Abstract
The development of mucoadhesive lipidic nanoemulsion based on hyaluronic acid, co-encapsulating two polyphenols (resveratrol and curcumin) for the transnasal treatment of neurodegenerative diseases was attempted in the current manuscript. Nanoemulsions were prepared by the spontaneous emulsification method, and were characterized for their particle size, zeta potential, mucoadhesive strength and morphology. The selected formula was tested for its antioxidant potential, in vitro and ex vivo release of the two polyphenols, safety on nasal mucosa and in vivo quantification of the two drugs in rat brains. Its stability was tested by monitoring the change in particle size, zeta potential, drugs' content and antioxidant potential upon storage for 3 months. The optimized hyaluronic acid based nanoemulsion formula displayed a particle size of 115.2 ± 0.15 and a zeta potential of −23.9 ± 1.7. The formula displayed a spherical morphology and significantly higher mucoadhesive strength compared to its non mucoadhesive counterpart. In addition, the nanoemulsion was able to preserve the antioxidant ability of the two polyphenols and protect them from degradation. Diffusion controlled release of the two drugs was achievable till 6 hours, with an ex vivo flux across sheep nasal mucosa of 2.86 and 2.09 µg/cm2hr for resveratrol and curcumin, respectively. Moreover, the mucoadhesive nanoemulsion was safe on nasal mucosa and managed to increase the amounts of the two polypehnols in the brain (about 7 and 9 folds increase in AUC0–7 h for resveratrol and curcumin, respectively). Hyaluronic acid based lipidic nanoemulsion proved itself as a successful carrier enhancing the solubility, stability and brain targetability of polyphenols.
Introduction
Site-specific targeting has always been advantageous in drug delivery, since it reduces the exposure of non-target organs to the effect of drugs, with increased therapeutic efficacy achieved by localization. However, achieving site-specific targeting varies in difficulty according to the location of the organ and the protective barriers surrounding it. A typical example of a “difficult to reach organ” is the brain. However, owing to the fact that the brain and nose are connected via the olfactory region of the nasal mucosa, targeting of the brain via the nose was proven to be a very successful approach for overcoming the limitation of drug uptake by the brain caused by the lack of paracellular openings in the blood-brain barrier and the absence of pinocytosis (Kumar et al., Citation2008; Gabal et al., Citation2014).
Neurodegenerative diseases are very commonly encountered with the aging population, in which Alzheimer's disease and Parkinsonism are the most common (Sahni et al., Citation2011; Gregori et al., Citation2015). The concept of nose to brain targeting in treatment of neurodegenerative disorders has benefited to a great deal from nanotechnology, provided that these nanocarriers overcome the rapid mucociliary clearance and poor nasal absorption (Sood et al., Citation2013, Citation2014). Trans-nasal brain delivery of nanoparticles offers many advantages, such as ease of self-administration, non-invasiveness as well as effective brain targeting.
Oil in water nanoemulsions, composed of nanosized oil droplets dispersed in an aqueous phase, represent a promising delivery system for encapsulation of water insoluble drugs, their stabilization in aqueous media, protection of drugs from degradation, controlling their release and enhancing their bioavailability (Sessa et al., Citation2014). Despite the reported benefits of microemulsions, lipidic nanoemulsions are preferred to the former in that they require lesser amount of surfactants and cosurfactants for their formation (Mahajan & Dinger, Citation2011). Many papers refer to microemulsions as nanoemulsions based on their nanometer size of the globules; however, in the current manuscript, nanoemulsions will strictly refer to lipidic nanoemulsions.
Among the naturally occurring polyphenolic phytochemicals used in the treatment of neurodegenerative disorders, most promising ones are resveratrol and curcumin. Resveratrol is the most biologically active compound found in grapes and red wine, while curcumin is the bioactive component of the rhizomes of Curcuma longa. The antioxidant activity of the polyphenols curcumin and resveratrol provides the pharmacological basis for their use in many diseases especially neurodegenerative ones (Vanaja et al., Citation2013). Curcumin was reported to affect the amyloid-β pathway by reducing the formation of amyloid-β fibrils and inhibiting its aggregation (Sahni et al., Citation2011). It also exhibits cytoprotective and anti-inflammatory properties through protection of the cells against amyloid-β-induced toxicity and reduction of the expression of cytokines and chemokines, with improvement in memory and cognitive defects, leading to reversal of neurodegeneration (Agrawal et al., Citation2010; Sahni et al., Citation2011; Monroy et al., Citation2013). Similarly, resveratrol was reported to affect the amyloid-β pathway by reducing the production of amyloid-β peptides and remodeling of the soluble oligomers and fibrils into non-toxic forms of amyloid-β, in addition to its cytoprotective actions and reduction of cognitive defects (Sahni et al., Citation2011). Resveratrol was also reported to decrease reactive oxygen species and lipid peroxides levels in animal models, in addition to its activation of sirtuin 1, which is an important therapeutic target for treatment of age-related neurodegenerative diseases (Sharma & Gupta, Citation2002; Kumar et al., Citation2007; Sun et al., Citation2010). The co-utilization of the two polyphenols was reported to result in positive synergism in their antioxidant activity (Aftab & Vieira, Citation2010). Despite their reported efficacy, both drugs suffer from bioavailability problems, owing to their poor water solubility, instability and metabolism (Chen et al., Citation2013; Vanaja et al., Citation2013; Amri et al., Citation2014; Basavaraj & Betageri, Citation2014; Sood et al., Citation2014). The encapsulation of these two polyphenols using pharmaceutical carriers has provided a solution to these problems (Vanaja et al., Citation2013).
