Abstract
Context: Overactive bladder (OAB) is a common problem and anticholinergic drugs are first-line therapy, but they have side effects.
Objective: Development of oxybutynin chloride (OC) proniosomal gels and analyses of its efficacy for OAB treatment.
Materials and methods: Phase separation coacervation was used to prepare proniosomal gels using various non-ionic surfactants, lipids, soy lecithin and isopropyl alcohol. Gels were characterized with regard to entrapment efficiency (EE), vesicle size, surface morphology (using environmental scanning electron microscopy [E-SEM]), stability, attenuated total reflectance Fourier transform infrared (ATR-FTIR) spectroscopy, in vitro skin permeation, in vivo animal studies and histopathology.
Results and discussion: EE was 87–92%, vesicle size was 0.38–5.0 μm, and morphology showed some loosened pores in proniosomes after hydration. ATR-FTIR spectroscopy showed no significant shifts in peaks corresponding to OC and excipients. Most formulations exhibited >50% permeation but the cholesterol-containing formulations P3 (Span 20:Span 60 [1:1]) and P4 [Tween 20:Tween 80 (1:1)] had the highest percent cumulative permeation. P3 and P4 also showed faster recovery of cholinergic effects on salivary glands than oral formulations. P3 and P4 had pronounced therapeutic effects in reduction of urinary frequency and demonstrated improvements in bladder morphology (highly regenerative surface of the transitional epithelium).
Conclusion: These results suggest that OC could be incorporated into proniosomal gels for transdermal delivery in the treatment of OAB.
Introduction
The main feature of overactive bladder (OAB) is urgency with or without urge incontinence, which is often accompanied by frequency and nocturia (Abrams et al., Citation2002). It is a common problem suffered particularly by women aged 40–60 years (Van Der Vaart et al., Citation2002). Commonly used anticholinergic drugs are first-line therapy. Oxybutynin, tolterodine, propiverine, solifenacin, darifenacin, trospium and fesoterodine are antimuscarinic agents approved for use in OAB treatment. Oxybutynin remains the mainstay of OAB treatment (Sussman, Citation2007). Oxybutynin can act on a wide range of M3 muscarinic receptors, but has the side effects of dry mouth, constipation, cognitive deficits and hallucinations (Salvatore et al., Citation2005). However, studies by Appell (Citation2002) showed that oxybutynin given via the oral route results in anticholinergic adverse effects due to the high concentration of N-desethyloxybutynin (N-DEO). In addition, Nitti et al. (Citation2006) proposed that the bioavailability of oxybutynin given via the oral route is only 6%, and that oxybutynin avoids first-pass metabolism and follows first-order kinetics, thereby resulting in an unstable concentration of the drug in plasma. Nevertheless, plasma levels of desethyloxybutynin (which is primarily responsible for the anticholinergic side effects) were 5–12 times greater than those of oxybutynin (Krause et al., Citation2013).
To meet the needs of tolerability and compliance, oxybutynin is available as a topical gel, a once-daily oral dose and an immediate-release capsule. Newer delivery systems maintain steady-state characteristics and avoid pre-systemic metabolism of oxybutynin (Kennelly, Citation2010). In a randomized placebo-controlled trial, Zobrist et al. (Citation2001) showed that daily incontinence episodes of OAB were reduced markedly by use of an oxybutynin transdermal patch (OXY-TDS). Nevertheless, some studies have revealed that OXY-TDS can cause application-site reactions such as erythema, pruritus and dermatitis (Dmochowski et al., Citation2003; Sand et al., Citation2007; Radomski & Barkin, Citation2012). US Food and Drug Administration approved an oxybutynin chloride (OC) topical gel in January 2009. This topical gel, which comprises a 10% w/w ethanolic gel of OC, is applied once daily to the abdomen, buttocks or thighs and causes skin irritation in ≈6% of patients (Kennelly, Citation2010). Proniosomes are versatile delivery systems due to their components of lecithin, non-ionic surfactants and cholesterol. Non-ionic surfactants form a layer that can bring the drug deep into the skin without causing skin irritation or side effects; and cholesterol gives high stability and permeability to proniosome vesicles (Rawat et al., Citation2011). Hence, proniosomes could be used for transdermal delivery.
