Abstract
Gambogic acid (GA) is a naturally derived potent anticancer agent with extremely poor aqueous solubility. In the present study, positively charged PEGylated liposomal formulation of GA (GAL) was developed for parenteral delivery for the treatment of triple-negative breast cancer (TNBC). The GAL was formulated with a particle size of 107.3 ± 10.6 nm with +32 mV zeta potential. GAL showed very minimal release of GA over 24 h period confirming the non-leakiness and stability of liposomes. In vitro cytotoxicity assays showed similar cell killing with GA and GAL against MDA-MB-231 cells but significantly higher inhibition of HUVEC growth was observed with GAL. Furthermore, GAL significantly (p < 0.05) inhibited the MDA-MB-231 orthotopic xenograft tumor growth with >50% reduction of tumor volume and reduction in tumor weight by 1.7-fold and 2.2-fold when compared to GA and controls, respectively. Results of western blot analysis indicated that GAL significantly suppressed the expression of apoptotic markers, bcl2, cyclinD1, survivin and microvessel density marker-CD31 and increased the expression of p53 and Bax compared to GA and control. Collectively, these data provide further support for the potential applications of cationic GAL in its intravenous delivery and its significant role in inhibiting angiogenesis against TNBC.
Introduction
Currently, patients with triple-negative breast cancer (TNBC) have neither effective targeted therapy nor do the breast cancer clinicians have a biomarker to identify aggressive and recurrent TNBC. To date, TNBCs are managed with standard chemotherapy but consequently, relapses are high due to acquired resistance and over 522 000 deaths per year from breast cancer worldwide as a direct result of this disease (Tao et al., Citation2014). TNBC accounts for 15% of all invasive breast cancers. It is a higher grade cancer and occurs at higher rates in young and African-American women (Palmer et al., Citation2014). Moreover, unlike the other type of solid tumor, TNBC patients have susceptibility toward the development of visceral metastases early in the course of the disease. Developments of effective approaches with improved treatment potential against these cancers are critical as the survival rate of patients with metastatic TNBC is very few months (Bonotto et al., Citation2014). Very poor prognosis and limited treatment options due to lack to typical breast cancer markers are the two basic factors compromising the cure of TNBC today. Therefore, there is an urgent need to develop new drugs or novel formulation strategy to potentiate the therapeutic outcome to combat TNBC.
Gambogic acid (GA) is a recently explored polyprenylated xanthene from the Garcinia hanburryi tree for its multiple therapeutic actions. GA has demonstrated significant anticancer activity against various cancers, both in vitro and in vivo conditions (Wang & Chen, Citation2012). GA was reported to act via multiple mechanisms promoting the tumor growth which involves apoptosis, cell cycle arrest, telomerase inhibition, anti-angiogenesis activity and an anti-metastasis effect (Zou et al., Citation2012). Lu et al. (Citation2007) have reported that GA possesses both anticancer and anti-angiogenesis activity. As like other potent natural origin anticancer drugs (paclitaxel, doxorubicin, camptothecin, etc.), in future GA could transfer to clinical studies.
The major hurdle for clinical application of GA would be its extremely poor water solubility (<5 ppm) and very short biological half-life (less than 1 h in dogs and less than 20 min in rats) (Liu et al., Citation2006). It has been demonstrated that, by using surfactant micelles, the solubility of GA was increased and which results in enhanced in vivo anticancer activity. For preclinical studies, it has served the purpose but such surfactant have one or the multiple side effects, such as life-threatening hypersensitivity reactions, vascular stimulation, hemolytic toxicity, neurotoxicity, nephrotoxicity and cardiotoxicity, which discourage its application for the clinical purpose (Qi et al., Citation2008b). Similar problems are observed with the existing taxane formulations, and extensive research has been carried out to develop a safe and effective parenteral formulation with extended half-life.
PEGylated liposomes could be the most suitable formulation approach for parenteral delivery of GA because of lipophilic nature of GA and superior in vivo biocompatibility of PEGylated liposomes (Adlakha-Hutcheon et al., Citation1999; Chang & Yeh, Citation2012). The liposomal formulation is a safe, industry feasible and therapeutically superior alternative to solvent and surfactant-based formulation approach. There are several reports on supporting encapsulation of various anticancer drugs (doxorubicin, mitoxantrone, paclitaxel, docetaxel, etc.) into liposomes (Immordino et al., Citation2006; Deshpande et al., Citation2013). Many liposomal drugs are already approved for clinical formulations, such as AmBisome®, Doxil®, DaunoXome® and Marqibo® while others are under clinical trial (Chang & Yeh, Citation2012). Nanocarriers-based solubilization approach is preferred over other approaches because of additional advantages of nanocarriers in facilitating tumor uptake of the drug. Nanocarriers are passively accumulated into tumor because of enhanced permeation and retention (EPR) effect of leaky neovasculature of the tumor which minimizes the off-target side effects of the anticancer drugs. Doxorubicin-loaded liposomes showed a significant reduction in cardiotoxicity side effect of the drug and provides better therapeutic effect at a low dose (Xing et al., Citation2015). Surface PEGylation plays a crucial role in prolonging the circulation life of cationic liposome in blood circulation. It helps in two ways; enhance the probability of accumulation into the tumor by EPR effect and provide prolong exposure of drug to cancer cells. It has also been demonstrated that positively charged nanocarriers were preferentially bound to angiogenic blood vessels of the tumor and enhances intracellular uptake of the drug in cancer cells (Sawant & Torchilin, Citation2012). Due to more negative charge of neovasculature compared to healthy vasculature, cationic PEGylated liposomes will be of paramount significance to target the tumor more efficiently. Endothelial cells targeting of tumor can be achieved without anchoring a ligand on the liposomal surface. Targeting tumor endothelium could help in inhibiting the tumor growth at a reduced dose. GA is an ideal candidate for cationic PEGylated delivery because it has both anticancer and antiangiogenic effects. Such surface modifications are very facile in liposomal formulation compared to other type of nanocarriers.
