Abstract
Chronic plaque psoriasis is an inflammatory skin disease affecting 2–3% of the world population. With increasing understanding of the progress of disease and its causes, bacterial infection is reported to be one of the potential reasons. In this regard, fusidic acid (FA), a steroidal antibiotic, has been a drug of choice which could play an important role by virtue of its unique mechanism of action. Despite many topical formulations of FA in practice, drug-delivery issues like permeability in the prevailing infectious conditions and stability of the drug are yet the challenges not been covered so far from the formulation development perspective. For these issues, liposomes, on account of their carrier-specific properties, have been suggested as delivery tools to fulfill the expectations. In the present work, FA liposomes (FA-LP) were prepared and characterized for its varied traits such as size (420–740 nm), surface charge, morphology, percent skin permeation (>75%), and retention (1.620 ± 0.8 mg/cm2). Confocal laser scanning microscope (CLSM) images revealed appreciable cell-uptake of fluorescent dye-loaded liposomes. In stability, FA-LP proved to be stable with respect to drug leakage and vesicle size. In vivo studies using the mouse tail model, FA-LP, are found significantly better (p < 0.05) vis-à-vis conventional one with improved efficacy in and around the target site by the carrier-effect. Hence, the work suggests for the possibility of a better FA liposome-based formulation as a potential option in addressing the infectious challenges of psoriasis.
Introduction
Psoriasis is a complex, recurrent, inflammatory skin disease affecting 2–3% of global population owing to its complicated etiology. The disease can affect almost every part of the human body, including scalp, knees, and elbow (Mahajan & Handa, Citation2013). Exact etiology of psoriasis is although not well understood, yet the disease is believed to be an autoimmune inflammatory disorder associated with genetics (Lowes et al., Citation2007).
Chronic plaque psoriasis, in this context, is one of the most common forms of psoriasis, characterized by redness, thickness, and scaling of the skin. Owing to reduced time-scale of skin cell maturation, hyper-proliferation of epidermal keratinocytes results in plaque psoriasis, leading eventually to symptoms like pain, irritation, inflammation, dryness, itching, burning, and silver-white scale formation on the skin (Katare et al., Citation2010).
Various risk factors influencing recurrence of psoriasis are genetic changes, infections, local trauma, duration of treatment, sunlight exposure, alcohol intake, smoking, endocrine factors and stress. Besides, the bacterial infections play an important role in the initiation and dissemination of psoriatic conditions in case of chronic plaque psoriasis (Owen et al., Citation2001). Therefore, the drugs with capability to restore normal keratinization with enucleated squames (i.e. orthokeratosis) and prevent hyperproliferation are rendered effective anti-psoriatic agents (Raza et al., Citation2011a; Bhatia et al., Citation2012). Heavy colonies of Staphylococcus strains have been found in skin samples of chronic plaque psoriatic patients. Existing facts revealed that bacteria, due to their super-antigens, induce immunological changes which release cytokines, subsequently resulting in psoriasis like condition (Marples et al., Citation1973).
Out of the various treatment options available, the topical treatment is generally regarded as the first-line therapy for the skin diseases (Menter et al., Citation2009; Mason et al., Citation2013). Not only anti-psoriatic agents are being employed as drugs to manage the psoriatic conditions, but antibiotics are also supplemented to tackle the bacterial cause of disease propagation (Owen et al., Citation2001). Out of various antibiotics, imidazole family, sulfacetamide, and sulfur have been frequently employed for the purpose.
Fusidic acid (FA) is a steroidal bacteriostatic agent, derived from the fungus, Fusidium coccineum, in the year 1962 (Godtfredsen et al., Citation1962; Turnidge, Citation1999). It is prescribed for the management of skin, ocular, and orthopedic infections (Skov et al., Citation2001; Falck et al., Citation2006; Chhibber et al., Citation2015). The antipsoriatic potential of FA was explored in 1970 during the preliminary level clinical trials (Kurwa & Abdel-Aziz, Citation1973; Nyfors, Citation1973). However, several reviews (Hall-Smith, Citation1971; Jackson, Citation1971; Levantine, Citation1971; Voetmann, Citation1971; Forstrom & Lassus, Citation1972; Mackey Citation1974; Christiansen, Citation1999) are available for the use of FA in psoriasis but till date there was lack of preclinical evidences for the same. Possible mechanism for this action is not well known but may be due to other reported activities of FA (Bendtzen et al., Citation1990; Christiansen, Citation1999).
Presently, FA is available as oral, parenteral, and topical formulations for the treatment of various infections. However, oral and parenteral routes have been reported to be linked with several health risks including GIT discomfort, liver upset, diarrhea, hepatotoxicity, phlebitis, and rhabdomyolysis. These adverse effects are ascribable to the wide systemic distribution and absorption of FA to different body organs, which potentially decreases its therapeutic efficacy at the target site, i.e. skin (Fuller, Citation2009; Lipsky & Hoey, Citation2009). The topical drug delivery also poses drug-delivery-related obstacles such as local irritation, poor dermal penetration, and sub-optimal efficacy (Fisher, Citation1996; Levy & Marshall, Citation2004; Katare et al., Citation2010; Vanangamudi et al., Citation2011). The key challenge in this context is the tough keratinized upper layer of skin and the absence of normal moisturizing factors such as water, which makes the delivery of drugs extremely difficult.