We focused on the development of an OC proniosomal gel and assessed its therapeutic efficacy against OAB.
Material and methods
Oxybutynin chloride (OC; Pi Chemicals, Shanghai, China), Span® surfactants, Tween® surfactants, cholesterol, soy lecithin, glycerine, acetic acid, pilocarpine (Sigma–Aldrich, Saint Louis, MO), glyceryl distearate (Gattefosse, Saint-Priest France), Wecobee® M (Stepan, Northfield, IL), isopropyl alcohol (IPA; Merck, Darmstadt, Germany), formalin solution, neutral-buffered 10% (Sigma–Aldrich) and Start® M membranes (Merck Millipore, Billerica, MA) were purchased. Other chemicals were of analytical grade.
Preparation of proniosomal gels
Proniosomal gels containing OC were prepared by the coacervation phase separation method. First, precisely weighed amounts of soy lecithin, cholesterol, surfactant, glyceryl distearate and drug were added to 0.5 mL of IPA and heated over a water-bath (80–100 °C) until they had dissolved completely. Then, 0.1% glycerol solution was added and warmed until a clear solution was obtained on the water-bath. A proniosomal gel was obtained upon cooling of the solution, which was preserved in the dark for further evaluation (Sandhya et al., Citation2013). Compositions of proniosomal gels are shown in .
Table 1. Composition of oxybutynin chloride proniosomal gels.
Characterization of proniosomal gels
Entrapment efficiency (EE)
To evaluate the loading capacity of proniosomal systems, OC and gel (100 mg) were dispersed in phosphate-buffered saline (PBS) at pH 7.4 and centrifugal force 12 074 × g for 30 min at 5 °C (5810R; Eppendorf, Hamburg, Germany). The supernatant layer was used for the determination of free drug by high-performance liquid chromatography (HPLC) (Thomas & Viswanad, Citation2012).
Vesicle size
The vesicle size of each formulation was determined after suitable dilution by a Zetasizer Nano ZS90 Particle Analyzer (Malvern Instruments, Malvern, UK). Results are shown in .
Table 2. Entrapment efficiency and vesicle size of hydrated proniosomes.
Surface morphology
A small amount of selected proniosomal gel was placed on an optical microscope as well as on a holding disk and analyzed, respectively. Then, a drop of distilled water was added. The disk was placed in a chamber inside an Environmental Scanning Electron Microscope (eSEM: Quanta 450 FEG; FEI, Hillsboro, OR). Water within the sample was allowed to evaporate before examination.
Stability
Selected formulations P3 and P4 were stored at room temperature (25 ± 2 °C) and oven temperature (45 ± 2 °C) (Radha et al., Citation2013). After 1 month, EE was determined, as described previously.
Attenuated total reflectance-Fourier transform infrared spectroscopy
The materials used and formulations were analyzed by attenuated total reflectance-Fourier transform infrared (ATR-FTIR) spectroscopy on a Magma-IR™ Spectrometer 750 (Nicolet Instruments, Madison, WI) equipped with a Golden Gate™ Single Reflection Diamond ATR system. Spectra are presented in .
Table 3. Change in entrapment efficiency after storage for 3 months.
In vitro skin-permeation study
Franz (vertical) diffusion cells (PermeGear, Hellertown, PA) were used for skin-permeation studies in vitro. This study was carried out following the method of Rajan et al. (Citation2008) with slight modification: instead of animal skin, a Strat® M membrane (Merck Millipore) was mounted. The amount of OC in the buffer solution was analyzed by a μBondapak Cyano Reverse-phase HPLC column with sample injection of 20 µL. The mobile phase had two solvents: (i) a mixture of water, methanol and triethyl amine (3200:800:0.9, v/v/v), pH adjusted to 3.5 with phosphoric acid; (ii) acetonitrile (80:20, v/v). The OC proniosomal gel was identified at 203 nm with a UV detector at a flow rate of 2.0 mL/min (Bae & Park, Citation2015).