Therefore, the aim of the current work is to test the feasibility of co-encapsulating the two polyphenols, resveratrol and curcumin in a mucoadhesive lipidic nanoemulsion as a carrier system for enhancing their transnasal brain delivery.
Materials and methods
Materials
Curcumin, 2,2-diphenyl-1-picrylhydrazyl (DPPH), mucin from procine stomach, HPLC grade water and acetonitrile were purchased from Sigma (St. Louis, MO). Resveratrol was purchased from Skin Actives Scientific (Glibert, AZ). Labrafac Lipophile WL 1349 and Labrafac PG were kindly gifted by Gattefosse' (Lyon, France). Cremophor RH was kindly gifted by BASF (Ludwigshafen, Germany). Tween 80 and orthophosphoric acid were purchased from El-Nasr Pharmaceutical Company (Cairo, Egypt). Cellulose membrane (M.wt. cut off 12 000–14 000) was purchased from Spectrum Laboratories (Rancho Dominguez, Ontario, Canada). Hyaluronic acid (HA-EP1) was kindly gifted by Shandong Freda Biopharm Company (Jinan, China). Uranyl acetate-2-hydrate was purchased from Allied Signal (Riedel-de haen, Seelze, Germany).
Preparation of nanoemulsions
Prior to the preparation of nanoemulsions, selection of the proper surfactants/oil combinations was done based on conduction of preliminary solubility experiments and previously reported research work by other authors (Amri et al., Citation2014; Sood et al., Citation2014). Labrafac Lipophile and Labrafac PG were selected as the oily phases, and tween 80 and Cremophor RH 40 were selected as the surfactants for nanoemulsion preparation. The aforementioned excipients were safely utilized for transnasal delivery (Sood et al., Citation2014). Fifty milligrams of each of curcumin and resveratrol were added to glass vials containing appropriate amounts of oil/surfactant, as shown in . Vials were placed in a thermostatically controlled shaking water bath at 37 °C for 72 h at 100 rpm (Kottermann GmbH, Uetze/Hanigsen, Germany) to reach equilibrium. Preparation of nanoemulsions was carried out using the spontaneous emulsification method by the portion-wise addition of the vial contents to 40 ml of distilled water with continuous magnetic stirring for 2 h, for the formation of o/w nanoemulsions (Lovelyn & Attama, Citation2011; Davidov-Pardo & McClements, Citation2015). The selected nanoemulsion formula was modified by hyaluronic acid incorporation in the aqueous phase at a concentration of 1.5% w/v. Preparation of nanoemulsions was conducted in the dark to avoid the conversion of resveratrol from the trans to the cis structural isomer, which occurs in solution owing to its extreme photosensitivity (Amri et al., Citation2012, Citation2014).
Table 1. Composition of nanoemulsion formulae.
Characterization of the prepared nanoemulsions
Measurement of particle size and zeta potential of nanoemulsions
The particle size and the zeta potential of the selected nanoemulsions were measured using the Zetasizer (Nano ZS 3600, Malvern Instruments Ltd., Worcestershire, UK) (Nasr et al., Citation2012).
Measurement of the pH of nanoemulsions
The pH of the prepared nanoemulsions was measured using a pH meter (model 3510, Jenway, Burlington, NJ).
Development of HPLC method for co-determination of curcumin and resveratrol
A method was developed in the current manuscript, for the co-analysis of both curcumin and resveratrol using HPLC (Agilent 1200, Minneapolis, MN), which is a modification of the method developed by Moorthi et al. (Citation2013) for quantification of curcumin. The mobile phase (water containing 1% orthophosphoric acid:acetonitrile at a ratio of 45:55 v/v) was delivered with a flow rate of 1.2 ml/min at 35 °C, and separation was performed using an Agilent Eclipse column XDB-C18 (5 µm, 4.6 × 150 mm) with sample injection volume of 10 µl. The wavelength for detection of resveratrol was 304 nm, representing the maximum UV absorbance of trans-resveratrol (Amri et al., Citation2014), while that of curcumin was 425 nm. Calibration curves for the two drugs were carried out in methanol (for calculation of drugs' content) and in phosphate buffered saline pH 6 containing 2% tween 80 (for in vitro release and ex vivo diffusion experiments).
Measurement of the mucoadhesive strength
The mucoadhesive strength of the selected formulae was tested using texture analyzer (TAXT Plus, Stable Micro Systems, Surrey, UK), by measuring the force required to detach mucin discs from the formulae. The formulae were kept at the lower platform, while mucin discs were attached to the upper probe of the device. The upper probe was lowered at a speed of 1 mm/s to touch the surface of the formulae, with an applied force of 1 N for 3 min and a deformation of 20 mm (Gabal et al., Citation2014).