In the present study, GA-loaded positively charged PEGylated liposomes were developed and characterized for parenteral delivery of GA and to achieve tumor neovasculature specific delivery. The formulation could be a potentially viable clinical approach for the treatment of TNBC.
Materials and methods
Chemicals and drugs
GA was purchased from Santa Cruz Biotechnology (Ann Arbor, MI) and dimethyl sulfoxide (DMSO) was purchased from Sigma-Aldrich (St. Louis, MO). Fetal bovine serum (FBS) from Invitrogen (Grand Island, NY) and an antibiotic mixture containing penicillin (5000 U/ml), streptomycin (0.1 mg/ml) and neomycin (0.2 mg/ml) from Sigma-Aldrich. Dulbecco’s modified Eagle’s medium (DMEM)/F-12 medium from Invitrogen and HUVEC cells and other cell culture materials like endothelial growth media and growth factors were purchased from Lonza (Basel, Switzerland). N-[1-(2,3-dioleoyloxy)propyl]-N, N, N-trimethylammonium methyl-sulfate (DOTAP), dipalmitoyl phosphatidylcho-line (DPPC) and N-(carbonyl-methoxy polyethylene glycol 2000)-1,2-distearoyl-sn-glycero-3-phosphoethanolamine, sodium salt (DSPE-PEG-2000) were purchased from Lipoid (Ludwigshafen, Germany).
HPLC analysis
HPLC-UV method was developed to analyze GA for release studies. Chromatographic separation was achieved on Waters 717 instrument equipped with a Waters symmetry (250 mm/4.6 mm/5 μm) column using ACN:phosphate buffer pH 3 (90:10) as mobile phase with a flow rate of 1 ml/min, detected at 290 nm. The retention time of GA was 14.7 ± 0.15 min.
Cell culture
MDA-MB-231, Hep G2 and MIA PaCa-2 cells were obtained from American Type Culture Collection and maintained in DMEM (Sigma-Aldrich) nutrient mixture supplemented with 10% FBS and antibiotic-antimycotic mixture comprising penicillin (5000 U/ml), streptomycin (0.1 mg/ml) and neomycin (0.2 mg/ml) at 37 °C. When cells became approximately 80–90% confluent, cells lines were subcultured with 0.25% trypsin-EDTA (Invitrogen).
Animals
Female Nu/Nu mice (six-weeks old from Harlan, Indianapolis, IN) were grouped and housed (n = 5 per cage) in sterile microisolator caging unit supplied with autoclaved Tek-Fresh bedding. The animals were housed at Florida A&M University in accordance with the standards of the Guide for the Care and Use of Laboratory Animals and the Association for Assessment and Accreditation of Laboratory Animal Care.
Preparation and characterization of liposomes containing gambogic acid (GAL)
Different batches of GAL were prepared using ethanol injection – ultrasonication method. Briefly, GA, DPPC, cholesterol, DOTAP and DSPE-PEG 2000 in 1:14:6:2:0.6 molar ratios were dissolved in absolute ethanol and injected into water at 55 °C with constant stirring. After a minute, the mixture was sonicated for 2 min using a probe sonicator at 400 W, 30% amplitude (Branson, Danbury, CT) to reduce the particle size. Liposomes were further stirred for 1 h at 55 °C to evaporate the ethanol. The weight ratio of GA to phospholipids was varied to optimize the GA loading and particle size. We have used the lowest amount of ethanol because ethanol is difficult to evaporate and affect the long-term physical stability of liposomes. Further, sonication was done to achieve particle size around 100 nm. Release study of GA loaded liposomes and GA solution in DMSO was carried out by dialysis bag method. Drug release study was performed at 37 °C under shaking (100 rpm) which was monitored using a dialysis bag containing phosphate-buffered saline (PBS) with the addition of 0.5% Tween-80 as a sink solution at pH 7.4. GA solution was prepared by dissolving 20 mg of GA in 1 ml of ethanol:Tween-80 (1:1) solution. For animal study, it was diluted appropriately with water for injection. Data are calculated from triplicate experiments and presented as mean, and error bars refer to SD.
In vitro cytotoxicity of GA and GAL
MDA-MB-231, HepG2 and MIA Paca-2 cells were seeded at a density of 10 000 cells/well in 96-well plates and allowed to adhere for 24 h. Cells were exposed to different concentration of GA and GAL for 48 h in triplicate. Cytotoxicity was assessed at the end of drug exposure using crystal violet assay. Absorbance was measured at 545 nm using a microplate reader (ChroMate 4300, Palm city, FL). Untreated cells were used as control and results were expressed as the relative percentage of absorbance compared to control. Half-maximal inhibitory concentration (IC50) was calculated using Sigma Plot software, version 9.0 (SYSTAT Software, San Jose, CA).