In vivo animal study
Acetic acid-induced bladder hyperactivity
Acetic acid (ACA) solution (0.75%) was administered to unconscious rats by a urinary catheter. Chemical irritation caused by ACA produces acute inflammation of the urinary bladder and, consequently, facilitates micturition in rats (Oki et al., Citation2006).
Salivary secretion
Measurement of salivary secretion was undertaken according to the method described by Oki et al. (Citation2006). Rats were anaesthetized with diethyl ether. Effects of proniosomal gels and oral administration of OC given 1, 3, 12 and 24 h after pilocarpine (cumulative dose)-induced salivary secretion were examined (Oki et al., Citation2006).
Histopathology
The urinary bladder was removed from each group of rats and immersed in formalin (10%) immediately. Tissue sections (thickness, 5 mm) were stained with hematoxylin and eosin and, after processing and embedding, were observed under a light microscope.
Statistical analyses
Statistical analyses of data were undertaken using SPSS version 8.0 (IBM, Armonk, NY). Analysis of variance and the paired t-test were applied. p < 0.05 was considered significant. Values are the mean ± standard deviation.
Results
EE and vesicle size
The different surfactants and lipids used to prepare OC proniosomal gels are shown in . Results of EE and vesicle size are presented in . All formulations showed EE of >87%. Sizes of vesicles formed were 0.38–5.01 μm (). Among gel formulations, P3 (which contained Span 20:Span 60 at 1:1) had the smallest vesicle size, whereas P4 (Tween 20:Tween 80 at 1:1) had the largest vesicle size. EE of P7 was lower than that for P4, but glyceryl distearate could improve vesicle rigidity, which suggested that some leakage of the drug (Nimbalkar et al., Citation2011). Furthermore, increasing the amount of surfactant increased EE significantly. P6 had higher EE than that for P5 probably because P6 contained 900 mg of Tween 80 whereas P5 contained 100 mg of Tween 80 (p < 0.05).
Surface morphology
Optical microscopy revealed that P3 () and P4 () contained proniosomes () and that, after hydration with distilled water, liquid crystals were formed in spherical vesicles ().
Figure 1. Optical microscope images. (A, B) P3 gel with lower and higher levels of hydration, respectively; (C, D) P4 gel with lower and higher levels of hydration, respectively.
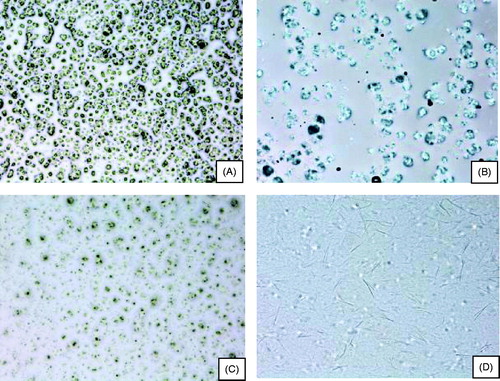
eSEM imaging revealed that the proniosomes formed were spherical and homogenous in P3 () and P4 () at low and high magnifications. These results are consistent with the EE of P3, in which the drug was entrapped perfectly in the micellar system with some loosened pores. For P4 (which contained Tween 20 and Tween 80 without glyceryl distearate), twisted, matted lamellar strands of proniosomes without precipitates were observed.
Stability
Stability studies were carried out by storing P3 and P4 formulations of proniosomes at various temperatures [refrigeration (2–8 °C), room temperature (25 ± 0.5 °C) and elevated temperature (45 ± 0.5 °C)] for 3 months. Variations in mean diameter of vesicles and drug content are presented in .
