-Asn-Gly-Arg-Gly (MW 571.59), was obtained from Qiangyao Biotechnology (Shanghai, China). NGR-PEG-DSPE was synthesized in our laboratory according to a previously reported method (Negussie et al., Citation2010). Near infrared lipophilic carbocyanine dye 1,1′-diocatdecyltetramethyl indotricarbocyanine iodide (DiR) was purchased from AAT Bioquest Inc. (Sunnyvale, CA). Coumarin-6 and brucine were supplied by Sigma-Aldrich Inc., (St Louis, MO). Reference substances of brucine and the internal standard (IS) huperzine A were supplied by National Institute for the Control of Pharmaceutical and Biological Products (Beijing, China). The purities of brucine (95.9%) and huperzine A (98.0%) were determined by HPLC analysis. Sephadex G-50 was purchased from Pharmacia Biotech (Uppsala, Sweden). HPLC-grade acetonitrile was obtained from Tedia Company Inc. (Fairfield, OH). All other solvents and reagents were of analytical grade.
The human coronary artery endothelial cells (HCAEC) were purchased from Kaiji Biotechnology Inc. (Nanjing, China). Cells were cultured at 37 °C in a 5% CO2/95% air humidified atmosphere in RPMI 1640 medium containing 25 mM HEPES and 2 mM l-glutamine supplemented with 20% (v/v) heat-inactivated FCS, 100 IU/mL penicillin and 100 mg/mL streptomycin. The HT1080 human fibrosarcoma cell line was obtained from American Type Culture Collection (Manassas, VA) and cultivated under the recommended conditions.
Female BALB/c nude mice were purchased from Shanghai Slac Laboratory Animal Co. Ltd (Shanghai, China) with the license number SCXK (Shanghai) 2007-0005. According to the requirement of the National Act on the Use of Experimental Animal (People’s Republic of China), the protocols of animal experiments were approved by the Animals Ethics Committee of Nanjing University of Chinese Medicine.
Preparation of NGR-modified stealth liposomes containing coumarin-6
The NGR-modified stealth liposomes containing coumarin-6 (NGR-SL-C) were prepared by the thin-film hydration method. Briefly, the mixture of PC, cholesterol, PEG-DSPE, NGR-DSPE-PEG and coumarin-6 were dissolved in chloroform and dried in a RE52A rotary evaporator (Yarong Biochemistry Instrument Company, Shanghai, China) in a round-bottom flask at 55 °C. The lipid film was flushed with nitrogen gas for 5 min and maintained overnight in a desiccator to remove traces of chloroform. The thin film was then hydrated by pH 7.4 PBS and the resulting multilamellar liposomes were sized by extrusion (10×) with a LIPEX Extruder (Northern Lipids Inc., Burnaby, Canada) at 55 °C through two stacked polycarbonated membrane filters with a pore size of 200 nm. For the preparation of stealth liposomes containing coumarin-6 (SL-C), an identical procedure was carried out except that the equivalent molar NGR-PEG-DSPE was replaced by PEG-DSPE.
Preparation of NGR-modified stealth liposomes containing brucine
The NGR-modified stealth liposomes containing brucine (NGR-SL-B) were prepared by the ammonium sulfate gradient loading method, as described previously (Chen et al., Citation2010). Briefly, the mixture of PC, cholesterol, PEG-DSPE and NGR-PEG-DSPE were dissolved in anhydrous ethanol and then injected into the solution of 5 mL of 200 mM ammonium sulfate with magnetically stirring at 55 °C. The ethanol was evaporated to no odor under vacuum, and then water was added to adjust the volume of final liposomes suspension to 5 mL. The resulting liposome suspensions were sized by extrusion (10×) with a LIPEX Extruder (Northern Lipids Inc., Burnaby, Canada) at 55 °C through two stacked polycarbonated membrane filters with a pore size of 200 nm. The transmembrane ammonium sulfate gradient was created by four consecutive dialysis exchanges (2 h at a time) against 20 vols. of phosphate buffer solution (PBS) (pH = 7.4) under room temperature. Brucine in powder form in a drug/lipid mass ratio of 1/6 was added to liposomes at 55 °C for 20 min. For the preparation of stealth liposomes containing brucine (SL-B), an identical procedure was carried out except that the equivalent molar NGR-PEG-DSPE was replaced by PEG-DSPE.