Western blot analysis
For in vitro experiments, 3.2 μm of GA and 3.8 μm of GAL were used to prepare protein lysates for immunoblotting. The dose of GA 10 mg/kg was used for all the in vivo experiments. Protein samples for western blot analysis were extracted from MDA-MB-231 cells and tumor tissues by using RIPA lysis buffer according to Godugu et al. (Citation2014). Protein concentration was estimated by using BCA protein assay reagent kit. Equal amounts of 50 μg protein from different groups were denatured by heating for 5 min in SDS sample buffer and protein samples were separated by 10% SDS-PAGE. The protein samples separated on SDS-PAGE were transferred to nitrocellulose membranes for immunoblotting. After blocking, the membranes were incubated with skim milk (5% skim milk in 10 mm Tris-HCl (pH 7.6), 150 mm NaCl and 0.5% Tween-20) and probed with primary antibodies. The primary antibodies such as bcl-2, survivin, Bax, cyclin D1, p53, CD31 and β-actin were used in 1:1000 dilutions. The horse radish peroxidase (HRP)-conjugated secondary antibodies were used. Evaluation of expression of each protein was calculated in comparison to the expression of β-actin.
Analysis of miR-224 and miR-34b expression
MDA-MB-231 cells were treated with GA (3.2 μm) and GAL (3.8 μm) for microRNA analysis. miR-224 and 34b expression was carried out using 2 μg of total RNA for polyadenylation reaction analysis according to the manufacturer’s protocol (ABI Systems, Green Island, NY). At 37 °C for 30 min, the reaction mixture was incubated and the reaction tubes were immediately transferred to the ice to proceed for first-strand cDNA synthesis (ABI Systems) according to the manufacturer’s protocol. The target transcript levels (miR-224 and miR-34b) were normalized against U6 snRNA expression. Untreated cells were used as a control and compared the expression level of these miRNA’s to the control samples according to the Doddapaneni et al. (Citation2013). The data of qRT-PCR are expressed as the mean ± standard deviation (SD). All the experiments were carried out in triplicates.
In vitro HUVEC tube formation assay
HUVECs were grown in epithelial basal medium supplemented with 10% FBS at 37 °C and 5% CO2. Keeping 24-well culture plates on the ice, added chilled corning matrigel matrix (10 mg/ml) according to the manufacturer’s instructions. Briefly, plates were incubated at 37 °C for 30–60 min. The remaining liquid was carefully removed (medium) from the cultureware without disturbing the layer of corning matrigel matrix just before use. Endothelial cell suspensions was prepared by trypsinization and resuspending the cells in culture medium at 4 × 105 cells/ml. In order to study the effect, GA and GAL were added to the wells. HUVECs were suspended in EGM-2 medium containing various growth factors and drugs were seeded onto the matrigel. The effect of DTX on angiogenesis was also evaluated as a positive control by tube formation assay. After 12 h, cells were labeled by adding 300 μl/well of 8 μg/ml corning calcein AM in Hank’s balanced salt solution and plates were incubated for 30 min at 37 °C, 5% CO2. The cells were washed twice with PBS and photographed. Tube formation was calculated as follows: a three branch point event was taken as one tube and percentage inhibition expressed using untreated wells at 100%. This assay was repeated three times.
Anticancer studies in MDA-MB-231 cells induced breast cancer models
Triple-negative MDA-MB-231 breast carcinoma subcutaneous xenograft model was used for anticancer evaluations of GA PEGylated liposomes. MDA-MB-231 cells (1 million in 100 μl of phosphate buffer) were subcutaneously injected into the right flank of the mice. Two weeks after the tumor cells implantation, GA and GAL treatment was started and tumor sizes were measured using vernier caliper. At the time of the first dose of drug administration, the tumor volumes were approximately 100 mm3. Mice were randomly divided into three groups (n = 6 for each group) to receive IV injection of saline (control group), GA solution (10 mg/kg) and GAL (10 mg/kg). The therapy was continued six times at 2 d intervals through IV injection. The greatest longitudinal diameter (length) and the greatest transverse diameter (width) were measured. Tumor volumes were monitored every alternate day till the termination of the study.
Immunohistochemistry of CD31 expression in xenograft breast tumor model
Paraffin-embedded tumor tissues were deparaffinized and blocked for peroxidase activity for CD31 expression. Washed specimens with PBS twice, then specimens were incubated in normal goat serum according to the manufacturer’s instructions (Santa Cruz Biotechnology, Santa Cruz, CA). The section slides were washed with PBS twice and incubated with primary antibody against CD31 overnight at 4 °C. The HRP-conjugated secondary antibody was applied to locate the primary antibody (Santa Cruz Biotechnology). After three washes with PBS, the section slides were stained with Nova Red stain and counterstained with hematoxylin. The Olympus BX40 light microscope equipped with a computer-controlled digital camera (DP71, Olympus Center Valley, PA) was used to visualize the images on the slides.
Statistics
All data are presented as the mean ± SD or mean ± standard error of mean (SEM). The significance of the difference in treatment groups was determined using one-way ANOVA and Tukey’s multiple comparison test using GraphPad prism version 5.0 (San Diego, CA), where the value of p < 0.05 between the groups was considered as statistically significant difference between these groups.
Results
Preparation, characterization and in vitro drug release profile of liposomes
Different batches of GAL were prepared using ethanol injection – ultrasonication method. Particle size, entrapment efficiency and drug loading were optimized. Finally, optimized batch of GAL had a particle size of 107.3 ± 10.6 nm and 0.158 polydispersity index. The surface charge of GAL was positive with an average zeta potential of + 32 mV. Gel permeation chromatography showed 92.1 ± 2.7% entrapment efficiency of GA within liposomes. Drug loading did not show a remarkable difference in particle size. However, liposomes prepared with more than 10% w/w GA loading and 1.5 mg/ml final GA concentration were not stable for more than 2 h at room temperature. The in vitro release behavior of the GAL is shown in . GA solution showed the immediate and complete release of GA in release medium within 1 h. GAL showed much sustained and minimal release of GA. Only 11.65% of GA release was observed over the period of 24 h.