Morphology of selected formulae
The morphology of the selected nanoemulsion formulations was visualized by transmission electron microscopy TEM (Jeol, JEM 1010, Tokyo, Japan), after being adsorbed to a carbon-coated grid and negatively stained with 2% uranyl acetate aqueous solution.
DPPH antioxidant assay
In order to verify that the encapsulation within nanoemulsion does not compromise the inherent antioxidant ability of the two polyphenols, the antioxidant potential of the two drugs in the encapsulated form was assessed by their free radical scavenging ability of 2,2-diphenyl-1-picrylhydrazyl (DPPH) (Caddeo et al., Citation2013) and compared to that of the non-encapsulated drugs. A methanolic DPPH solution was prepared, of which 3.9 ml were mixed with either 0.1 ml nanoemulsion formulation or 0.1 ml of the equivalent amount of drugs dissolved in methanol. The antioxidant activity was calculated according to the following equation, in which absorbance measurement was conducted at 517 nm (Tavano et al., Citation2014):
In vitro release of the two polyphenols from the selected nanoemulsion formula
In vitro release of the two drugs was performed using the membrane diffusion technique (Nasr et al., Citation2008a,Citationb). Two milliliters of the selected mucoadhesive nanoemulsion formula was placed in glass cylinders (7 cm in length, 2.5 cm in diameter) fitted with the cellulose membrane downside. The cylinders were clamped to the shaft of a USP dissolution apparatus (Pharma Test, Hainburg, Germany) and allowed to touch the surface of the dissolution medium (100 ml of PBS pH 6 containing 2% tween 80 to achieve sink conditions for the two drugs). The cylinders were rotated at 100 rpm at 34 °C, with samples withdrawn from the dissolution medium at definite time intervals with replacement. The withdrawn samples were analyzed for the concentration of the two drugs using the HPLC method previously described.
Ex-vivo diffusion of the two polyphenols from the selected nanoemulsion formula
Ex-vivo diffusion study of the two drugs from the selected mucoadhesive formula was conducted using freshly isolated sheep nasal mucosa collected from a local slaughter house, after cleaning the mucosa from the adhered tissues (Sood et al., Citation2013, Citation2014). The nasal mucosa was clamped on a Franz diffusion apparatus (model Variomag Telesystem, H + P Labortechnik, Munich, Germany). The diffusion area of the cells was 1.77 cm2 and the receptor medium was 7.5 ml of phosphate buffered saline pH 6 containing 2% tween 80, which was stirred at 100 rpm at 34 °C. A constant volume (0.1 ml) of the selected nanoemulsion formulation was placed in the donor compartment, with samples withdrawn from the receptor compartment via a sampling port at definite time intervals with replacement. All samples were filtered with membrane filter of pore size 220 nm before injection into the HPLC column for analysis of the two drugs. The permeation profile of both drugs was constructed by plotting the amount of drug permeated per unit skin surface area (µg/cm2) versus time, with the calculation of the steady state flux (Jss, µg/cm2h) from the slope of the linear part of the plot by linear regression analysis.
Stability of the selected nanoemulsion formula
Stability of the selected nanoemulsion formula was assessed by monitoring the change in particle size and zeta potential, after refrigeration storage for 3 months. In addition, the antioxidant potential of the nanoemulsion formula was assessed using the DPPH test and the value of DPPH inhibition percentage was compared to that obtained with the freshly prepared formula. The content of the two drugs was also calculated after dissolving an aliquot of the nanoemulsion in methanol and calculating the amounts of the two drugs after 3 months storage.
Histology of the treated nasal mucosa
A group of three male albino rats received 50 µl of the selected formula in the left nostril for seven consecutive days using a micropipette, to assess toxicity related to multiple administrations, with the right nostril considered as the control. Autopsy samples of the nasal septum with the epithelial cell membrane were fixed in 10% formol saline for 24 h, followed by washing in tap water, then serial dilutions of alcohol for dehydration. Nasal specimens were cleared in xylene and then embedded in paraffin in hot air oven at 56 °C for 24 h (Nasr et al., Citation2011, Citation2013). Paraffin bees wax tissue blocks were prepared for sectioning at 4 µm by slidge microtome (Rotary Leica RM2245, Buffalo Grove, IL). The obtained tissue sections were mounted on glass slides then deparaffinized, followed by hematoxylin and eosin staining for histopathological examination using a light microscope (Axiostar Plus, Zeiss, Thornwood, NY).
In vivo quantification of the two polyphenols in the brain
For analysis of the drugs content in brain homogenate samples, calibration curve of the two drugs was carried out in acetonitrile. Dissection of brain tissues from male Albino rats (220–250 g) after killing them was performed, followed by removal of adhering tissue, weighing and washing twice using distilled water. Brain homogenates were obtained by homogenization of the brain tissue with three folds its volume distilled water at 25 000 rpm for 1 min (IKa T25 Ultra-turrax homogenizer, Jahnke und Kinkel, Staufen, Germany). A total of 300 µl of the brain homogenate was mixed with 700 µl of different concentrations of both drugs in acetonitrile, followed by vortex mixing for 30 s and centrifugation at 9000 rpm at 8 °C for 15 min (Cooling centrifuge, Hermle Labortechnik GmbH, Wehingen, Germany).The supernatant was filtered and analyzed for drugs content using the HPLC method described previously.