ATR-FTIR spectroscopy
ATR-FTIR spectroscopy was used to scan individual excipients and gels P3 and P4 ( and ) at a resolution of 4 cm–1 in the frequency range 650–4000 cm–1 to observe spectral shifts in proniosomal gels. FTIR spectra of OC, cholesterol, Tween 20, Tween 80 and Wecobee, as well as OC, cholesterol, Span 20, Span 60 and Wecobee with proniosomal gels are shown in and .
In vitro permeation studies
Proniosomes must be hydrated so that niosomal vesicles can be formed before the drug permeates across the skin. In vitro permeation studies are undertaken in a non-occlusive environment to better simulate topical application of proniosomal gels (Balakrishnan et al., Citation2009). Percent cumulative permeation (PCP) and permeation rate (µgm/cm2 h) for proniosomal gels after 8 h are shown in . PCP and permeation rate were higher for gels containing Span (72–81%) compared with those containing Tween (51–64%). PCP for proniosomal gels was plotted against time (). PCP after 8 h for gels containing Span was higher than that for gels containing Tween (p < 0.05).
Figure 5. Percent cumulative permeation of various proniosomal formulations through mouse skin in vitro. Each plot represents the mean ± SD of 4 rats with p < 0.05.
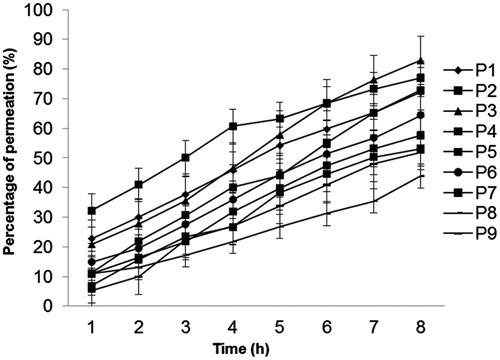
Table 4. Percent cumulative permeation and permeation rate of oxybutynin chloride proniosomes across mouse skin in vitro.
In vivo animal studies
Based on the results of EE and in vitro permeation study, P3 and P4 gels were selected for in vivo animal study. Weights of whole saliva secreted in the first 10 min in control, OC-treated, P3-treated, and P4-treated rats were similar 1–24 h after pilocarpine stimulation (). Compared with control rats, the weight of whole saliva secreted was increased markedly after oral administration of OC. Transdermal application of P3 and P4 gels markedly decreased pilocarpine-induced salivation (). Nevertheless, the respective weights (246.9, 217.4 and 279.9 mg) of whole saliva secreted at 2, 4 and 12 h were significantly higher (p < 0.05) than those 1 and 4 h after oral administration of OC. clearly depicts the recovery of pilocarpine-induced salivation after transdermal administration of OC.
Figure 6. (A) Effect of control, oral and transdermal administration of oxybutynin chloride proniosomal gel (P3 and P4) on pilocarpine-induced salivary secretion in rats. (B) Recovery of pilocarpine-induced salivary secretion after transdermal administration of oxybutynin chloride in rats. Each column represents the mean ± SD of four rats. Statistically significant data (p < 0.05 = ***).
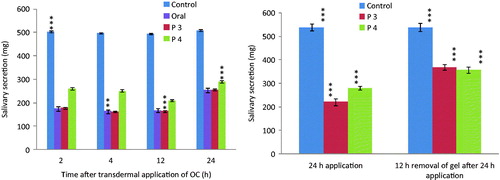
Histopathology
The control group showed a normal urinary bladder with multiple layers of epithelial cells. The transitional epithelium was resting on a submucosal layer comprising fibrous connective tissue, beneath which was a layer of irregular muscle fibers ().
Figure 7. Histologic images of bladders in different groups of rats. (A) control; (B and C) acetic acid-induced overactive bladder; (D) oxybutynin treated (p.o.); (E) P3 gel-treated; (F) P4 gel-treated.