Among the numerous forms of drug delivery vehicles, vesicular systems like liposomes have emerged as potential and promising carrier for effective drug delivery in the past few decades (Agarwal et al., Citation2002; Cortesi et al., Citation2004; Saraswat et al., Citation2007; Agarwal et al., Citation2011; Hallaj-Nezhadi & Hassan, Citation2015; Rahman et al., Citation2015). These are spherical vesicles consisting of phospholipid bilayers surrounding an aqueous core while cholesterol is present within their lipid bilayers (Valenta et al., Citation2000; Touitou, Citation2002; Drulis-Kawa & Dorotkiewicz-Jach, Citation2010). The topical administration of FA, in vesicular system, accordingly, is considered as one of the most promising approaches (Nicolosia et al., Citation2015) which resolves the delivery issues and leads to improved targeting at the psoriatic lesions. Therefore, it was envisioned to encapsulate FA in a biocompatible vesicular system in an endeavor towards safe and better delivery to manage the psoriatic conditions.
Materials
Fusidic acid (FA), Carbopol® 980, and phospholipids (Phosphatidylcholine 90%) were obtained ex-gratis from M/s Glenmark Private Limited, Baddi, India; M/s Lubrizol Advanced Materials India Private Limited, Mumbai, India, and M/s Phospholipid GmbH, Nattermannallee, Germany, respectively. All other analytical grade chemicals were used as procured. Ultrapure water (Milli-Q® Integral system; M/s Merck Millipore, Billerica, MA) was used all over the protocols. Fucidin™ cream (FA Commercial Cream) containing FA (2% w/w); Batch No. G24313, Mfg. Lic. No. 313, Mfg. Date June, 2013; M/s Ranbaxy Labs Ltd., Goa, India) was procured from local drug store.
Methods
Preparation of FA liposomes
FA-loaded liposomes were prepared by thin-film hydration technique, as per the procedure described previously (Raza et al., Citation2011b). Accurately weighed amounts of drug (FA), phospholipid (PL), cholesterol (CH), and/or Span 80 (SP80) were dissolved in a solvent mixture (chloroform:methanol, 2:1 v/v) in 250 ml round-bottom flask (nitrogen flushed). The amount of drug (2% w/w) and mass ratio of FA:PL (1:1.5) was kept constant while different PL:CH (7:3, 8:2, and 9:1) and PL:CH:SP80 (7:3:1, 8:2:1, 9:1:1, 7:3:2, 8:2:2, 9:1:2, a total mass of 100 mg) ratios were prepared. A thin layer of lipid film was formed on the wall of round-bottom flask by evaporating the organic solvent using a rotary film evaporator (Buchi RE 121 (Rotovap), Flawil, Switzerland) under reduced pressure. During the preparation process, the instrumental conditions such as temperature (55 °C) and rotation speed (100 rpm) were maintained. The flask was kept under vacuum in desiccator to ensure complete removal of residual solvents. The obtained thin lipid film formed on the wall of the flask was then hydrated using phosphate buffer saline pH 7.4 in (10% v/v) ethanolic solution at 55 ± 2 °C. The resulting vesicle dispersion was vortexed for 5 min and then left undisturbed for 2–3 h for complete process of hydration. The developed formulations were characterized with respect to carrier-specific parameters.
Incorporation of nano-carrier system in a secondary vehicle
In order to make the formulations rheologically acceptable for topical application, the selected vesicular dispersion was further incorporated in neutralized Carbopol 980 hydrogel (1% w/w).
Animals and maintenance
Healthy Swiss albino LACA mice (female), aged between 4 and 6 weeks and weighing between 20 and 25 g, were acquired from Central Animal House of Panjab University, Chandigarh, India. The procured animals were housed in polypropylene cages. Prior to the treatments, these were acclimatized for a week with 12 h light-and-dark cycle at optimum temperature of 27 ± 3 °C and relative humidity of 52 ± 3%. The mice were provided with standard rodent diet and drinking water ad libitum. Taking cognizance that the research protocol adheres to the guidelines for care and use of the laboratory animals, all the animal studies were performed as per the requisite procedure approved by the Panjab University Animal Ethics Committee duly approved for the purpose of control and supervision of experiments on animals by the Government of India (Ref. letter no. IAEC/156).
Characterization and evaluation
Micromeritics and surface charge
Sample was placed in the cuvette and particle size of the prepared vesicles was determined using dynamic light scattering (DLS) technique (Zetasizer 2000 HS, M/s Malvern Instruments Limited, Malvern, UK). DLS measures the diffusion of particles which are moving under Brownian motion, and converts into size and its distribution by the Stokes–Einstein relationship. As zeta potential holds promise to predict the in vivo stability of the liposomes, the same instrument was employed to determine the zeta potential of liposomal formulations too.