In vitro cytotoxicity of GA and GAL
In vitro cytotoxicity studies with GA against MDA-MB-231, Hep G2 and MIA PaCa-2 cells showed IC50 values of 3.2 ± 0.28, 4.06 ± 0.41 and 5.29 ± 0.53 μm, respectively (). The GAL treated MDA-MB-231, HepG2 and MIA PaCa-2 cells were showed IC50 values of 3.8 ± 0.34, 4.35 ± 0.48 and 5.61 ± 0.30 μm, respectively. All three cell lines showed more potency toward GA and GAL with little variations between their potencies. There was no significant difference between cytotoxicity of GA-free drug and GAL because permeability was similar for both free drug and liposome. Highly metastatic MDA-MB-231 human carcinoma cells were, however, selected for further studies as an in vitro model to study the effect of GA and GAL on TNBC.
Table 1. IC50 values of MDA-MB-231, Hep G2 and MIA PaCa-2 cells treated by GA and GAL for 48 h. Data represent mean ± SD (n = 3).
Analysis of apoptotic and survival markers
Western blot analysis of the antiapoptotic marker bcl-2 significantly (p < 0.01) down regulated in GAL-treated cells when compared to untreated control and GA-free drug-treated cells (). As shown in , expression of bcl-2 was found to be reduced (1.33-fold) in comparison to untreated control cells. The GAL resulted in a 0.81-fold higher repression in the bcl-2 expression compared to GA-free drug, suggesting the superior anticancer effects of GAL in breast cancer. The expression of survivin protein was significantly (p < 0.05) decreased (0.72 and 1.25-fold) in GA-free drug and GAL-treated cells compared to control cells, respectively (). The cell proliferating related protein cyclin D1 expressions were found to be higher in MDA-MB-231 control lysates. On treatment with GAL formulation, cyclin D1 was significantly (p < 0.01) down regulated. The relative expressions of cyclin D1 were found to be reduced 0.51 and 0.71-fold significantly in GA-free drug and GAL formulations, respectively compared to untreated control lysates (). The relative expression of p53 was increased in GA-free drug- (1.08-fold) and GAL- (2.30-fold) treated cells compared to control. In GA- and GAL-treated groups, Bax expression was increased significantly (p < 0.01) compared to untreated control cells, thus highlighting the potential of GAL inducing apoptosis in breast cancer cells ().
Figure 2. Determination of protein expression levels by western blot. (A) Expression of bcl2, survivin, Bax, cyclin D1 and p53 in comparison to the control β-actin in GA- and GAL-treated MDA-MB-231 cells by western blot. Untreated cells were used as control. (B) Western blot analysis of expression of different proteins up/downregulated significantly in GAL treated cells. Data are calculated from triplicate experiments and presented as mean, and error bars refer to SD, *p < 0.05, **p < 0.01 compared with control.
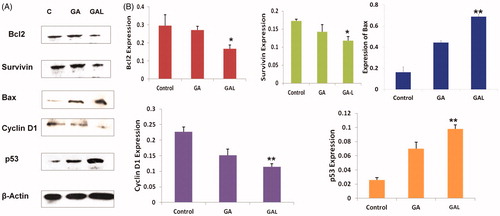
Real-time PCR analysis
The study was carried out to gain additional insights into the mechanisms/crucial factors regulating GA and GAL induced anticancer effects. We examined the expression pattern of miR-224 and miR-34b in MDA-MB-231 cells. A down-regulation of miR-224 () and up-regulation of miR-34b () expression was observed in cells treated with GA and GAL. As our primary data, the miR-224 was downregulated (1-fold) and miR-34 was up-regulated (1.7-fold) significantly (p < 0.05) in cells treated with GAL as compared with control cells which were treated with PBS (). However, miR-34b expression was significantly (p < 0.05) increased compared to control. Thus, these findings espouse the role of miR-224 and miR-34 in GA induction of anticancer effects.
Figure 3. Quantification of microRNA-224 and microRNA-34b expression by real-time PCR. MDA-MB-231 cells were treated with GA and GAL and quantified the expression of miR-224 and miR-34b. Each experiment was performed in triplicate. The expression level of each gene was normalized by snRNA and then compared to control cells. The means of the normalized microRNA expression values were calculated for each time point and expressed as relative fold changes (mean ± SD). *p < 0.05, compared with control.
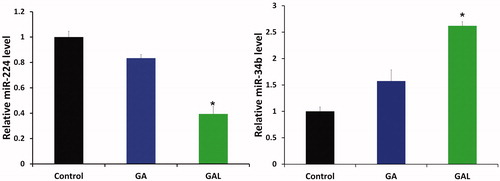
Anti-angiogenic effect
Compared to control group, a decrease in capillary tube branch point formations were observed in GA and GAL group (). We observed that an extensive meshwork of branched capillary-like tubules having multicentric junctions in the control group (). GAL () showed remarkable inhibition of branching point of HUVEC at as low as 50 nm concentration compared with docetaxel (). Effect of GAL was more pronounced with >90% inhibition of branching point compared to control while positive control docetaxel (20 nm) showed 80.0% inhibition ().
Figure 4. Effect of GA and GAL on angiogenesis by tube formation assay. HUVEC cells were treated with (A) no treatment, control (B) Docetaxel (C) GA and (D) GAL at indicated concentrations and docetaxel used as a positive control. (E) Analysis of percentage inhibition of angiogenesis by calculating branch points in GA and GAL-treated cells. Data are calculated from triplicate experiments and presented as mean, and error bars refer to SD, *p < 0.05 compared with control.