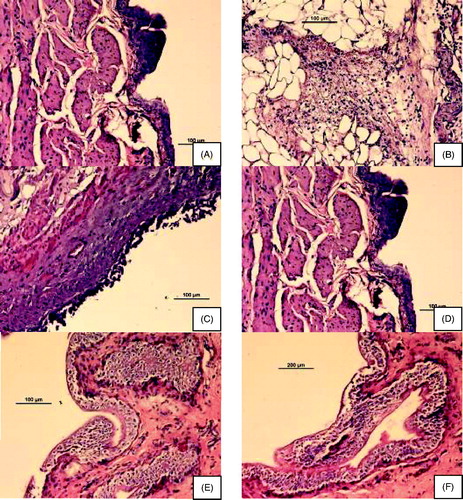
In the ACA-induced group (), necrosis of the transitional epithelium and focal areas of discontinuity and ulceration of the mucosa were observed. Submucosal and muscle layers showed inflammatory infiltrates composed predominantly of neutrophils and scattered lymphocytes, suggesting an acute form of inflammation in bladder tissue that extended throughout its thickness.
OC-, P3- and P4-treated groups are shown in , respectively. P3- and P4-treated groups showed highly regenerative surfaces of the transitional epithelium.
Discussion
Entrapment efficiency and vesicle size
Formulations with cholesterol exhibit higher EE because Span is more hydrophobic than Tween, with a lower hydrophilic–lipophilic balance (HLB) value of 8.6 for Span 20 and 4.7 for Span 60 (Rawat et al., Citation2011). However, the HLB is not the only factor that affects EE. The phase transition temperature (Tc) is directly proportional to the length of hydrocarbon alkyl chains of surfactants (Mohammed et al., Citation2004; Guinedi et al., Citation2005; El-Laithy et al., Citation2011). Surfactants with a higher Tc and longer alkyl chain show higher EE because they are more likely to form gels in an ordered form with less “leaky” niosomes (El-Laithy et al., Citation2011). Addition of cholesterol to formulations enables more hydrophobic surfactants to form vesicles, suppresses surfactant aggregation and provides greater stability to the lipid bilayer by increasing the gel-to-fluid transition temperature of vesicles (Lawrence et al., Citation1996).
In Span-containing formulations, increasing hydrophobicity using various types of lipids results in reduction of water intake by niosome bilayers and surface free energy, leading to smaller vesicle sizes (El-Laithy et al., Citation2011). This study suggests that use of different lipids does not increase hydrophobicity in Tween-containing formulations, and that the particle size remains large. It has been proposed that, in general, vesicle size is dependent upon the alkyl chain length of the surfactant, whereby a longer alkyl chain leads to a larger vesicle size. Highly lipophilic drugs such as OC (log P = 5.049) are intercalated almost completely within the bilayered vesicles formed by hydrophobic non-ionic surfactants (Vanhal et al., Citation1996; Alsarra et al., Citation2005). These changes lead to significant difference in EE even among Tween-containing formulations.
Surface morphology
By increasing the hydration of proniosomes in aqueous media, niosomes are formed. These surfactants self-assemble into spherical vesicles, tubular, disk-like vesicles and vesicles with faceted edges known as “polyhedral niosomes” (Uchegbu et al., Citation1997). A homogenously dispersed drug in formed vesicles in the lipid bilayer increases the chance of constant-release permeation (Nimbalkar et al., Citation2011). Incorporation of a drug in a micellar system with few loosened pores leads to higher EE. In addition, formulations containing cholesterol tend to have higher EE than formulations containing glyceryl distearate, probably because the structure of cholesterol enables such formulations to act as “vesicular cement” and fill-in the spaces between bilayers assembled by surfactant monomers (Agarwal et al., Citation2001). Furthermore, cholesterol entraps vesicle pores but glyceryl distearate forms loose pores. Drugs with fine crystals and sharp edges are believed to reduce EE due to pore formation, which leads to leakage (Uchegbu & Florence, Citation1995).