Characterization of NGR-SL-C with different PC composition
The particle size and zeta potential of NGR-SL-C or SL-C were measured by photon correlation spectroscopy using a Malvern Zetasizer Nano ZS90 (Malevern, UK). The entrapment efficiency (EE) was calculated by the percentage of coumarin-6 encapsulated into liposomes relative to the total amount of coumarin-6 (encapsulated and free) in liposome suspension, and the contents of coumarin-6 in liposomes were assayed by HPLC after the liposomes were dissolved in methanol.
A Shimadzu HPLC System (Kyoto, Japan) consisting of an LC-20AT pump, a RF-10AXL fluorescence detector was used for the assay of coumarin-6. The mobile phase consisted of methanol and water (95:5, v/v) and a flow rate of 0.8 mL/min was employed. Separation was carried out at 35 °C using a reverse-phase C18 column (Hibar, 5 μm, 4.6 mm × 150 mm, Merck Corp., Darmstadt, Germany). The excitation and emission wavelengths for fluorescence detection were 466 and 504 nm, respectively.
Due to its high hydrophobic nature, free coumarin-6 readily partitioned into the lipid membranes, leading to high cellular accumulation. Consequently, before the following cell uptake tests, unentrapped coumarin-6 was removed from NGR-SL-C by passing the liposome suspension through Sephadex G-50 gel column with pH7.4 PBS as eluent.
Flow cytometry analysis
HT1080 cells were seeded at a density of 1 × 106 cells/well in 6-well plates and incubated at 37 °C for 24 h to allow cell attachment. After 24 h, the medium was replaced with coumarin-6 solution, NGR-SL-C or SL-C formulations (the concentration of coumarin-6 was 100 ng/mL). After a 1 h incubation at 37 °C, the cells were washed three times with PBS. The cells were then harvested by trypsinization and centrifuged at 1000 rpm for 5 min and resuspended in 1 mL PBS medium and examined by flow cytometry using a FACScan (Becton Dickinson, San Jose, CA).
Confocal laser microscopy
Following incubation of HT1080 and HCAEC on glass-bottomed dishes containing culture medium at 37 °C for 24 h, coumarin-6 solution or NGR-SL-C formulations were added to each dish to obtain the final coumarin-6 concentration of 100 ng/mL and incubated for another 1 h at 37 °C. The medium was then removed and cells were washed with ice cold PBS followed by fixing with 4% paraformaldehyde for 10 min. After fixing, cells were treated with Hoechst 33258 for 5 min. The fluorescent images of the cells were analyzed using a TCS SP5 confocal microscope (Lecia, Wetzlar, Germany). Cell-associated coumarin-6 was excited with an argon laser (467 nm) and fluorescence was detected at 502 nm.
Characterization of NGR-SL-B with different PC composition
The particle size, zeta potential and EE values were determined as described in “Characterization of NGR-SL-C with different PC composition” section. To determine the total amount of brucine in liposome suspension, 0.5 mL NGR-SL-B or SL-B was disrupted by the addition of 2 mL ethanol:isopropanol (1:4, v/v) to form a clear solution. The concentration of brucine was assayed by UV spectroscopy (UV2800AH, Unic Instrument Company, Shanghai, China) at 264 nm compared to a standard curve. To completely remove unentrapped drug, NGR-SL-B was passed through a Sephadex G-50 column (1 × 27 cm) equilibrated with PBS (pH 7.4). The resulted PBS fraction containing the liposomes free of non-entrapped brucine was collected and the encapsulated brucine concentration was determined as described above.