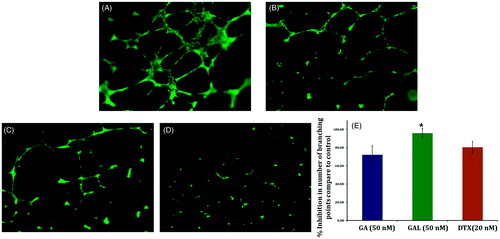
Antitumor activity of GA and GAL in vivo
The in vivo antitumor efficacy of GAL was investigated in MDA-MB-231 orthotropic xenograft tumor-bearing nude mice and compared with GA solution. As illustrated in , the treatment group showed significant tumor growth inhibition compared to the control group. In particular, the tumor volumes in the GAL group were much smaller than those of GA solution group (p < 0.05), indicating a statistically improved antitumor effect of GAL compared to the free drug group. Compared to untreated control groups, 1.31- and 2.0-fold, respective decrease in the tumor volume in GA-free drug and GAL-treated tumor groups was observed (). Similarly, the tumor weights of mice treated with GAL were significantly (p < 0.05) lower compared to GA-free solution (). There was no statistically significant change in the body weights of the animals treated with GA (25 ± 2.3 g) and GAL (25 ± 1.9 g) groups, suggesting the safety of the GA and GAL.
Antitumor and anti-angiogenic effects of GA and GAL in xenograft breast tumors
Next, we conducted the mechanistic studies to confirm the superior anticancer effects of GAL compared to GA-free drug-treated groups in vivo (). As shown by western blot analysis in , in GA-free drug and GAL groups, Bcl-2 expressions were found to be decreased (0.9 and 1.6-fold) significantly (p < 0.01), respectively compared to untreated control tumors. The GAL produced 0.7-fold higher repression in the bcl-2 expression compared to GA-free drug, suggesting the superior anticancer effects of GAL in breast cancer. Similarly, the cell survival marker survivin expression was also significantly (p < 0.05) down regulated to 0.6- and 1.79-fold in GA-free drug and GAL groups compared to the control group, respectively (). In regressed tumors, the GA and GAL treatments significantly (p < 0.05) decreased cyclin D1 expressions to a 0.8- and 1.4-fold, respectively, of controls. The tumor suppressor protein p53 levels also indicated the promising anticancer effects of GA in both free drug and liposome formulations. GA and GAL-treated groups significantly (p < 0.05) increased p53 expression to 1.51 and 2.36-fold, in regressed tumor samples compared to control. Similarly, the expression of Bax also increased significantly (p < 0.01) in GAL-treated groups than GA-free drug and control, thus providing the further evidence that GAL inducing apoptosis of breast cancer cells through bcl2/Bax. CD31 (platelet endothelial cell adhesion molecule-1) expression was significantly (p < 0.01) decreased in GAL-treated group than control or GA-treated group ().
Figure 6. In vivo apoptotic activity of GA and GAL in Balb/c nu/nu mice by western blot. (A) Expression of Bcl2, survivin, Bax, cyclin D1, p53 and CD31 in comparison to the control β-actin in GA and GAL-treated tumor tissues by western blot. The untreated animal group were maintained as control. (B) Western blot analysis of expression of different proteins up/downregulated in GA-treated cells. Data are calculated from triplicate experiments and presented as mean, and error bars refer to SD, *p < 0.05, **p < 0.01 compared with control.
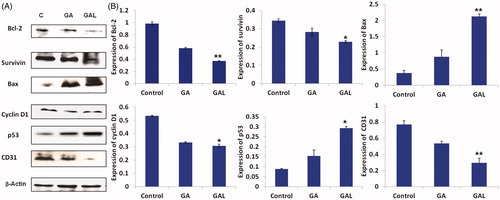
Further, the expression of CD31 was also confirmed by immunohistochemistry. The highest expression of CD31 was seen in tumor tissues harvested from untreated mice (). Decreased CD31 staining was observed in tumors treated with GAL () compared to tumors treated with GA alone (). All these results revealed that GA in free drug form and in liposome form significantly produced anticancer effects. Moreover, GAL formulation showed better anticancer and antiangiogenic effects than GA-free drug treated groups, which suggest the superior anticancer potential of our liposome formulation.
Discussion
Breast carcinoma is the leading cause of cancer-related death in women. The poor prognosis in breast carcinoma is due to its resistance to current therapies and to tumor metastasis (André & Zielinski, Citation2012). Development of an effective chemotherapeutic agent with the potential to trigger cancer cell death to prevent tumor invasion and metastasis is highly essential to prevent this form of cancer. Although GA potency as an anti-tumor agent has been demonstrated (Zou et al., Citation2012), the antitumor and antiangiogenic activities of GAL have not been explored yet. To the best of our knowledge, this is the first study concerning the use of GA loaded in cationic liposomes in TNBC therapy in vivo.
The high amount of organic solvent and surfactant are needed for parenteral delivery of GA because of its extremely poor aqueous solubility (<0.5 μg/ml) (Cai et al., Citation2014). For preclinical and clinical studies, we need at least 0.5 mg/ml solubility and physically stable solution in water for injection or saline. That means, 1000 times higher solubility, which can be achieved using solvent and surfactants. However, parenterally accepted non-ionic surfactants like polysorbate 80 and cremophor EL have severe life-threatening side effects. Therefore, there is a huge demand of solvent and surfactant-free formulations of existing anticancer molecules like paclitaxel and docetaxel as well (Patel et al., Citation2013). The liposomal formation is a safe, industry feasible and therapeutically superior alternative to solvent and surfactant-based formulation approach. GA is a highly lipophilic molecule with a log p of 7.6, so liposomal formulation could be the most appropriate choice for parenteral delivery. We used ethanol for liposomal preparation; but ethanol is difficult to evaporate, so we kept the formulation for a long time on the heated stirrer. Importantly, a marketed formulation like taxol and taxotere contains 2–3 ml of ethanol/dose, and thus it is very safe solvent for parenteral use. Hence, a trace amount of ethanol will not harm at all. GA can be easily solubilized and stabilized by entrapping within lipid bilayers of liposomes. The release profile of GAL depicted that GA was confined to lipid bilayers of liposomes and as long as the liposomes are stable release of GA would be difficult. Such release profile is very desirable for targeted drug delivery since drug will release only on liposomal disintegration after cellular internalization. It helps in minimizing the systemic exposure of drug and related side effects.