Morphology
The prepared vesicles were examined with different microscopical techniques, i.e., optical microscopy (OM), transmission electron microscopy (TEM), and confocal laser scanning microscopy (CLSM) and were compared in order to highlight advantages of these techniques as below:
Optical microscopy
The prepared vesicles were characterized for surface morphological examination employing phase contrast optical microscope (Medilux, Kyowa Opticals Co. Ltd., Hashimoto, Japan) at suitable magnification.
Transmission electron microscopy
A drop of liposomal suspension was placed and adsorbed on microscopic carbon-coated grids. These grids were subsequently negatively stained with 1% (w/v) aqueous solution of phosphotungstic acid, dried, and viewed under TEM at suitable magnifications, operating at an acceleration voltage of 200 kV.
Confocal microscopy
Coumarin-6 (0.015 mg/ml) loaded liposomes were prepared (as per “Preparation of FA liposomes” section) and analyzed using confocal microscopy (Olympus FV10i, M/s Olympus Singapore Pte Ltd., Singapore, Singapore). Unentrapped dye was removed from the formulation using dialysis bags (M/s Himedia, Mumbai, India; 12 kDa), as per previously reported procedure (Ruozi et al., Citation2011; Raza et al., Citation2013a).
Entrapment efficiency
The entrapment efficiency of liposomes was determined by mini-column centrifugation technique (Raza et al., Citation2011a). Sephadex G 50 columns were prepared and pre-saturated with blank vesicular carriers. After complete saturation of these columns, FA-loaded vesicular dispersion (0.2 ml) was then placed in the column. Centrifugation was done at speed of 3000 rpm for 3–4 min in order to separate drug-entrapped liposomes. The column was rinsed using water and was pooled with the previously collected elute. Thereafter, the eluted dispersion was appropriately digested with chloroform–methanol (2:1 v/v) solvent mixture (Raza et al., Citation2011b, Citation2013b; Aisha et al., Citation2014) and analyzed for drug content using reverse phase high-performance liquid chromatography (RP-HPLC) (Chhibber et al., Citation2015). The chromatographic separation was achieved using methanol:phosphoric acid (10 g/l): water:acetonitrile (10:20:20:50 v/v) as the mobile phase with a flow rate at 2 ml/min and column oven temperature at 30 °C. The runtime for each sample was fixed at 25 min and the peaks were monitored with UV detection at 235 nm (European Pharmacopoeia, Citation2008).
Rheological and texture analysis
The rheology studies on the developed hydrogels and commercial cream were carried out by cup and bob rheometer (Rheometer HS-143; M/s Rheolab QC, Anton Paar, Germany). The tested samples (approximately 5 g) were placed in a concentric cylinder arrangement. The shear stress value was increased, automatically and linearly from 0.1 to 100 s−1. The texture analysis was performed using TA-XT.2 Texture AnalyzerTM (M/s Stable Microsystems, Godalming, UK) with specific instrument settings. Before testing, the upper cone probe was calibrated against the lower cone to adjust the height for each test, i.e. 25 mm above the lower cone. During testing, sample (5 g) was placed into the lower cone and then upper cone probe approached and penetrated into the sample to the depth of 2 mm above the surface of sample holder, i.e. the probe moved to a distance of 23 mm from its starting point at a test speed of 3 mm/s. Various texture parameters along with force of penetration and detachment of probe were measured (Bhatia et al., Citation2009).
Skin permeation and retention studies
The skin permeation studies were performed on Franz diffusion cells (M/s Permegear, Inc., Hellertown, PA) using shaved skin of Laca mice as the permeation barrier. Harvested mouse skin was freed of adhering fat layers and mounted on diffusion cells (a cross-sectional area of 3.142 cm2 and a receptor volume was about 30.0 ml). Diffusion medium placed in the receptor compartment was composed of 10% ethanolic phosphate buffer solution of pH 7.4. Diffusion cell was maintained at temperature of 32 ± 1 °C with the help of thermo-regulated outer water jacket, while the placed medium was continuously stirred. Each formulations (the developed formulation, conventional hydrogel and commercial cream), containing equivalent amount of FA (2% w/w), were applied onto the mice skin in the donor compartment. Aliquots of 2 ml each were periodically withdrawn at suitable time intervals (0, 0.25, 0.5, 0.75, 1, 2, 4, 6, 8, 12, and 24 h) from the sampling port and replaced with an equal volume of fresh diffusion medium to maintain the constant receptor volume (Raza et al., Citation2011b). The collected samples were analyzed using RP-HPLC.
After completion of permeation studies, the processing for the skin retention was performed. In brief, skin mounted on the diffusion cell assembly was carefully removed and washed thrice with ultrapure water and dried on lint-free cotton swab. The skin was then chopped into small pieces and macerated in methanol (5 ml) for 24 h for the complete drug extraction. After filtering the solution through a membrane filter (0.45 µm), the filtrate was analyzed using RP-HPLC.