HPLC analysis of brucine
A Shimadzu HPLC System (Kyoto, Japan) consisting of an LC-20AT pump, a SPD-20A UV-VIS detector was used for the assay of brucine in PBS. The mobile phase consisted of acetonitrile and buffer (10 mM sodium heptane sulfonate and 20 mM potassium dihydrogen phosphate, adjusted pH to 2.8 with 10% phosphonic acid). The ratio of acetonitrile/buffer (v/v) was adjusted to 24:76. Separation was carried out at 35 °C using a reverse-phase C18 column (Lichrospher, 5 μm, 4.6 mm × 250 mm, Hanbang Corp., Huaian, China). The detection wavelength was 264 nm and a flow rate of 1.0 mL/min was employed.
In vitro release of brucine from liposomes
The in vitro release profiles of NGR-SL-B with different lipid composition and SL-B were analyzed according to the published method (Chen et al., Citation2014). Non-entrapped drug was removed from NGR-SL-B over a Sephadex G-50 column as described above in vitro release test. To evaluate the effect of plasma albumin on drug release from liposomes, NGR-SL-B or SL-B suspension combined with fresh rat plasma (1:1, v/v) was placed into the dialysis bag with a molecular weight cut-off of 8000. The dialysis bag was suspended in 100 mL isotonic PBS (pH 7.4), which was incubated at 37 °C under constant rotation at 500 rpm. At scheduled time intervals, aliquot samples were withdrawn and assayed for brucine content by HPLC. A sample volume of 20 μL was injected. The volume of dissolution medium was maintained at 100 mL throughout the experiment.
In-vitro cytotoxicity assays
HT1080 cells were seeded in 96-well plates at a density of 5 × 103 cells/well (in triplicates). Twenty-four hours later, cells were washed once with PBS and exposed for 48 h to various concentrations of brucine solution, SL-B, NGR-SL-B with different PC compositions and the corresponding blank liposomes (as control) at 37 °C. Cell growth was assessed by MTT assay and the optical densities were measured at 490 nm. Cells that did not receive any drug (control) were considered as having 100% cell growth and growth from treated cells was compared with this value (percent growth versus control).
Bio-distribution of NGR-SL-DiR in tumor-bearing mice
The bio-distribution in HT1080 tumor-bearing female BALB/c nude mice was investigated using an in vivo imaging system (Luo et al., Citation2013). HT1080 tumor-bearing nude mice were prepared by injecting a suspension of HT1080 cells (4 × 106) into the subcutaneous in the right armpits of nude female BALB/c mice. Once the tumor masses in the xenografts reached 200 mm3 in volume, 200 μL of 5% glucose injection, the NGR-modified stealth liposomes containing DiR (NGR-SSL-DiR) or the stealth liposomes containing DiR (SSL-DiR) with different PC composition was intravenously administered via the tail vein of the tumor-bearing mice at a dose of 1.7 mg/kg. The mice were sacrificed at 20 h, and the major organs, including brain, heart, liver, spleen, lungs, kidneys and tumor were excised. The near-infrared fluorescence signal intensities in different tissues were measured using a Kodak In Vivo Imaging System FX PRO (CarestreamHealth, Inc., Rochester, NY) with an excitation bandpass filter at 730 nm and an emission at 790 nm. The fluorescent signal intensities in the tumor bearing mice were analyzed using Carestream MI SE software.
Results
Effect of the PC composition on properties of NGR-SL-C
Particle size, zeta potential and EE of the prepared formulations are shown in . The particle size of NGR-SL-C was approximately 200–250 nm and the polydispersity was below 0.15. The value of the zeta potential of liposomes had a negative charge and the EE value was all higher than 90%. Moreover, NGR modification and lipid composition seemed to have little effect on the particle size, zeta potential and EE values of liposomes.
Table 1. Characterization of coumarin-6 liposomes with different PC composition (n = 3).