In vitro cytotoxicity studies showed that GAL retained the cytotoxicity of GA against MDA-MB-231 cell line. Once internalized, liposomes are degraded in endosomes and release the drug into the cytoplasm. Hence, the difference in the cytotoxicity between free GA and GAL was found to be negligible in vitro. In similar observations with our study, Saxena & Hussain (Citation2012) demonstrated that GA encapsulated in poloxamer 407/TPGS showed comparable in vitro cytotoxicity than free GA in breast cancer MCF-7 cells (Saxena & Hussain, Citation2012).
Interestingly, a huge difference in anti-angiogenic activity of free GA and GAL was observed in HUVEC study. Angiogenic endothelial cells are negatively charged which might affect their interaction with the chemical nature of drug and nanocarrier. As GA is an anionic molecule while GAL has cationic charge. We can assume that intracellular GA concentration would be much higher when given as GAL compared to free GA solution. Lu et al. (Citation2007) showed that GA inhibits angiogenesis and secretion of VEGF reduced from tumor cells. Furthermore, Qi et al. (Citation2008a) reported that when the cells were treated with GA in vitro, expression of extracellular matrix proteins (like MMP-2 and MMP-9) was decreased. To study the possible mechanisms involved in the enhanced cytotoxicity of GA-free drug and GAL, we evaluated apoptosis and HUVEC tube formation of tumor cells. It has been suggested that apoptosis may represent a protective mechanism against neoplastic development as it eliminates genetically damaged cells or excess cells that have improperly been induced to proliferate (Vaseva & Moll, Citation2009; Andreopoulou et al., Citation2015). Zhai et al. (Citation2008) have shown that induced cell apoptosis by repressing bcl2 expression by GA. Low concentrations of GA have no obvious inhibitory effect on cell viability or apoptosis-inducing effect (Li et al., Citation2011). Moreover, we observed that in vitro treatment with GA significantly (p < 0.05) up-regulates the expression of the pro-apoptotic protein Bax and down-regulated the expression of the anti-apoptotic protein Bcl-2 in MDA-MB-231 cells at the protein level. It is not known whether GA affects other genes in addition to Bax and Bcl-2, how it affects, and also if other mechanisms may contribute to changes in cell apoptosis. This would like to explore in future in our laboratory.
Previous studies have demonstrated the anti-angiogenic potential of GA-free drug on HUVEC (Lu et al., Citation2013). There has been no study so far that evaluated the effect of GA loaded in cationic liposomes on HUVEC tube formation. GAL treatment showed linear structures of the network were significantly (p < 0.01) disrupted compared to GA-free drug treatment or control. GA-free drug and GAL exhibited anti-angiogenic activity by inhibition of tube formation compared to control (). In a similar study, Lu et al. (Citation2007) showed that GA has potential to inhibit angiogenesis in vitro and in vivo via suppressing phosphorylation of VEGFR2 selectively and reduction of the VEGF secretion from tumor cell. In our study, we observed a significantly potent inhibition in GAL-treated cells compared to GA-free drug. Our study demonstrates that GA and GAL directly reduce HUVEC proliferation and tube formation on matrigel. In the present study, we provide evidence for the first time that GAL has a potent anti-angiogenic activity. The dual anti-tumor and anti-angiogenic activities of GAL are consistent with those of some other conventional anticancer drugs such as docetaxel, paclitaxel and camptothecin, and thus suggest its potential use as anti-tumor agents as well as its efficacy to be included in tumor therapy (Patel et al., Citation2014).
Due to the observed in vitro anti-angiogenic and apoptotic activity, we were interested in evaluating the expression of microRNAs involved in the apoptosis. In this study, our microarray data analysis (data not shown) and validation by qRT-PCR revealed that miR-34b and 224 expressions positively correlates with the expression of the apoptotic genes Bcl2, p53 and Bax, which are known to induce apoptosis. But the strength of the conclusions of this study was hampered as these results are based on in vitro experiments. In order to assess the functional role of miR-34b and miR-224, further studies should be done using animal models. Also, studies of miR-34b and miR-224 with gene targets in TNBC are of further importance. Our study provided an addition confirmation that miR-34b has an anti-tumor function in TNBC. Findings from our study by both microarray and real-time PCR analysis indicated that these microRNAs act as tumor suppressors. In addition to miR-34b and miR-224, supporting our data, other miRs like miR-335, miR-206 and miR-126 have also been identified as human breast cancer metastasis suppressor miRs (Misso et al., Citation2014).