Cell uptake efficiency of delivery systems
For the evaluation of cell uptake efficiency, CLSM technique is reported to facilitate better visualization of drug transport process in different parts of the skin. Fluorescent marker (Coumarin-6)-labeled vesicular gel was prepared as per “Preparation of FA liposomes” and “Confocal microscopy” sections, and compared with dye-loaded plain hydrogel. Slides of mice skin treated with fluorescence-labeled formulations for 6 h were fixed and observed for the presence of fluorescence dye in the various skin layers with the help of confocal laser scanning microscope (Olympus FV10i, M/s Olympus Singapore Pte Ltd., Singapore, Singapore). The slides were placed in argon laser beam and images were captured at optical excitations wavelength of 488 nm.
Evaluation of antipsoriatic activity – mouse tail model
The selected formulations were screened for their possible antipsoriatic activity using mouse tail model (Bosman et al., Citation1992; Vogel & Vogel, Citation2002). The normally prevailing conditions in mouse tail serve as one of the frequently used testing models for screening of drugs for the treatment of psoriasis (Bladon et al., Citation1985).
Treatment groups
Following 1 week of acclimatization, mice were divided into four groups, six mice in each group, as follows:
Group 1: Control (Normal saline)
Group 2: Treated with FA (2% w/w) commercial cream
Group 3: Treated with FA (2% w/w) liposomal gel
Group 4: Treated with FA (2% w/w) hydrogel
In Groups 2, 3, and 4, equivalent amount of formulations (2% w/w of fusidic acid) was applied topically on the proximal half of the mouse tail once daily for five times a week for 2 weeks. Histopathological features was examined for n = 6 animals per treatment group, n = 6 sections per animal, and n = 3 dimensions per section. Twenty-four hours after the last application of formulation(s), the animals were sacrificed by spinal dislocation, and the tail skin sections were cut by longitudinal dissection with a scalpel and fixed in formalin solution for histological examination (Bhatia et al., Citation2009, Citation2012).The obtained skin samples were appropriately processed and stained with hematoxylin–eosin (H&E) dye. The processed skin samples were examined microscopically for the presence of granular layer in the scale regions, epidermal thickness, elongation of rete-ridges, and capillary loop dilation. Induction of orthokeratosis in those parts of the adult mouse tail, having normal parakeratotic differentiation, was quantified by measuring the length of the granular layer (A) and the length of the scale (B) (Bhatia et al., Citation2014). Ten sequential scales were examined for each skin section. Percent orthokeratosis (% OK) was calculated by Equation (Equation1(1) ) and drug activity (DA) is defined by an increase in the percentage of orthokeratotic regions per scale and was calculated using Equation (Equation2
(2) ), as follows:
(1)
(2)
Stability studies: drug leakage and integrity of vesicles
The tendency of drug leakage, particle size, and extent of structural integrity of selected formulation was observed for a period of 3 months by storing the sealed formulation in glass vials (n = 3) at different temperatures, i.e. 5 ± 2 °C, 30 ± 2 °C/65% ± 5% RH, and 40 ± 2◦C/75% ± 5% RH. Stored formulation was periodically estimated at regular intervals and physical changes in the formulation were monitored by microscopic and visual observations (Raza et al., Citation2011a).
Results and discussion
Micromeritics and surface charge
The average particle size of FA-loaded liposomes () was found to be in range between 420 nm and 740 nm, indicating nanometric size range with acceptable polydispersity index of less than 0.4. Among all the prepared formulations, FA-LP5 was selected as the optimized formulation (Verma et al., Citation2003) primarily owing to narrow size range (). The smaller size of FALP5 formulation as compared with other ratios may possibly be attributed to the difference in the composition of liposomes. Evaluation of zeta-potential revealed the neutral values (), apparently ascribed to the presence of neutral phospholipid (Raza et al., Citation2011b).
Figure 1. (a) Particle size distribution curve of the selected liposomal dispersion and (b) zeta potential measurement observed during analysis.
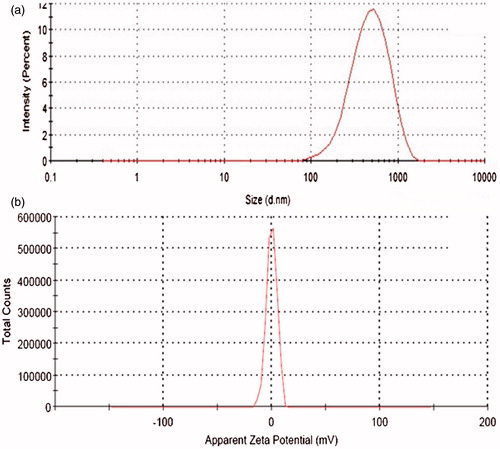
Table 1. Composition and different parameters of liposome formulation.