Flow cytometry was applied to quantify the total coumarin-6 uptake by HT1080 and HCAEC cells for different coumarin-6 liposomes. As shown in , the cellular coumarin-6 level for NGR-SL-C-HS in the HT1080 cell lines was about 1.5-fold higher than for SL-C-HS. When the HT1080 cells were pre-incubated with free NGR for 30 min, the cellular coumarin-6 level of NGR-SL-C-HS was only 1.2-fold higher than that for SL-C-HS. As shown in , it was obvious that the uptake of NGR-modified liposomes varied with lipid composition. And the cells treated with NGR-SL-C-HS and NGR-SL-C-S both showed higher uptake compared to with NGR-SL-C-D and NGR-SL-C-H.
Figure 1. The flow cytometric measurement of coumarin-6 uptake from NGR-SL-C-HS and SL-C-HS by HT1080 (CD13+) cells at the time of 1 h. Cells were incubated with NGR-SL-C-HS and SL-C-HS at the final coumarin-6 concentration of 100 ng/mL, and the time point of 1 h, the cells were trypsinized, washed and analyzed using flow cytometry. 1 – Control, 2 – SL-C-HS, 3 – NGR+NGR-SL-C-HS, 4 – NGR-SL-C-HS.
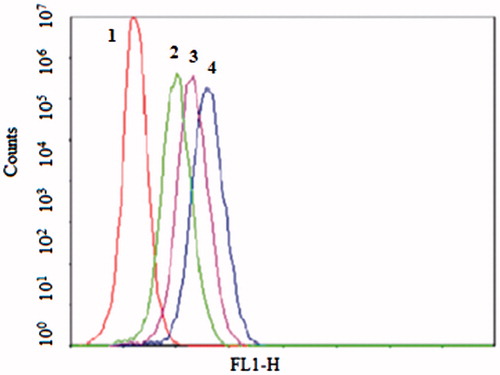
Figure 2. The flow cytometric measurement of coumarin-6 uptake from NGR-SL-C-HS, NGR-SL-C-S, NGR-SL-C-H and NGR-SL-C-D by HT1080 (CD13+) cells at the time of 1 h. Cells were incubated with liposomes at the final coumarin-6 concentration of 100 ng/mL. And the time point of 1 h, the cells were trypsinized, washed and analyzed using flow cytometry. 1 – Control, 2 – NGR-SL-C-D, 3 – NGR -SL-C-H, 4 – NGR-SL-C-HS, 5 – NGR-SL-C-S.

shows the confocal microscopic images of HT1080 cells after incubation with SL-C-HS, NGR-SL-C-HS, NGR-SL-C-S, NGR-SL-C-H and NGR-SL-C-D. The image of HCAEC cells incubation with NGR-SL-C-HS was also included. For NGR-SL-C-HS, the images showed a more intense fluorescence of coumarin-6 in HT1080 cells compared to other coumarin-6 liposomes. For HCAEC cells, little fluorescence of coumarin-6 was found after incubation with NGR-SL-C-HS, which indicated minimal uptake of NGR-modified liposomes by CD13− cells.
Figure 3. The confocal microscopy images of HT1080 (CD13+) and HCAEC (CD13−) incubated with different coumarin-6 liposomes for 1 h at 37 °C. Green represents fluorescence of coumarin-6, blue represents fluorescence of Hoechst 33258. (For interpretation of the reference to color in this figure legend, the reader is referred to the web version of this article.)
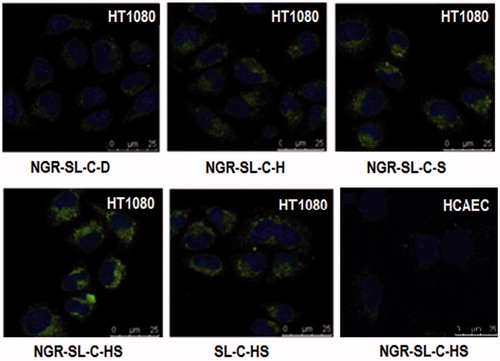
It was also found that the uptake of SL-C-HS by HT1080 cells was enhanced compared with that of NGR-SL-C-HR by HCAEC cells. They were both uptaken by non-specific endocytosis. But NGR modification seemed to offer the greater steric hinderance to reduce cellular uptake.