The anticancer effect of GAL may be induced by inhibiting angiogenesis and directly killing tumor cells. Our in vivo studies demonstrated that administration of the GAL did not lead to any abnormal behavioral changes in these animals, suggesting that the GA-free drug and nanosized assemblies of GAL are safe for parenteral administration. However, due to the presence of non-ionic surfactant in a free drug solution, administration in human may cause severe toxicities. In the in vivo study, Yao et al. (Citation2014) demonstrated that GA loaded hyaluronic acid nanoparticles exhibited therapeutic efficacy with reduced toxicity. Encouragingly, we also observed these findings in the GAL-treated group, suggesting that the GAL did not have toxicity in mice. In vivo anticancer efficacy study of intravenously administered GA on MDA-MB-231 bearing balb/c nude mice was also carried out in this study. However, no description of solubilizer or formulation was given in the report. We assume that author may have used ethanol and surfactant based formulation. In spite of injecting less number of cells (1.5 million/animal) compared to Li et al. (Citation2012) (2 million/animal), a significant difference in tumor progression was observed for GA in TNBC tumor bearing mice. In that study, average tumor volume in the animals of control group attained 2000 mm3 in 10 weeks while in our study the same volume was achieved within just 21 d. Further, the author showed that anticancer efficacy of GA was observed for 7 weeks after the first dose. It is noteworthy that in that report, animals were given about 25 doses of GA by IV route every other day. While in our study only three doses were administered every third day and the study was terminated after 21 d. In both the studies, cell line, type of animals and organ of injection are same, but tumor development and growth rate is very different. The possible reason for such variation could be the passage number of injected MDA-MB-231 cells. We have injected the cells which were freshly cultivated from tumor tissue of MDA-MB-231 tumor-bearing mice. Generally, tumor cells obtained by this method are very aggressive in proliferation and invasionConsidering the results of our study and previously reported studies on anticancer efficacy of GA against TNBC, we can conclude that liposomal formulation prepared in our laboratory has some salient advantages over solution-based formulation: (a) liposomal formulation requires significantly less number of doses for inhibiting tumor growth; (b) formulating cationic charged liposomes of GA could potentially helpful in targeting angiogenesis; (c) organic solvents and surfactants are not required to deliver GA.
One of the widely accepted mechanism of tumor development is apoptotic inhibition and to inhibit the carcinogenic process, numerous chemopreventive agents have been shown to act through the induction of apoptosis (Naithani et al., Citation2008). Our observations in the current study also support that GA-free drug and GAL act by the induction of apoptosis. We observed in the current study that GA-free drug and GAL treatment decreases the expression of survivin in breast tumors (). Survivin, an inhibitor of apoptosis protein, is highly expressed in most of the cancers and associated with chemotherapy resistance (Pereira & Amarante-Mendes, Citation2011). Therefore, our observed down-regulation of survivin expression results in activation of caspases and thereby induces apoptosis in tumor cells. Expression of p53 plays a vital role in regulating tumor cell response to drugs and modulate the effect of downstream effectors like Bax and p21 (Xu et al., Citation2013). In our study, found that Bax was activated in GA-free drug and GA cationic liposomes treatment group leading to apoptosis in breast tumors. Our observations were similar to Gu et al. (Citation2009) reported that GA in vitro (at a concentration of 4 μm) induces apoptosis of non-metastatic breast cancer MCF-7 cells by reducing Bcl2 expression via p53 in a time- and concentration-dependent fashion. To sum up, our findings in the current study suggests that mechanism of action of GA to induce apoptosis mediated via downregulation of antiapoptotic Bcl2 and upregulation of proapoptotic Bax. Our immunohistochemical analysis also found that the expression of CD31 was decreased in GAL group compared to GA-free drug and control groups which clearly indicated that microvessel density and neovascularization was significantly affected by GAL treatment. The superior antitumor activity of GAL was expected to majorly contribute by its preferential interaction and consequent disruption of neovasculature.
Conclusion
Solvent and non-ionic surfactant-free delivery system of GA was developed for parenteral administration. Cationic PEGylated liposome enhanced the anti-angiogenic efficacy of GA by facilitating preferential interaction with endothelial cells of tumor neovasculature. Significant reduction in tumor volume and alteration in apoptosis and angiogenic markers were attributed to enhanced delivery of GA to tumor tissues due to long circulation and positive surface charge of liposomes. Similar to TNBC, GA-loaded cationic PEGylated liposome could be useful in the treatment of other solid tumor with extensive angiogenesis.
Declaration of interest
This research was supported with funding from the National Institutes of Health’s Minority Biomedical Research Support (MBRS)-SC1 program [Grant # SC1 GM092779-01]; the National Institute on Minority Health and Health Disparities (NIMHD) P20 program [Grant # 1P20 MD006738-03] and the Department of Defense (DOD) Breast Cancer Program [Grant # W81XWH-11-1-0211].