Morphology
Optical photomicrographs in depict uniform morphology and low degree of aggregation while the TEM images () reveal negatively stained photomicrographs of vesicles. The CLSM images () demonstrated optimum dye retention by lipid-rich vesicles as well as their spherical shape. The optical photomicrograph of liposomal hydrogel () reveals that the structural integrity of the liposomes is well maintained, when dispersed in a secondary vehicle. It was observed that Carbopol hydrogels remain stable when liposomes were incorporated, this might be due to the accommodation of liposomes within the three-dimensional network of the hydrogels (Gabrijelcic & Sentjurc, Citation1995).
Entrapment efficiency
Entrapment efficiency (%) of different formulations was found to range between 52.1 ± 2.3% and 72.60 ± 1.4% (). Despite the lipophilic nature of FA (log P = 4.42), the developed systems were able to offer > 55% of drug entrapment with the highest value observed for FA-LP5. This could be ascribed to the presence of optimum concentration of surfactant, which eventually increases the solubility of drug in the lipid layers. Whereas further increase in the surfactant concentration leads to aggregation, which lowered the values of entrapment efficiency and results in large particle size of the liposomes (Raza et al., Citation2011b).
Rheological and texture analysis
Various rheological attributes of the prepared gel(s) and commercial cream have been portrayed in . The developed lipogel of selected formulation was found to be non-Newtonian in behavior with significant magnitude of yield value (125.6), which may have contributed to the rigid structure of the hydrogel (Raza et al., Citation2013a). As per the Herschel–Bulkley model, the values of “n” were found to be markedly less than unity in case of liposomal gel (0.3292), plain hydrogel (0.4191), and commercial cream (0.1642), thus indicating their shear-thinning nature (Basu et al., Citation2007).
Figure 3. Rheological profile of (a) selected FA liposome gel, (b) FA commercial cream, and (c) FA hydrogel and texture analysis of (d) selected FA liposome gel, (e) FA commercial cream, and (f) FA hydrogel.
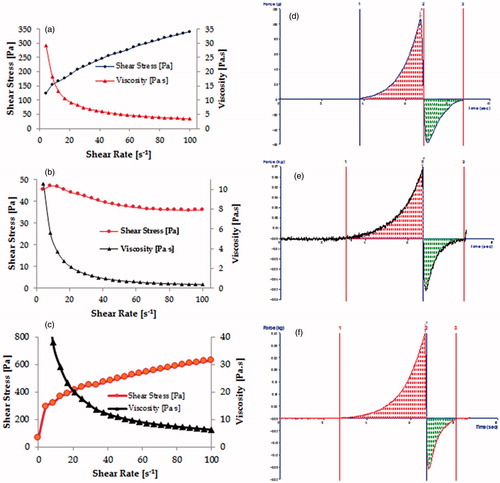
Texture analysis of liposomal gel, commercial cream, and plain hydrogel, depicted in , shows the variation of force as a function of time, to reveal the gel strength, its extrusion force, stickiness, and work of spreading. The results of texture analysis confirmed that the developed gel exhibited fairly good gel strength, ease of spreading, and optimum extrusion from the tube as compared with commercial cream. Further, uniformity of curve, plotted using the Exponent 32® software (Hunter Associates Inc., Reston, VA), confirmed the smoothness and lack of any grittiness in the FA-liposomal gel, a highly desirable characteristic for any topical preparation (Bhatia et al., Citation2009).
Skin permeation and retention
Permeation profiles of FA liposomal gel (selected formulation), commercial cream, and plain hydrogel employing mice skin are shown in . Drug permeation (%) in 24 h was found to be around 75 ± 1.2%, 53 ± 2.5%, and 59 ± 1.7% in the case of liposomal gel, commercial cream, and plain hydrogel, respectively. While in skin retention studies, notably higher values of drug retention were obtained from liposomal gel (1.620 ± 0.8 mg/cm2) vis-à-vis the commercial cream (0.476 ± 1.4 mg/cm2) and plain hydrogel (0.659 ± 1.6 mg/cm2). The immense level of permeation with the liposomal gel vis-à-vis conventional systems is attributed to the alteration in the physico-chemical environment built up by the phospholipid constituted vehicle. That it altered skin layers to make the drug better accessible and hence transfer across the barrier while in case of conventional systems this type of interaction has been considered to be unavailable (Katare et al., Citation2010). Further, the higher retention within the skin could be affected by virtue of the reservoir effect which is expected for the reason that lipid-based systems do possess the ability to integrate with the skin cells and more compatible with the physiology (Mitchell & Winham, Citation1981; Raza et al., Citation2011b). Thus, all the factors plead for the better availability of the drug that too in a conducive climate, towards the target receptors, of the prevailing psoriatic disease.
Cell uptake efficiency of delivery systems
Confocal laser scanning microscope images () showed penetration of coumarin-6 dye-loaded liposomal gel and plain hydrogel in various skin layers, following 6 h of topical application. The circular fluorescent nano-structures in skin layers ratified the transport of dye-entrapped liposomal systems () in the epidermis locale with wide distribution in the deeper skin layers as compared with the plain hydrogel (), which remained confined to the upper layer of the skin only. These favorable outcomes are by virtue of carrier effect and are in agreement with the findings of skin permeation and retention studies (Dragicevic-Curic et al., Citation2009).