Effect of the PC composition on properties of NGR-SL-B
The particle sizes of NGR-SL-B with different lipid compositions are shown in . It could be observed that the lipid composition had little effect on the EE values. Polydispersity index was all lower than 0.15, indicating that liposome population was homogenous in size. The results of zeta potential measurements revealed that four liposomal formulations were all electrically negative.
Table 2. Characterization of brucine-loaded liposomes with different lipid composition (n = 3).
The in vitro drug release profiles are given in . With the existence of fresh rat plasma, the drug release ratios of NGR-SL-B-H, NGR-SL-B-D, NGR-SL-B-S, NGR-SL-B-HS and SL-B-HS in 10 h were 11.83 ± 1.20%, 13.46 ± 2.43%, 54.70 ± 4.17%, 45.58 ± 4.78% and 61.19 ± 5.11%, respectively. Compared with NGR-SL-B-S and NGR-SL-B-HS, the release ratios of NGR-SL-B-H and NGR-SL-B-D at 10 h were significantly decreased (p < 0.001). It was evident that the extent of drug retention in NGR-modified liposomes corresponded to the Tm of PC.
Figure 4. The release of brucine from NGR-SL-B with different lipid compositions and SL-B-HS incubated with fresh rat plasma at 37 °C (n = 3).
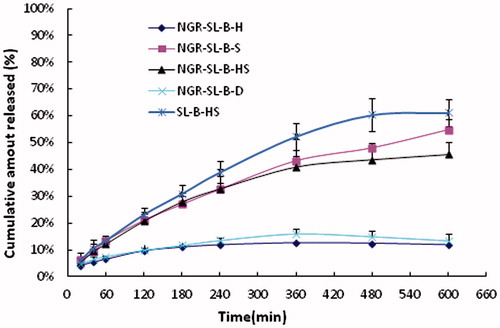
HT1080 cell proliferation was determined by MTT assay. As shown in , the results demonstrated that HT1080 proliferation was significantly inhibited by different brucine formulations. The cytotoxicity of NGR-SL-B-HS was found to be the highest, which was in accordance with the results of the uptake of NGR-SL-C-HS. The value of the viability% at 120 μg/mL was measured to be 42.57 ± 0.98%, 25.62 ± 1.37%, 25.30 ± 1.31% and 7.69 ± 0.80%, and 28.73 ± 3.39% for NGR-SL-H, NGR-SL-S, NGR-SL-D and NGR-SL-B-HS, respectively. Compared with NGR-SL-H, the anti-proliferative activity of NGR-SL-S, NGR-SL-D and NGR-SL-B-HS was significantly enhanced (p < 0.001).
Figure 5. Cytotoxicity of brucine-loaded liposomes with different lipid composition and brucine solution. After the addition of brucine formulations (NGR-SL-B-HS, SL-B-HS, NGR-SL-B-S, NGR-SL-B-H, NGR-SL-B-D or brucine solution) to cells, the mixture was incubated for 48 h and the viability of HT1080 cells was measured by MTT assay.