References
- Adlakha-Hutcheon G, Bally MB, Shew CR, Madden TD. (1999). Controlled destabilization of a liposomal drug delivery system enhances mitoxantrone antitumor activity. Nat Biotechnol 17:775–9
- André F, Zielinski CC. (2012). Optimal strategies for the treatment of metastatic triple-negative breast cancer with currently approved agents. Ann Oncol 23:vi46–51
- Andreopoulou E, Schweber SJ, Sparano JA, McDaid HM. (2015). Therapies for triple negative breast cancer. Expert Opin Pharmacother 16:983–98
- Bonotto M, Gerratana L, Poletto E, et al. (2014). Measures of outcome in metastatic breast cancer: insights from a real-world scenario. Oncologist 19:608–15
- Cai L, Qiu N, Xiang M, et al. (2014). Improving aqueous solubility and antitumor effects by nanosized gambogic acid-mPEG2000 micelles. Int J Nanomedicine 9:243–55
- Chang HI, Yeh MK. (2012). Clinical development of liposome-based drugs: formulation, characterization, and therapeutic efficacy. Int J Nanomedicine 7:49–60
- Deshpande PP, Biswas S, Torchilin VP. (2013). Current trends in the use of liposomes for tumor targeting. Nanomedicine (Lond) 8:1509–28
- Doddapaneni R, Chawla YK, Das A, et al. (2013). Overexpression of microRNA-122 enhances in vitro hepatic differentiation of fetal liver-derived stem/progenitor cells. J Cell Biochem 114:1575–83
- Godugu C, Patel AR, Doddapaneni R, et al. (2014). Approaches to improve the oral bioavailability and effects of novel anticancer drugs berberine and betulinic acid. PLoS One 9:e89919
- Gu H, Rao S, Zhao J, et al. (2009). Gambogic acid reduced bcl-2 expression via p53 in human breast MCF-7 cancer cells. J Cancer Res Clin Oncol 135:1777–82
- Immordino ML, Dosio F, Cattel L. (2006). Stealth liposomes: review of the basic science, rationale, and clinical applications, existing and potential. Int J Nanomedicine 1:297–315
- Li C, Lu N, Qi Q, et al. (2011). Gambogic acid inhibits tumor cell adhesion by suppressing integrin β1 and membrane lipid rafts-associated integrin signaling pathway. Biochem Pharmacol 82:1873–83
- Li C, Qi Q, Lu N, et al. (2012). Gambogic acid promotes apoptosis and resistance to metastatic potential in MDA-MB-231 human breast carcinoma cells. Biochem Cell Biol 90:718–30
- Liu YT, Hao K, Liu XQ, Wang GJ. (2006). Metabolism and metabolic inhibition of gambogic acid in rat liver microsomes. Acta Pharmacol Sin 27:1253–8
- Lu N, Hui H, Yang H, et al. (2013). Gambogic acid inhibits angiogenesis through inhibiting PHD2-VHL-HIF-1α pathway. Eur J Pharm Sci 49:220–6
- Lu N, Yang Y, You QD, et al. (2007). Gambogic acid inhibits angiogenesis through suppressing vascular endothelial growth factor-induced tyrosine phosphorylation of KDR/Flk-1. Cancer Lett 258:80–9
- Misso G, Di Martino MT, De Rosa G, et al. (2014). Mir-34: a new weapon against cancer? Mol Ther Nucleic Acids 3:e194. doi: 10.1038/mtna.2014.47
- Naithani R, Huma LC, Moriarty RM, et al. (2008). Comprehensive review of cancer chemopreventive agents evaluated in experimental carcinogenesis models and clinical trials. Curr Med Chem 15:1044–71
- Palmer JR, Viscidi E, Troester MA, et al. (2014). Parity, lactation, and breast cancer subtypes in African American women: results from the AMBER Consortium. J Natl Cancer Inst 106:dju237. doi: 10.1093/jnci/dju237
- Patel K, Patil A, Mehta M, et al. (2013). Medium chain triglyceride (MCT) rich, paclitaxel loaded self nanoemulsifying preconcentrate (PSNP): a safe and efficacious alternative to Taxol. J Biomed Nanotechnol 9:1996–2006
- Patel K, Patil A, Mehta M, et al. (2014). Oral delivery of paclitaxel nanocrystal (PNC) with a dual Pgp-CYP3A4 inhibitor: preparation, characterization and antitumor activity. Int J Pharm 472:214–23
- Pereira WO, Amarante-Mendes GP. (2011). Apoptosis: a programme of cell death or cell disposal? Scand J Immunol 73:401–7
- Qi Q, Gu H, Yang Y, et al. (2008a). Involvement of matrix metalloproteinase 2 and 9 in gambogic acid induced suppression of MDA-MB-435 human breast carcinoma cell lung metastasis. J Mol Med (Berl) 86:1367–77
- Qi Q, You Q, Gu H, et al. (2008b). Studies on the toxicity of gambogic acid in rats. J Ethnopharmacol 117:433–8
- Sawant RR, Torchilin VP. (2012). Challenges in development of targeted liposomal therapeutics. AAPS J 14:303–15
- Saxena V, Hussain MD. (2012). Poloxamer 407/TPGS mixed micelles for delivery of gambogic acid to breast and multidrug-resistant cancer. Int J Nanomedicine 7:713–21
- Tao Z, Shi A, Lu C, et al. (2014). Breast cancer: epidemiology and etiology. Cell Biochem Biophys 72:333–8
- Vaseva AV, Moll UM. (2009). The mitochondrial p53 pathway. Biochim Biophys Acta 1787:414–20
- Wang X, Chen W. (2012). Gambogic acid is a novel anti-cancer agent that inhibits cell proliferation, angiogenesis and metastasis. Anticancer Agents Med Chem 12:994–1000
- Xing M, Yan F, Yu S, Shen P. (2015). Efficacy and cardiotoxicity of liposomal doxorubicin-based chemotherapy in advanced breast cancer: a meta-analysis of ten randomized controlled trials. PLoS One 10:e0133569. doi: 10.1371/journal.pone.0133569
- Xu J, Zhou M, Ouyang J, et al. (2013). Gambogic acid induces mitochondria-dependent apoptosis by modulation of Bcl-2 and Bax in mantle cell lymphoma JeKo-1 cells. Chin J Cancer Res 25:183–91
- Yao J, Li Y, Sun X, et al. (2014). Nanoparticle delivery and combination therapy of gambogic acid and all-trans retinoic acid. Int J Nanomedicine 9:3313–24
- Zhai D, Jin C, Shiau CW, et al. (2008). Gambogic acid is an antagonist of antiapoptotic Bcl-2 family proteins. Mol Cancer Ther 7:1639–46
- Zou ZY, Wei J, Li XL, et al. (2012). Enhancement of anticancer efficacy of chemotherapeutics by gambogic acid against gastric cancer cells. Cancer Biother Radiopharm 27:299–306