Anti-psoriatic activity
Mouse tail epidermis keratinization mimics human keratinization in psoriasis condition, therefore, it helps in more accurate estimation of both test and control groups (Hofbauer et al., Citation1988; Sebok et al., Citation2000). indicates the histological features of H & E-stained mouse tail skin sections. On one hand, group 1 (control) shows distinct signs of parakeratotosis in , as high density of nucleated cells, elongation of rete ridges with no evidence of granular layer was observed. On the other hand, group 2 (), group 3 (), and group 4 (), demonstrated the absence of rete ridges, a decline in the population of nucleated cells and the presence of granular layer. Two weeks treatment with selected FA liposomal formulation () resulted in marked histological changes in tail skin section as compared with conventional formulations, as the regions of orthokeratotic stratum corneum extended longitudinally into the regions of previously present parakeratotic condition. All the groups exhibited different scores of orthokeratosis (OK) as well as drug activity (DA). It can be observed that control group 1 exhibited OK to the order of 15.5 ± 0.54%, while groups 2, 3, and 4 had OK (%) and DA (%) to the extent of (30.6 ± 0.75% and 17.8 ± 1.42%), (56.2 ± 1.20% and 48.1 ± 1.27%), and (39.8 ± 1.81% and 28.7 ± 1.30%), respectively, as depicted in . The results revealed that FA liposomal formulation possessed significantly enhanced (p < 0.05) anti-psoriatic activity as compared with the control group and conventional formulations. These indicated that liposomes exhibited enhanced penetration and permeation behavior as compared with conventional ones (Raza et al., Citation2011a). This phenomenon was confirmed by skin permeation and retention studies as well as by CLSM observation in cell-uptake studies. The results of the current studies, therefore, are in consonance with the previous findings on antipsoriatic activity (Kurwa Citation1973; Raza et al., Citation2011a; Dhanabal et al., Citation2012) and liposomes as a drug delivery tool (Mezei & Gulasekharam Citation1980; Katare et al., Citation2001).
Stability studies
The stability data of 3 months study construed that drug assay was found in range between 95.14% and 101.21%, well within the pharmacopoeial limits, when stored at ambient and low temperature (USP 32-NF 27 2009) than at high temperature (11.5% loss). There was an increase in the particle size when stored at high (945.1 nm) and ambient temperature (685.7 nm) whereas this change was less when stored at low temperature (474.4 nm) from initial particle size (420.6 nm). Optical microscopic studies revealed that structural integrity was much better maintained at low and ambient temperatures vis-à-vis the high temperatures, where slight aggregation and vesicle disruption like problems were apparent. Hence, to circumvent the drug loss and to maintain the size and structure of nano-constructs, the developed formulation should be stored at low temperature conditions.
Conclusions
The outcome of the present studies indicate the enhanced potential in fusidic acid, one of the most frequently used antibiotics, when an appropriate formulation strategy using liposomal system is affected. The comparative studies carried out with respect to conventional system highlights the difference on all levels of performance. The enhanced efficacy, safety, and stability of the FA-liposomal system can be correlated to the aspects of composition, constituents, and process conditions which could play a pivotal role in altering the ambience surrounding drug molecule. This reflects on the physico-chemical behavior of drug per se, favorable changes in the barrier properties of the skin, the facilitated delivery, and localization of the molecule in the vicinity of the target-receptors present on infective organisms. And also, it is the spiked interaction of drug in liposomal form with the receptor which could mediate improved pharmacodynamic response. As far stability is concerned, the phospholipids being antioxidant in character held accountable for building the protective interior so as to preserve the molecule for the betterment of the shelf-life. Thus, it may well be concluded that the adopted approach in the work is worth fetching the interest of the researchers. It will not only transform the product quality of the fusidic acid but also help contemplating applications based on the current approach for other such “difficult-to-formulate” molecules.
Acknowledgements
The authors are thankful to M/s Glenmark Pvt. Ltd, Baddi (India), M/s Phospholipid GmbH, (Germany) and M/s Lubrizol Advanced Materials India Pvt. Ltd. for generous gift samples of Fusidic acid, Phospholipids and Carbopol®. The support of Sophisticated Analytical Instrument Facility, Punjab University, Chandigarh for providing TEM facility and IMTECH, Chandigarh, for size and zeta potential measurement facility is gratefully acknowledged.
Declaration of interest
The authors report that they have no conflicts of interest. Financial grant obtained from Indian Council of Medical Research (ICMR), New Delhi, India.