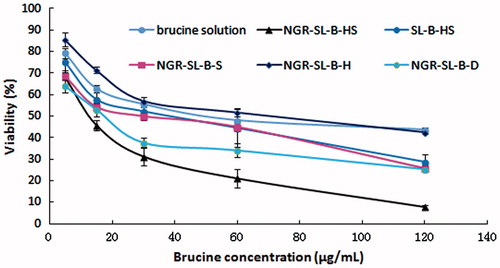
Bio-distribution of NGR-SL-DiR in tumor-bearing mice
shows the distribution and tumor accumulation of fluorescent DiR in the HT1080 tumor-bearing mice. The DiR fluorescence signal of the tumor tissue was strongest for the mice treated with NGR-SL-DiR-HS than those treated with SL-DiR-HS or NGR-SL-DiR composed of SPC, HSPC and DPPC. Moreover, PC composition also affected the distribution of NGR-modified liposomes in liver, spleen and lung, which contain mononuclear phagocyte system (MPS). Therefore, it is suggested that PC composition influence the PEG grafting density on the surface of liposomes.
Discussion
Brucine is the most potent antitumor alkaloid from Semen Strchyni, especially for the treatment of human hepatoma cells (Deng et al., Citation2006; Yin et al., Citation2007). It also possesses anti-angiogenic effects. The anti-angiogenic effects were accompanied with decreased vascular endothelial growth factor and tumor necrosis factor and increased interleukin-2 (Agrawal et al., Citation2011). Brucine could also inhibit the expression of vascular endothelial growth factor (VEGF) and microvessel density in nude mouse model of bone metastasis due to breast cancer (Li et al., Citation2012). Prepared by ammonium sulfate gradient methods, brucine, an active antitumor alkaloid, was encapsulated into the inner aqueous phase of NGR-modified liposomes. However, coumarin-6, a lipophilic fluorescent dye for the research of targeted drug delivery (Eley et al., Citation2004), was encapsulated into the lipid bilayer of the liposomes due to its high hydrophobicity. Consequently, the effect of PC composition revealed by the present study seemed to be non-specific to different drugs.
Immuno-histochemical staining showed that both tumor cells and endothelial cells of tumor blood vessels expressed CD13 and could bind with NGR in the HT1080 model. Though the expression of CD13 in tumor cells was not as strong as in blood endothelial cells, in the case that tumor cells also express CD13, it will further improve the tumor selectivity of NGR-modified liposomes. To our knowledge, in the present study, it is the first time that brucine was applied for the treatment of HT1080 cells.
The in vivo performance of NGR-modified liposomes was compared in terms of NGR density. The AUC values in tumor were determined to be 223 ± 93 and 164 ± 59 mg·h/mL for NGR-modified liposomes containing 0.64% and 2.56% NGR-PEG-DSPE, respectively (Dunne et al., Citation2011). Therefore, 0.64% NGR-PEG-DSPE was selected for the preparation of NGR-modified liposomes in the present study.
Liposomal encapsulation might alter tissue distribution of chemotherapeutic agents and selectively deliver drug into malignant zones, thus resulting in reduced toxicity and enhanced antitumor effects. It is well known that lipid composition affects properties of liposomes. Generally, liposomes composed of PC in a solid gel phase retain compounds more efficiently than liposomes composed of PC in a liquid phase. Therefore, liposomes with a higher Tm possess higher membrane stability and lower drug release rates compared to that with a lower Tm (Lindner & Hossann, Citation2010). The results revealed that drug release rate increase with the decrease of Tm of PCs. The drug release rates from liposomes with different PC could be ordered as follows: HSPC < DPPC < 1,2-Dimyristoyl-sn-glycero-3-phosphocholine (DMPC) (Li et al., Citation2008). In the present study, it had been demonstrated that lipid composition also affected the uptake efficiency of NGR-SL-C, the cytotoxicity of NGR-SL-B and the targeted delivery of NGR-SL-DiR. In the previous study (Takara et al., Citation2012), EPC and HSPC were applied for the preparation of rhodamine-labeled NGR-modified and NGR-modified doxorubicin (DOX), respectively. The behavior of these two liposomes was both used to prove the potential of the active targeted liposomes. However, based on the results of our study, since PC composition obviously affected the behavior of NGR-modified liposomes, the application of different PC (EPC and HSPC) to prepare dye-containing and drug-containing NGR-modified liposomes seemed to be unreasonable.