The protocol for the conducted animal experiments was approved by the Research Ethics Committee of the Faculty of Pharmacy, Ain Shams University (approval number REC-ASU7). Quantification of the amount of resveratrol and curcumin reaching the brain was made at different time intervals following the intranasal administration of the selected nanoemulsion, compared to an equivalently administered amount of the solution of both drugs with the aid of 10% cremophor RH40. The study was conducted on Albino rats weighing 220–250 g, with six rats tested for each time point. Rats were held in slanted position and 50 µl of the selected nanoemulsion formulation or the aqueous solution was administered in one nostril, with the help of a micropipette attached with a tubing of 0.1 mm internal diameter (Kumar et al., Citation2008).
Dissection of rat brains was performed at time intervals up to 7 h (5 min, 10 min, 15 min, 30 min, 1 h, 2 h, 4 h and 7 h). Brain homogenate samples were stored at −20 °C till analysis. Quantification of the drugs in the brain tissues was performed by mixing an amount of 300 µl of brain homogenate with 700 µl of acetonitrile, followed by vortex mixing for 30 s and centrifugation at 9000 rpm at 8 °C for 15 min. The supernatant was filtered and analyzed for drugs content using the HPLC method previously described.
Statistical and pharmacokinetic analysis of the data
The mean values and standard deviations were calculated for all experiments carried out in triplicate. Values were tested for their statistical significance using the ANOVA followed by the Tukey-Kramer post-hoc test at p ≤ 0.05 using Graph pad Instat software version 3.06 (GraphPad Software, Inc., La Jolla, CA). Pharmacokinetic calculations were performed on each set of data using Kinetica software version 5.0 (Innaphase, Philadelphia, PA).
Results and discussion
Preparation of nanoemulsions
Nanoemulsions co-encapsulating resveratrol and curcumin were successfully prepared at 10% surfactant concentration, while the 5% was insufficient for solubilization of both drugs. Furthermore, the only successful oil/surfactant combination for achieving complete solubilization of the two drugs was Labrafac Lipophile/tween 80 (formula N2), Labrafac Lipophile/cremophor 40 (formula N4) and Labarafac PG/tween 80 (formula N6). Therefore, these aforementioned formulae proceeded to the next characterization step.
Characterization of the prepared nanoemulsions
Measurement of particle size and zeta potential of nanoemulsions
As shown in , the particle size of formulae N2, N4 and N6 was 229.5 ± 13.7, 105.1 ± 0.64 and 176.3 ± 3.5 nm, respectively. Their respective zeta potential values were −18.6 ± 0.14, −19 ± 0.74 and −18.5 ± 1.77. PDI values were 0.36 ± 0.01, 0.16 ± 0.03 and 0.17 ± 0.03 for N2, N4 and N6, respectively. The small nanometer size with the relative monodispersity of the nanoemulsion particles (PDI less than 0.4) is considered advantageous. Coradini et al. (Citation2014) reported that co-encapsulation of the two polyphenols curcumin and resveratrol in lipid core nanocapsules at a concentration of 1 mg/ml produced micrometer-sized particles demonstrating the higher solubilizing capacity of the prepared nanoemulsions.
Table 2. Particle size, polydispersity index and zeta potential of the selected nanoemulsion formulae.
Given the aforementioned results, the smallest particle size and polydispersity index was achieved with formula N4. Therefore, this formula was selected for hyaluronic acid incorporation (N9). The aforementioned formula displayed a particle size of 115.2 ± 0.15, a zeta potential value of −23.9 ± 1.7, and a PDI of 0.235 ± 0.01. The significant increase in particle size upon inclusion of hyaluronic acid in the aqueous phase is attributed to the hyaluronic acid coat on the particles (to be displayed in the electron microscopy pictures), in addition to the increased viscosity of the external phase of the nanoemulsion due to the presence of hyaluronic acid, leading to larger sized particles (Ibrahim et al., Citation2010). The increase in the negativity is also attributed to the presence of hyaluronic acid in the formulation (Shen et al., Citation2015).
Measurement of the pH of the selected formulae
The pH of both formulae ranged from 6.6–6.7.
Development of HPLC method for co-determination of curcumin and resveratrol
The calibration curves obtained were linear in the tested concentration ranges (0.1–100 µg/ml) in both methanol and PBS pH 6 with r2 = 0.9994 and 0.9992 for resveratrol and r2 = 0.9978 and 0.9995 for curcumin in the aforementioned media, respectively. Validation parameters of the method are described elsewhere (data not shown).
Measurement of the mucoadhesive strength
The mucoadhesive forces of formula N4 and its hyaluronic acid-based counterpart N9 were 0.12 ± 0.02 and 0.67 ± 0.01 N, respectively, with respective work of adhesion of 0.27 ± 0.22 and 12.53 ± 0.08 mJ. The aforementioned values prove that hyaluronic acid incorporation was able to enhance the mucoadhesiveness of the nanoemulsion, which is a desirable property for intranasal administration to reduce mucociliary clearance and increase the residence time of the formula in the nasal cavity (Horvát et al., Citation2009).