Stability
In our stability study, the higher leakage of drug observed from vesicles at high temperature may have been due to the loosening of vesicles and higher fluidity of lipid bilayers (Radha et al., Citation2013), whereas it was expected to exhibit good stability at low temperatures (2–8 °C). Cholesterol also influences membrane permeability, EE and bilayer rigidity (Nimbalkar et al., Citation2011).
ATR-FTIR spectroscopy
Interaction between cholesterol and Span 60 in the bilayer of niosomes is due to hydrogen bonding (Kumar & Rajeshwarrao, Citation2011), which leads to aggregated and bilayer vesicles during niosome formation. Tween surfactants bear long alkyl chains and a large hydrophilic moiety in combination with cholesterol that leads to a clear spectral wavelength of OC (Arunothayanun et al., Citation2000).
In vitro permeation studies
If cholesterol and non-ionic surfactants are present in proniosomes, both can act as “penetration enhancers” to increase OC permeation. Sarpotdar & Zatz (Citation1986) hypothesized that a high thermodynamic activity gradient of the active constituent at the interface due to adsorption/fusion of vesicles onto the skin surface alters the structure of stratum corneum thanks to intracellular loosening of lipids, thereby leading to increased permeability after treatment with niosomes (Sarpotdar & Zatz, Citation1986). That hypothesis has been supported by various review articles. Cholesterol can influence niosome structure during permeation studies because niosomes interact with biological membranes (which contain cholesterol); hence, drug permeability is elevated by increases in fusion processes and elasticity, as well as changes in size and shape (Nasseri, Citation2005). This phenomenon may explain the higher permeation rate in Span plus cholesterol-containing formulations than in those containing Tween. In our permeation study, the skin membrane was moistened by PBS, which converts proniosomes into niosomes. This conversion leads to changes in consistency and fluidity, resulting in flexible vesicles that increase permeation of a drug (Liu et al., Citation2000, Citation2007). Proniosomal gels prepared with Tween incorporating cholesterol are expected to have higher PCP than formulations incorporating glyceryl distearate due to the higher lipophilicity of cholesterol. Cholesterol is present in skin membranes, where it can influence membrane properties such as ion permeability, fusion processes, and elasticity (Kumar & Rajeshwarrao, Citation2011). The drug-permeation profile is fairly constant and concentration-dependent with first-order kinetics due to the presence of non-ionic surfactants (Rajan et al., Citation2010).
In vivo animal studies
Salivary secretion
Increased accumulation of a drug in the body due to a long exposure time leads to the prolonged side effects of the drug. Ideally, reversal of the effects of a dry mouth (whereby saliva secretion is returned to normal levels) is the best option (Hesch, Citation2007; Kennelly, Citation2010). Such reversal can be achieved by absorption of IPA, an inhibitor of cytochrome P450 (present in the skin), which leads to a decrease in the conversion of oxybutynin to N-DEO. It is believed that dry mouth (a side effect of anticholinergic agents) is correlated with the plasma concentration of N-DEO (a metabolite of oxybutynin) (Sathyan et al., Citation2001).
Histopathology
The absence of inflammatory cells with a near-normal urinary bladder containing multiple layers of epithelial cells denotes healing tissue. A highly regenerative surface of the transitional epithelium denotes healthy tissue. Epithelial cells with multiple layers and cuboidal cells with prominent nuclei denote normal tissue. In our experiments, the mucosal surface had numerous papillary folds and was clustered with transitional cells. The submucosa showed fibrosis and blood vessels, thereby suggesting healing scar tissue.
Conclusion
This study suggests that, among the formulations studied, P3 gel had the most effective combination of surfactant, alcohol and aqueous phase. It also possessed the highest EE, drug permeation, therapeutic effect, including highly regenerative bladder surfaces and faster salivary secretion recovery. This work has established the foundation for future studies on the potential of OC proniosomal gels for transdermal delivery.