References
- Agarwal R, Katare OP, Vyas SP. (2011). Preparation and in vitro evaluation of liposomal/niosomal delivery systems for antipsoriatic drug dithranol. Int J Pharm 228:43–52
- Agarwal R, Saraswat A, Kaur I, et al. (2002). A novel liposomal formulation of dithranol for psoriasis: preliminary results. J Dermatol 29:529–32
- Aisha AFA, Majid AMSA, Ismail Z. (2014). Preparation and characterization of nano liposomes of Orthosiphon stamineus ethanolic extract in soybean phospholipids. BMC Biotechnol 14:23
- Basu S, Shivhare US, Raghavan GSV. (2007). Time dependent rheological characteristics of pineapple jam. Int J Food Eng 3:1–10
- Bendtzen K, Diamant M, Faber V. (1990). Fusidic acid, an immunosuppressive drug with functions similar to cyclosporin A. Cytokine 2:423–9
- Bhatia A, Raza K, Singh B, Katare OP. (2009). Phospholipid-based formulation with improved attributes of coal tar. J Cosmet Dermatol 8:282–8
- Bhatia A, Singh B, Raza K, et al. (2012). Tamoxifen-loaded novel liposomal formulations: evaluation of anticancer activity on DMBA-TPA induced mouse skin carcinogenesis. J Drug Target 20:544–50
- Bhatia A, Singh B, Wadhwa S, et al. (2014). Novel phospholipid-based topical formulations of tamoxifen: evaluation for antipsoriatic activity using mouse-tail model. Pharm Dev Technol 19:160–3
- Bladon PT, Taylor M, Wood EJ, Cunliffe WJ. (1985). Effect of crude coal tar in the mouse-tail model of psoriasis. Arch Dermatol Res 277:121–5
- Bosman B, Matthiesen T, Hess V, Friderichs E. (1992). A quantitative method for measuring antipsoriatic activity of drugs by the mouse tail test. Skin Pharmacol 5:41–8
- Chhibber T, Wadhwa S, Chadha P, et al. (2015). Phospholipid structured microemulsion as effective carrier system with potential in methicillin sensitive Staphylococcus aureus (MSSA) involved burn wound infection. J Drug Target 23:943–52
- Christiansen K. (1999). Fusidic acid non-antibacterial activity. Int J Antimicrob Agent 12:S73–8
- Cortesi R, Romagnoli R, Menegatti E, et al. (2004). Liposomes containing distamycins: preparation, characterization and antiproliferative activity. Drug Del 11:83–8
- Dhanabal SP, Dwarampudi LP, Muruganantham N, Vadivelan R. (2012). Evaluation of the antipsoriatic activity of aloe vera leaf extract using a mouse tail model of psoriasis. Phytother Res 26:617–19
- Dragicevic-Curic N, Scheglmann D, Albrecht V, Fahr A. (2009). Development of different temoporfin-loaded invasomes-novel nanocarriers of temoporfin: characterization, stability and in vitro skin penetration studies. Colloids Surf B Biointerfaces 70:198–206
- Drulis-Kawa Z, Dorotkiewicz-Jach A. (2010). Liposomes as delivery systems for antibiotics. Int J Pharm 387:187–98
- European Pharmacopoeia. (2008). European Directorate for the quality of medicines. 6th ed. Strasbourg, France: Council of Europe, 1645
- Falck E, Hautala JT, Karttunen MP, et al. (2006). Interaction of fusidic acid with lipid membranes: implications to the mechanism of antibiotic activity. Biophys J 91:1787–99
- Fisher A. (1996). Lasers and allergic contact dermatitis to topical antibiotics, with particular reference to bacitracin. Cutis Oct 58:252–4
- Forstrom L, Lassus A. (1972). Fucidic acid and psoriasis. Ann Clin Res 6:165–6
- Fuller FW. (2009). The side effects of silver sulfadiazine. J Burn Care Res 30:464–70
- Gabrijelcic V, Sentjurc M. (1995). Influence of hydrogels on liposome stability and on the transport of liposome entrapped substance into the skin. Int J Pharm 118:207–12
- Godtfredsen WO, Jahnsen S, Lorck H, et al. (1962). Fusidic acid: a new antibiotic. Nature 193:987–8
- Hall-Smith P. (1971). Fusidic acid treatment in psoriasis. Lancet 2:544
- Hallaj-Nezhadi S, Hassan M. (2015). Nanoliposome-based antibacterial drug delivery. Drug Del 22:581–89
- Hofbauer M, Dowd PM, Atkinson J, et al. (1988). Evaluation of a therapeutic concentration of dithranol in the mouse-tail test. Br J Dermatol 118:85–9
- Jackson N. (1971). Fusidic acid treatment in psoriasis. Lancet 2:712
- Katare OP, Raza K, Singh B, Dogra S. (2010). Novel drug delivery systems in topical treatment of psoriasis: rigors and vigors. Ind J Dermatol Venereol Leprol 76:612–21
- Kurwa A, Abdel-Aziz AH. (1973). Preliminary clinical trial of fusidic acid in psoriasis. Br J Clin Pract 27:92–4
- Levantine AV. (1971). Fusidic acid treatment in psoriasis. Lancet 2:661
- Levy SB, Marshall B. (2004). Antibacterial resistance worldwide: causes, challenges and responses. Nat Med 10:S122–9