Morphology of the selected nanoemulsion formulae
Formulae N4 and its mucoadhesive counterpart N9 were selected for morphological examination (, respectively). The spherical morphology and the nanometer size of the prepared nanoemulsions was confirmed, with a hyaluronic acid coat commonly present in formula N9 ().
DPPH antioxidant assay
The degree of discoloration of the DPPH solution is taken as index of the free radical scavenging potential of drugs or formulations (Caddeo et al., Citation2013). The retention of antioxidant capabilities of the two polyphenols is essential to ensure their mechanism of therapeutic action in neurodegenerative diseases. The averaged DPPH inhibition % for the polyphenols solution, formula N4 and formula N9 were 86.02 ± 3.32%, 82.46 ± 1.52% and 83.59 ± 0.54%, respectively. As obvious from the results, no statistical difference in DPPH inhibition percentage was observed upon encapsulation of the two polyphenols in the nanoemulsion and its hyaluronic coated counterpart (p > 0.05). This came in accordance with Sessa et al. (Citation2011), who stated that nanoemulsions preserved the antioxidant potential of resveratrol. Worthy to note that the liquid nature of the utilized lipid “Labrafac lipophile” was superior to solid matrix nanoemulsions encapsulating curcumin, where the lipid phase was stearic or palm oil, in which the latter led to a significant reduction in the antioxidant activity of curcumin despite the preservation of its stability (Donsi et al., Citation2011).
In vitro release of the two polyphenols from the selected nanoemulsion formula
illustrates the in vitro release behavior of resveratrol and curcumin from the selected formula N9. As evident from the figure, the in vitro release behavior was comparable for both drugs, in which the cumulative percentage of drug released was statistically insignificant at almost all time points (p > 0.05). This may be attributed to the close log D value of both drugs, being 3.1 for resveratrol and 2.9 for curcumin (Coradini et al., Citation2014). After 6 h, approximately 60% of both drugs were released from the formula following a diffusion-controlled mechanism.
Ex-vivo diffusion of the two polyphenols from the selected nanoemulsion formula
illustrates the amount of resveratrol and curcumin permeated per unit surface area of the skin versus time. The flux for resveratrol and curcumin was found to be 2.86 and 2.09 µg/cm2 h, respectively, proving that the hyaluronic acid containing formula allowed the penetration of the two drugs through the nasal mucosa. There was no statistically significant difference between the cumulative amount of resveratrol and curcumin permeated after 6 h (p > 0.05), with the release following a diffusion-controlled mechanism similar to what was obtained in the in vitro release experiment.
Stability of the selected nanoemulsion formula
The stability of formula N9 which was selected for the in vivo brain pharmacokinetic study was tested, by assessing the change in particle size, zeta potential and the content of the two drugs after three months storage. Results are shown in . Values of particle size, zeta potential and PDI were 114.3 ± 0.14, −21.8 ± 1.77 and 0.252 ± 0.015, suggesting that formula N9 was able to maintain its properties and resist aggregation following refrigeration storage.
Figure 4. The effect of three months storage of formula N9 on its particle size, zeta potential and DDPH inhibition percentage.
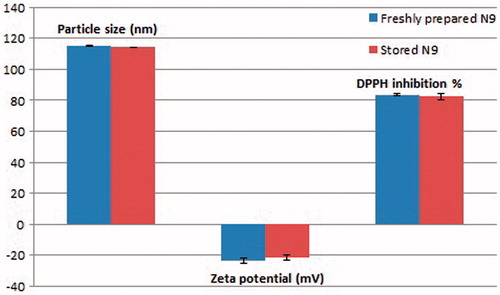
Drugs' content experiment proved that the amount of the loaded drugs did not change significantly from the freshly prepared nanoemulsion upon storage (p < 0.05). The DPPH inhibition % for formula N9 re-measured after three months storage was 82.35 ± 2.21. The preservation of the antioxidant activity after three months storage in nanoencapsulated form suggests that lipidic nanoemulsions can present a solution to the problem of instability of resveratrol, which was reported to be easily oxidized (Frozza et al., Citation2011). This also came in accordance with several other authors (Lucas-Abellán et al., Citation2007; Caddeo et al., Citation2008; Coimbra et al., Citation2011; Sessa et al., Citation2014), who declared that encapsulation of resveratrol in carriers, such as cyclodextrin complexes, liposomes and lecithin-based nanoemulsions were able to improve the stability of resveratrol. Similarly, this could be applied to curcumin, in which authors reported that its encapsulation in nanoformulations improves its stability (Rachmawati et al., Citation2015). The fact that the prepared nanoemulsion was able to maintain the concentration of the two polyphenols with no degradation, and preserve their antioxidant activity, proves that nanoemulsions provide an inert environment for both polyphenolic drugs.
Histology on nasal mucosa
As evident in , the integrity of the lining epithelium of the nasal cavity was maintained upon repeated administration of formula N9, with no inflammatory cellular infiltration in the lamina propria of the mucosal lining. Only mild congestion and dilatation of the blood vessels was apparent, which is sometimes encountered with surfactant containing formulae (Abdel-Bar et al., Citation2013; Bshara et al., Citation2014). The cartilaginous tissue underneath the epithelium was found to be normal as well, which suggests that the utilized formula was tolerable when tested on rat nasal tissues. This came in accordance with Hórvat et al. (Citation2009), who stated that the combination of cremophor RH 40 and hyaluronic acid in intranasal formulations did not cause any tissue damage or epithelial and ciliotoxicity.