Recently, a liposomal formulation composed of naturally unsaturated EPC and hydrogenated egg phosphatidylcholine (HEPC) with significant Tm difference was developed for incorporate high paclitaxel content (Kan et al., Citation2011). HEPC is referred to a PC with long hydrocarbon chain and high Tm of 50–55 °C. On the contrary, naturally occurring EPC containing high content of unsaturated fatty acid chains is considered to have a lower Tm of −8 °C. The difference of Tm between the two PCs is estimated to be about 60 °C. For liposomes composed of either EPC or HEPC alone, the EE was decreased to about 42.1% at the drug/lipid molar ratio of 7%. But for the liposomes composed both EPC and HEPC, the EE remained above 80% when the drug/lipid molar ratio was even increased to 20%. It was speculated that the separated phases, a solid gel phase of HEPC and liquid crystal phase of EPC, was formed separately in liposomal membrane at a given temperature in the range of −8 to 50 °C. Accordingly, formation of the phase boundary was speculated to restrict the lateral diffusion across segregated domains, hindering the self-aggregation of hydrophobic molecules such as paclitaxel. Therefore, a large amount of paclitaxel could be incorporated into liposomal membrane. In our previous study, the mixture of HSPC and SPC was applied for the preparation of liposomes containing antitumor drugs to obtain higher EE of brucine (Chen et al., Citation2010). Therefore, the mixture of HSPC and SPC was also used for the preparation of NGR-modified liposomes. Unexpectedly, the highest targeted drug delivery efficiency was obtained. It seemed that the presence of a phase separation in liposomal membrane could improve the uptake of NGR-modified liposomes by HT1080 cells whether in vitro or in vivo.
Two mechanisms, endocytosis or fusion, were included in the process of cellular uptake of liposomes and lipid composition was proved to affect the rate of liposomes uptake and internalization by cells (Dini et al., Citation1998). The results of our study revealed that more fluidic liposomes showed higher cellular uptake performance. It is speculated that it is easier for fluidic liposomal membrane to fuse with the membrane of cells. Furthermore, the phase separation which enhanced the fluidity of liposomal membrane might also be beneficial for the cellular fusion.
Declaration of interest
The authors report no conflicts of interest in this work. The authors alone are responsible for the content and writing of the paper.
This work was financially supported by the National Nature Science Foundation of China (No. 81001644), Guangdong Nature Science Foundation (No. 10451040701004643), Research project of Jingchu University of Technology (No. ZR201505) and the Priority Academic Program Development of Jiangsu Higher Education Institutions.
References
- Agrawal SS, Saraswati S, Mathur R, Pandey M. (2011). Cytotoxic and antitumor effects of brucine on Enrlich ascites tumor and human cancer cell line. Life Sci 89:147–58
- Alavizadeh SH, Badiee A, Golmohammadzadeh S, Jaafari MR. (2014). The influence of phospholipid on the physicochemical properties and anti-tumor efficacy of liposomes encapsulating cisplatin in mice bearing C26 colon carcinoma. Int J Pharm 473:326–33
- Chen J, He CQ, Lin AH, et al. (2014). Brucine-loaded liposomes composed of HSPC and DPPC at different ratios: in vitro and in vivo evaluation. Drug Dev Ind Pharm 40:244–51
- Chen J, Lin AH, Chen ZP, et al. (2010). Ammonium sulfate gradient loading of brucine into liposomes: effect of phospholipid composition on entrapment efficiency and physicochemical properties in vitro. Drug Dev Ind Pharm 36:245–53