Declaration of interest
The author reports no conflicts of interest in this work. The authors express their sincere gratitude to the Research Laboratory of the International Medical University for providing financial (No. B0109_Res182012) and laboratory facilities for the study.
References
- Abrams P, Cardozo L, Fall M, et al. (2002). The standardization of terminology of lower urinary tract function: report from the standardization sub-committee of the International Continence Society. Neurourol Urodyn 21:167–78
- Agarwal R, Katare OP, Vyas SP. (2001). Preparation and in vitro evaluation of liposomal/niosomal delivery systems for antipsoriatic drug dithranol. Int J Pharm 228:43–52
- Alsarra IA, Bosela AA, Ahmed SM, Mahrous GM. (2005). Proniosomes as a drug carrier for transdermal delivery of ketorolac. Eur J Pharm Biopharm 59:485–90
- Appell RA. (2002). The newer antimuscarinic drugs: bladder control with less dry mouth. Cleve Clin J Med 69:761–9
- Arunothayanun P, Bernard MS, Craig DQ, et al. (2000). The effect of processing variables on the physical characteristics of non-ionic surfactant vesicles (niosomes) formed from a hexadecyl diglycerol ether. Int J Pharm 201:7–14
- Bae J, Park JW. (2015). Formulation and pharmacokinetic evaluation of controlled-release oxybutynin tablets in dogs. Trop J Pharm Res 14:363–9
- Balakrishnan P, Shanmugam S, Lee WS, et al. (2009). Formulation and in vitro assessment of minoxidil niosomes for enhanced skin delivery. Int J Pharm 377:1–8
- Dmochowski RR, Sand PK, Zinner NR. (2003). Comparative efficacy and safety of transdermal oxybutynin and oral tolterodine versus placebo in previously treated patients with urge and mixed urinary incontinence. Urology 62:237–42
- El-Laithy HM, Shoukry O, Mahran LG. (2011). Novel sugar esters proniosomes for transdermal delivery of vinpocetine: preclinical and clinical studies. Eur J Pharm Biopharm 77:43–55
- Guinedi AS, Mortada ND, Mansour S, Hathout RM. (2005). Preparation and evaluation of reverse-phase evaporation and multilamellar niosomes as ophthalmic carriers of acetazolamide. Int J Pharm 306:71–82
- Hesch K. (2007). Agents for treatment of overactive bladder: a therapeutic class review. Proc (Bayl Univ Med Cent) 20:307–14
- Kennelly MJ. (2010). A comparative review of oxybutynin chloride formulations: pharmacokinetics and therapeutic efficacy in overactive bladder. Rev Urol 12:12–19
- Krause P, Fuhr U, Schnitker J, et al. (2013). Pharmacokinetics of intravesical versus oral oxybutynin in healthy adults: results of an open label, randomized, prospective clinical study. J Urol 190:1791–7
- Kumar GP, Rajeshwarrao P. (2011). Nonionic surfactant vesicular systems for effective drug delivery – an overview. Acta Pharm Sinica B 1:208–19
- Lawrence MJ, Chauhan S, Lawrence SM, Barlow DJ. (1996). The formation, characterization and stability of non-ionic surfactant vesicles. STP Pharm Sci 1:49–60
- Liu D, Chen W, Tsai L, Yang S. (2000). Microcalorimetric studies of the effects of cholesterol on the physical stability of liposome. Colloids Surf B Biointerfaces 172:57–67
- Liu T, Guo R, Hua W, Qiu J. (2007). Structure behaviors of hemoglobin in PEG 6000/Tween 80/Span 80/H2O niosome system. Colloids Surf A Physicochem Eng Aspects 293:255–61
- Mohammed AR, Weston N, Coombes AGA, et al. (2004). Liposome formulation of poorly soluble drugs: optimization of drug loading and ESEM analysis of stability. Int J Pharm 285:23–34