In vivo quantification of the two polyphenols in the brain
The drug uptake into the brain from the nasal mucosa occurs via two pathways; the systemic route by which the fraction of the drug absorbed into the blood reaches the brain by crossing the blood-brain barrier, the trigeminal neural pathway which causes direct transport of the drug to the central nervous system and finally via the olfactory pathway. However, the presence of ABC transporters, such as Pgp and BCRP at the blood-brain barrier tends to reduce the brain penetration of many drugs (Loscher & Potschka, Citation2005).
When intranasally administered, the solution of resveratrol and curcumin allowed the absorption of both drugs by passive diffusion, owing to their lipophilicity, as shown in and illustrated in . However, resveratrol was reported to be affected by the BCRP efflux pump, hence limiting its bioavailability (Scheepens et al., Citation2010). Curcumin was advantageously reported to lower the expression of p-glycoprotein transporters, as well as inhibit BCRP transporters in vivo (Holland et al., Citation2006; Nabekura, Citation2010; Kusuhara et al., Citation2012), and hence, the combination of curcumin and resveratrol in the same formula is expected to have facilitated the brain delivery of resveratrol. As illustrated in , the significantly lower amounts of the two drugs reaching the brain following intranasal administration of their aqueous solution could be ascribed to its rapid mucociliary clearance as well as its possible nasal drainage (Gabal et al., Citation2014). On the contrary, the significantly higher levels of the two drugs (p < 0.05) reaching the brain at various time intervals following the administration of the mucoadhesive formula N9 was observed (about 7- and 9-fold increase in AUC0–7h for resveratrol and curcumin, respectively), which is attributed to the lipidic matrix of the nanoemulsion. This is expected to have favored the partitioning of the nanoemulsion into the lipid bilayer of the nasal epithelial cells, in addition to its content of the permeation enhancer cremophor RH 40, which is known for its enhancement of cell membrane fluidity (Horvát et al., Citation2009). In addition to its mucoadhesive nature which delays the mucociliary clearance, hyaluronic acid was reported to enhance the absorption of drugs via mucosal tissues (Horvát et al., Citation2009). The combination of cremophor RH 40 and hyaluronic acid was reported to increase the nose to brain transport of fluorescein isothiocyanate through the olfactory pathway (Horvát et al., Citation2009). The non-significant difference in AUC values for resveratrol and curcumin (p > 0.05) may be attributed to the close log D values of the two drugs. Worthy to note that the concentrations obtained in the brain for the two drugs lied in the therapeutic range, in which curcumin was reported to inhibit the formation of amyloid β oligomers at concentrations of 0.1–1 µM, and resveratrol was reported to exhibit neuroprotective activity against Aβ induced toxicity at 5 µM concentration (Yang et al., Citation2005; Ma et al., Citation2014), suggesting the promising nature of our described nanoemulsion in achieving therapeutically relevant brain targetability.
Figure 6. Quantification of the amounts of resveratrol and curcumin reaching the brain following the intranasal administration of the mucoadhesive formula N9.
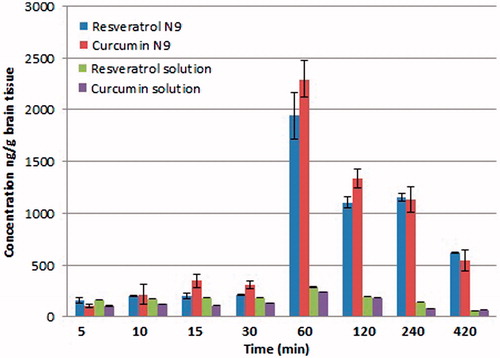
Table 3. Brain pharmacokinetic parameters for intranasally administered resveratrol and curcumin solution compared to the mucoadhesive nanoemulsion form N9.
Conclusion
The hyaluronic acid-based lipidic nanoemulsion system proved to be a promising carrier for transnasal brain delivery of the two polyphenols curcumin and resveratrol, owing to their lipidic nature, surfactant content, small particle size and mucoadhesiveness. The merits for its co-encapsulation of the aforementioned polyphenols were two-fold; in which it was able to preserve their antioxidant potential and protect them against degradation, while increasing their concentrations in the brain compared to the solution form of the drugs. The low concentration of surfactant employed in their preparation compared to that of microemulsions makes lipidic nanoemulsions favorable in terms of safety. Futuristic studies will be conducted involving the development of several models for neurodegenerative diseases to test the pharmacodynamic efficacy of the described system as well as the synergism between curcumin and resveratrol in these diseases.
Acknowledgements
The author would like to thank Gattefosse' Company (France) for their kind supply of Labrafac Lipophile WL 1349 and Labrafac PG. The author would also like to thank BASF Company (Germany) for their kind supply of Cremophor RH. In addition, special thanks to Shandong Freda Biopharm Company for their kind supply of hyaluronic acid. The author is also thankful to Professor Adel Bakir (Faculty of Veterinary medicine, Cairo University) for his help in the histopathological examination of the nasal samples.