- Chen J, Ping QN, Guo JX, et al. (2006). Effect of phospholipid composition on characterization of liposomes containing 9-nitrocamptothecin. Drug Dev Ind Pharm 32:719–26
- Chen J, Yan GJ, Hu RR, et al. (2012). Improved pharmacokinetics and reduced toxicity of brucine after encapsulation into stealth liposomes: role of phosphatidylcholine. Int J Nanomed 7:3567–77
- Corti A, Ponzoni M. (2004). Tumor vascular targeting with tumor necrosis factor alpha and chemotherapeutic drugs. Ann NY Acad Sci 1028:104–12
- Deng XK, Yin FZ, Lu XY, et al. (2006). The apoptotic effect of brucine from the seed of Strychnos nux-vomica on human hepatoma cells is mediated via Bcl-2 and Ca2+ involved mitochondrial pathway. Toxicol Sci 91:59–69
- Dini L, Falasca L, Ruzzittu MT, et al. (1998). Interaction between isolated and purified liver cells and small unilamellar liposomes. Liver 18:229–38
- Dunne M, Zheng J, Rosenblat J, et al. (2011). APN/CD13-targeting as a strategy to alter the tumor accumulation of liposomes. J Control Release 154:298–305
- Eley JG, Pujari VD, McLane J. (2004). Poly(lactide-co-glycolide) nanoparticles containing coumarin-6 for suppository delivery: in vitro release profile and in vivo tissue distribution. Drug Deliv 11:255–61
- Fukasawa K, Fujii H, Saitoh Y, et al. (2006). Aminopeptidase N (APN/CD13) is selectively expressed in vascular endothelial cells and plays multiple roles in angiogenesis. Cancer Lett 243:135–43
- Garde SV, Forté AJ, Ge M, et al. (2007). Binding and internalization of NGR-peptide-targeted liposomal doxorubicin (TVT-DOX) in CD13-expressing cells and its antitumor effects. Anticancer Drugs 18:1189–200
- Kan P, Tsao C, Wang A, et al. (2011). A liposomal formulation able to incorporate a high content of paclitaxel and exert promising anticancer effect. J Drug Deliv 2011:629234
- Li C, Cui J, Wang C, et al. (2008). Lipid composition and grafted PEG affect in vivo activity of liposomal mitoxantrone. Int J Pharm 362:60–6
- Li P, Zhang M, Ma W, et al. (2012). Effects of brucine on vascular endothelia growth factor expression and microvessel density in a nude mouse model of bone metastasis due to breast cancer. Chinese J Integr Med 18:605–9
- Lindner LH, Hossann M. (2010). Factors affecting drug release from liposomes. Curr Opin Drug Disc 13:111–23
- Luo LM, Huang Y, Zhao BX, et al. (2013). Anti-tumor and anti-angiogenic effect of metronomic cyclic NGR-modified liposomes containing paclitaxel. Biomaterials 34:1102–14
- Negussie AH, Miller JL, Reddy G, et al. (2010). Synthesis and in vitro evaluation of cyclic NGR peptide targeted thermally sensitive liposome. J Control Release 143:265–73
- Pastorino F, Brignole C, Marimpletri D, et al. (2003). Vascular damage and anti-angiogenic effects of tumor vessel-targeted liposomal chemotherapy. Cancer Res 63:7400–9
- Senior J. (1982). Is half-life of circulating liposomes determined by changes in their permeability? FEBS Lett 145:109–14
- Takara K, Hatakeyama H, Kibria G, et al. (2012). Size-controlled, dual-ligand modified liposomes that target the tumor vasculature show promise for use in drug-resistant cancer therapy. J Control Release 162:225–32
- Xie X, Yang Y, Yang Y, et al. (2015). A photo-responsive peptide- and asparagine-glycine-arginine (NGR) peptide-mediated liposomal delivery system. Drug Deliv [Epub ahead of print]. doi: 10.3109/10717544.2015.1008707
- Yin W, Deng XK, Yin FZ, et al. (2007). The cytotoxicity induced by brucine from the seed of Strychnos nux-vomica proceeds via apoptosis and is mediated by cyclooxygenase 2 and caspase 3 in SMMC 7721 cells. Food Chem Toxicol 45:1700–8