- Lipsky BA, Hoey C. (2009). Topical antimicrobial therapy for treating chronic wounds. Clin Infect Dis 49:1541–9
- Lowes MA, Bowcock AM, Krueger JG. (2007). Pathogenesis and therapy of psoriasis. Nature 445:866–73
- Mackey JP. (1974). Fusidic acid in psoriasis (letter). Lancet 2:232–7
- Mahajan R, Handa S. (2013). Pathophysiology of psoriasis. Ind J Dermatol Venereol Leprol 79:1–9
- Marples RR, Heatom CL, Kligman AM. (1973). Staphylococcus aureus in psoriasis. Arch Dermatol 107:568–70
- Mason AR, Mason J, Cork M, et al. (2013). Topical treatments for chronic plaque psoriasis. Cochrane Database Syst Rev 3:CD005028
- Menter A, Korman NJ, Elmets CA, et al. (2009). Guidelines of care for the management of psoriasis and psoriatic arthritis. Section 3. Guidelines of care for the management and treatment of psoriasis with topical therapies. J Am Acad Dermatol 60:643–59
- Mezei M, Gulasekharam V. (1980). Liposomes-a selective drug delivery system for the topical route of administration. I. Lotion dosage form. Life Sci 26:1473–7
- Mitchell DJ, Winham BW. (1981). Micelles, vesicles and microemulsions. J Chem Soc Faraday Trans II 77:601–29
- Nicolosia D, Cuprib S, Genovesea C, et al. (2015). Nanotechnology approaches for antibacterial drug delivery: preparation and microbiological evaluation of fusogenic liposomes carrying fusidic acid. Int J Antimicrob Agents 45:622–6
- Nyfors A. (1973). Fucidin in psoriasis. A double-blind study of twenty psoriatics over two periods of four weeks each. Dermatologica 46:281–4
- Owen CM, Chalmers RJG, Osullivan T, Griffiths CE. (2001). A systematic review of antistreptococcal interventions for guttate and chronic plaque psoriasis. Br J Dermatol 145:886–90
- Rahman M, Akhter S, Ahmad J, et al. (2015). Nanomedicine based drug targeting for psoriasis: potentials and emerging trends in nanoscale pharmacotherapy. Expert Opin Drug Deliv 12:635–52
- Raza K, Katare OP, Setia A, et al. (2011a). Improved therapeutic performance of dithranol against psoriasis employing systematically optimized nanoemulsomes. J Microencapsul 30:225–36
- Raza K, Singh B, Lohan S, et al. (2013a). Nano-lipoidal carriers of tretinoin with enhanced percutaneous absorption, photostability, biocompatibility and anti-psoriatic activity. Int J Pharm 456:65–72
- Raza K, Singh B, Mahajan A, et al. (2011b). Design and evaluation of flexible membrane vesicles (FMVs) for enhanced topical delivery of capsaicin. J Drug Target 19:293–330
- Raza K, Singh B, Singal P, et al. (2013b). Systematically optimized biocompatible isotretinoin-loaded solid lipid nanoparticles (SLNs) for topical treatment of acne. Colloids Surf B: Biointerfaces 105:67–74
- Ruozi B, Belletti D, Tombesi A, et al. (2011). AFM, ESEM, TEM, and CLSM in liposomal characterization: a comparative study. Int J Nanomed 6:557–63
- Saraswat A, Agarwal R, Katare OP, et al. (2007). A randomized, double-blind, vehicle-controlled study of a novel liposomal dithranol formulation in psoriasis. J Dermatol Treat 18:40–5
- Sebok B, Bonnekon B, Kerenyi M, Gollnick H. (2000). Tazarotene induces epidermal cell differentiation in the mouse tail test used as an animal model for psoriasis. Skin Pharmacol Appl Skin Physiol 13:285–91
- Skov R, Frimodt-Moller N, Espersen F. (2001). Correlation of MIC methods and tentative interpretive criteria for disk diffusion susceptibility testing using NCCLS methodology for fusidic acid. Diagnos Microb Infect Dis 20:111–16
- Touitou E. (2002). Drug delivery across the skin. Expert Opin Biol Ther 2:723–33
- Turnidge J. (1999). Fusidic acid pharmacology, pharmacokinetics and pharmacodynamics. Int J Antimicrob Agents 12:S23–34
- US Pharmacopoeial Convention, USP 32-NF 27. (2009). Pharmaceutical stability, 2009;1150:662–3
- Valenta C, Wanka M, Heidlas J. (2000). Evaluation of novel soya-lecithin formulations for dermal use containing ketoprofen as a model drug. J Control Release 63:165–73
- Vanangamudi SS, Srinivasan M, Chulliel NN, et al. Novel dermaceutical cream made using sodium fusidate. Pub. no. US 2011/0257144 A1
- Verma DD, Verma S, Blume G, Fahr A. (2003). Particle size of liposomes influences dermal delivery of substances into skin. Int J Pharm 258:141–51
- Voetmann E. (1971). Fusidic acid treatment in psoriasis. Lancet 2:435
- Vogel GH, Vogel WH. (2002). Drug discovery and evaluation: pharmacological assays. 2nd ed. Germany: Springer-Verlag, 1325