Declaration of interest
The author has no other relevant affiliations or financial involvement with any organization or entity with a financial interest in or a financial conflict with anything related to the manuscript. No writing assistance was utilized in the production of the manuscript. The author reports no other conflicts of interest in this work.
References
- Abdel-Bar HM, Abdel-Reheem AY, Awad GA, Mortada ND. (2013). Evaluation of brain targeting and mucosal integrity of nasally administrated nanostructured carriers of a CNS active drug, clonazepam. J Pharm Pharm Sci 16:456–69
- Aftab N, Vieira A. (2010). Antioxidant activities of curcumin and combinations of this curcuminoid with other phytochemicals. Phytother Res 24:500–2
- Agrawal R, Mishra B, Tyagi E, et al. (2010). Effect of curcumin on brain insulin receptors and memory functions in STZ(ICV) induced dementia model of rat. Pharmacol Res 61:247–52
- Amri A, Chaumeil JC, Sfar S, Charrueau C. (2012). Administration of resveratrol: what formulation solutions to bioavailability limitations? J Control Release 158:182–93
- Amri A, Le Clanche S, Therond P, et al. (2014). Resveratrol self-emulsifying system increases the uptake by endothelial cells and improves protection against oxidative stress-mediated death. Eur J Pharm Biopharm 86:418–26
- Basavaraj S, Betageri GV. (2014). Improved oral delivery of resveratrol using proliposomal formulation: investigation of various factors contributing to prolonged absorption of unmetabolized resveratrol. Expert Opin Drug Deliv 11:493–503
- Bshara H, Osman R, Mansour S, El-Shamy Ael H. (2014). Chitosan and cyclodextrin in intranasal microemulsion for improved brain buspirone hydrochloride pharmacokinetics in rats. Carbohydr Polym 99:297–305
- Caddeo C, Manconi M, Fadda AM, et al. (2013). Nanocarriers for antioxidant resveratrol: formulation approach, vesicle self-assembly and stability evaluation. Colloids Surf B Bionterfaces 111:327–32
- Caddeo C, Teskac K, Sinico C, Kristl J. (2008). Effect of resveratrol incorporated in liposomes on proliferation and UV-B protection of cells. Int J Pharm 363:183–91
- Chen X, Zhi F, Jia X, et al. (2013). Enhanced brain targeting of curcumin by intranasal administration of a thermosensitive poloxamer hydrogel. J Pharm Pharmacol 65:807–16
- Coimbra M, Isacchi B, van Bloois L, et al. (2011). Improving solubility and chemical stability of natural compounds for medicinal use by incorporation into liposomes. Int J Pharm 416:433–42
- Coradini K, Lima FO, Oliveira CM, et al. (2014). Co-encapsulation of resveratrol and curcumin in lipid-core nanocapsules improves their in vitro antioxidant effects. Eur J Pharm Biopharm 88:178–85
- Davidov-Pardo G, McClements DJ. (2015). Nutraceutical delivery systems: resveratrol encapsulation in grape seed oil nanoemulsions formed by spontaneous emulsification. Food Chem 167:205–12
- Donsi F, Sessa M, Mediouni H, et al. (2011). Encapsulation of bioactive compounds in nanoemulsion based delivery systems. Procedia Food Sci 1:1666–71
- Frozza RL, Salbego C, Bernardi A, et al. (2011). Incorporation of resveratrol into lipid-core nanocapsules improves its cerebral bioavailability and reduces the Aβ-induced toxicity. Alzheimers Dement 7:S114
- Gabal YM, Kamel AO, Sammour OA, Elshafeey AH. (2014). Effect of surface charge on the brain delivery of nanostructured lipid carriers in situ gels via the nasal route. Int J Pharm 473:442–57
- Gregori M, Masserini M, Mancini S. (2015). Nanomedicine for the treatment of Alzheimer's disease. Nanomedicine (Lond) 10:1203–18
- Holland ML, Panetta JA, Hoskins JM, et al. (2006). The effects of cannabinoids on P-glycoprotein transport and expression in multidrug resistant cells. Biochem Pharmacol 71:1146–54
- Horvát S, Fehér A, Wolburg H, et al. (2009). Sodium hylauronate as a mucoadhesive component in nasal formulation enhances delivery of molecules to brain tissue. Eur J Pharm Biopharm 72:252–9
- Ibrahim HK, El-Leithy IS, Makky AA. (2010). Mucoahesive nanoparticles as carrier systems for prolonged ocular delivery of gatifloxacin/prednisolone biotherapy. Mol Pharm 7:576–85
- Kumar A, Naidu PS, Seghal N, Padi SS. (2007). Neuroprotective effects of resveratrol against intracerebroventricular colchicine-induced cognitive impairment and oxidative stress in rats. Pharmacology 79:17–26
- Kumar M, Misra A, Babbar AK, et al. (2008). Intranasal nanoemulsion based brain targeting drug delivery system of risperidone. Int J Pharm 358:285–91