- Nasseri B. (2005). Effect of cholesterol and temperature on the elastic properties of niosomal membranes. Int J Pharm 300:95–101
- Nimbalkar UA, Dhoka MV, Sonawane PA. (2011). Solid lipid nanoparticles for enhancement of oral bioavailability of cefpodoxime proxetil. Int J Pharm Sci Res 2:2974–82
- Nitti VW, Sanders S, Staskin DR, et al. (2006). Transdermal delivery of drugs for urologic applications: basic principles and applications. Urology 67:657–64
- Oki T, Toma-Okura A, Yamada S. (2006). Advantages for transdermal over oral oxybutynin to treat overactive bladder: muscarinic receptor binding, plasma drug concentration and salivary secretion. J Pharm Exp Ther 316:1137–45
- Radha GV, Rani TS, Sarvani B. (2013). A review on proniosomal drug delivery system for targeted drug action. J Basic Clin Pharm 4:42–8
- Radomski SB, Barkin J. (2012). Medical management of overactive bladder. Can J Urol 19:1–9
- Rajan R, Khanam J, Nanda A. (2008). Design of a matrix patch formulation for long-acting permeation of diclofenac potassium. Asian J Pharm Sci 3:30–9
- Rajan R, Sheba RND, Jasmina K, et al. (2010). Studies on the effect of plasticizer on in vitro release and ex vivo permeation from Eudragit E 100 based chlorpheniramine maleate matrix type transdermal delivery system. J Exc Food Chem 1:3–12
- Rawat AS, Kumar MS, Khurana B, Mahadevan N. (2011). Proniosomal gel: a novel topical delivery system. IJAPR 3:1–10
- Salvatore S, Soligo M, Proietti F. (2005). Overactive bladder syndrome: considerations in pharmacotherapy and new perspectives. Eur J Obstet Gynecol Reprod Biol 120:129–33
- Sand P, Zinner N, Newman D. (2007). Oxybutynin transdermal system improves the quality of life in adults with overactive bladder: a multicenter, community-based, randomized study. BJU Int 99:836–44
- Sandhya P, Mehvash SF, Subrahmanyam CVS. (2013). Enhancement of permeability of losartan potassium by formulating as proniosomal gel. IJBPR 4:743–8
- Sarpotdar PP, Zatz JL. (1986). Percutaneous absorption enhancement by non-ionic surfactants. Drug Dev Ind Pharm 12:1625–47
- Sathyan G, Chancellor MB, Gupta SK. (2001). Effect of OROS® controlled-release delivery on the pharmacokinetics and pharmacodynamics of oxybutynin chloride. Br J Clin Pharmacol 52:409–17
- Sussman DO. (2007). Overactive bladder: treatment options in primary care medicine. JAOA 107:379–85
- Thomas L, Viswanad V. (2012). Formulation and optimization of clotrimazole-loaded proniosomal gel using a 32 factorial design. Sci Pharm 80:731–48
- Uchegbu IF, Florence AT. (1995). Non-ionic surfactant vesicles (niosomes): physical and pharmaceutical chemistry. Adv Colloid Interface Sci 58:1–55
- Uchegbu IF, Schätzlein A, Vanlerberghe G, et al. (1997). Polyhedral Non-ionic Surfactant Vesicles. J Pharm & Pharmacol 49:606–10
- Van Der Vaart CH, De Leeuw JRJ, Roovers JPWR, et al. (2002). The effect of urinary incontinence and overactive symptoms on quality of life in young women. BJU Int 90:544–9
- Vanhal D, Vanrensen A, Devringer T, et al. (1996). Diffusion of estradiol from nonionic surfactant vesicles through human stratum-corneum in vitro. STP Pharm Sci 6:72–8
- Zobrist RH, Schmid B, Feick A, et al. (2001). Pharmacokinetics of the R- and S- enantiomers of oxybutynin and N-desethyloxybutynin following oral and transdermal administration of the racemate in healthy volunteers. Pharmacol Res 18:1029–34