- Kusuhara H, Furuie H, Inano A, et al. (2012). Pharmacokinetic interaction study of sulphasalazine in healthy subjects and the impact of curcumin as an in vivo inhibitor of BCRP. Br J Pharmacol 166:1793–803
- Loscher W, Potschka H. (2005). Blood-brain barrier active efflux transporters: ATP-binding cassette gene family. NeuroRx 2:86–98
- Lovelyn C, Attama A. (2011). Current state of nanoemulsions in drug delivery. J Biomater Nanobiotechnol 2:626–39
- Lucas-Abellán C, Fortea I, López-Nicolás JM, Nunez-Delicado E. (2007). Cyclodextrins as resveratrol carrier system. Food Chem 104:39–44
- Ma T, Tan MS, Yu JT, Tan L. (2014). Resveratrol as a therapeutic agent for Alzheimer's disease. Biomed Res Int 2014:350516
- Mahajan HS, Dinger SB. (2011). Design and in vitro evaluation of nanoemulsion for nasal delivery of artemether. IJNDD 3:272–7
- Monroy A, Lithgow GJ, Alavez S. (2013). Curcumin and neurodegenerative diseases. Biofactors 39:122–32
- Moorthi C, Senthil Kumar C, Mohan S, et al. (2013). Application of validated RP-HPLC-PDA method for the simultaneous estimation of curcumin and piperine in Eudragit E 100 nanoparticles. J Pharm Res 7:224–9
- Nabekura T. (2010). Overcoming multidrug resistance in human cancer cells by natural compounds. Toxins (Basel) 2:1207–24
- Nasr M, Awad GA, Mansour S, et al. (2011). Different modalities of NaCl osmogen in biodegradable microspheres for bone deposition of risedronate sodium by alveolar targeting. Eur J Pharm Biopharm 79:601–11
- Nasr M, Mansour S, Mortada ND, El Shamy AA. (2008a). Lipospheres as carriers for topical delivery of aceclofenac: preparation, characterization and in vivo evaluation. AAPS Pharm Sci Tech 9:154–62
- Nasr M, Mansour S, Mortada ND, Elshamy AA. (2008b). Vesicular aceclofenac systems: a comparative study between liposomes and niosomes. J Microencapsul 25:499–512
- Nasr M, Nawaz S, Elhissi A. (2012). Amphotericin B lipid nanoemulsion aerosols for targeting peripheral respiratory airways via nebulization. Int J Pharm 436:611–16
- Nasr M, Taha I, Hathout RM. (2013). Suitability of liposomal carriers for systemic delivery of risedronate using the pulmonary route. Drug Deliv 20:311–18
- Rachmawati H, Budiputra DK, Mauludin R. (2015). Curcumin nanoemulsion for transdermal application: formulation and evaluation. Drug Dev Ind Pharm 41:560–6
- Sahni JK, Doqqui S, Ali J, et al. (2011). Neurotherapeutic applications of nanoparticles in Alzheimer's disease. J Control Release 152:208–31
- Scheepens A, Tan K, Paxton JW. (2010). Improving the oral bioavailability of beneficial polyphenols through designed synergies. Genes Nutr 5:75–87
- Sessa M, Balestrieri ML, Ferrari G, et al. (2014). Bioavailability of encapsulated resveratrol into nanoemulsion-based delivery systems. Food Chem 147:42–50
- Sessa M, Tsao R, Liu R, et al. (2011). Evaluation of the stability and antioxidant activity of nanoencapsulated resveratrol during in vitro digestion. J Agric Food Chem 59:12352–60
- Sharma M, Gupta YK. (2002). Chronic treatment with trans resveratrol prevents intracerebroventricular streptozotocin induced cognitive impairment and oxidative stress in rats. Life Sci 71:2489–98
- Shen H, Shi S, Zhang Z, et al. (2015). Coating solid lipid nanoparticles with hyaluronic acid enhances antitumor activity against melanoma stem-like cells. Theranostics 5:755–71
- Sood S, Jain K, Gowthamarajan K. (2013). Intranasal delivery of curcumin-/INS; donepezil nanoemulsion for brain targeting in Alzheimer's disease. J Neurol Sci 333:e316–17
- Sood S, Jain K, Gowthamarajan K. (2014). Optimization of curcumin nanoemulsion for intranasal delivery using design of experiment and its toxicity assessment. Colloids Surf B Biointerfaces 113:330–7
- Sun AY, Wang Q, Simonyi A, Sun GY. (2010). Resveratrol as a therapeutic agent for neurodegenerative diseases. Mol Neurobiol 41:375–83
- Tavano L, Muzzalupo R, Picci N, de Cindio B. (2014). Co-encapsulation of lipophilic antioxidants into niosomal carriers: percutaneous permeation studies for cosmeceutical applications. Colloids Surf B Biointerfaces 114:144–9
- Vanaja K, Wahl MA, Bukarica L, Heinle H. (2013). Liposomes as carriers of the lipid soluble antioxidant resveratrol: evaluation of amelioration of oxidative stress by additional antioxidant vitamin. Life Sci 93:917–23
- Yang F, Lim GP, Begum AN, et al. (2005). Curcumin inhibits formation of amyloid beta oligomers and fibrils, binds plaques and reduces amyloid in vivo. J Biol Chem 280:5892–901