Abstract
Context: As the first-line agent for genital warts, podophyllotoxin (POD) could induce extensively skin burning, itching, and erythema. Meanwhile, as a common anti-inflammatory agent, glycyrrhizic acid (GA), also has amphipathic and solubilizing properties, indicating that it might be a promising drug carrier.
Objective: The objective of this study is to formulate and characterize the POD-loaded GA micelles preparation and to evaluate its drug release characteristics and anti-inflammatory properties.
Materials and methods: The novel micelles preparation was prepared by ultrasonic dispersion method and characterized using different scanning calorimetries, dynamic light scattering, and transmission electron microscopies. Subsequently, its encapsulation efficiency (EE), drug-loading content (LC), in vitro skin permeation, in vivo drug retention, and distribution of POD were detected. The anti-inflammatory effect of the preparation was reflected by HE staining and immunohistochemistry in the rat skin.
Results: The POD-loaded GA micelles formed spherical shapes (approximately 10 nm) with an EE of 78.53 ± 2.17% and a LC of 7.293 ± 0.42%. Meaningfully, unlike the extensive distribution of the POD tincture throughout the skin tissue, POD released from the POD-loaded GA micelles mainly located in the epidermis and could maintain steady skin retention for 12 h. Moreover, the POD-loaded GA micelles induced less leukocyte infiltration and inflammatory factors (IL-6 and TNF-α) expression when compared with the POD tincture.
Discussion and conclusion: Our results suggested that the POD-loaded GA micelles could achieve a higher POD distribution in the epidermal layer as well as a lighter skin inflammation. This new POD delivery system might be a potential and promising candidate for genital warts and deserved further researches.
Introduction
Genital warts are caused by the human papillomavirus (HPV) 6 or 11 viruses. The virus penetrates the skin or mucosa, affecting epithelial basal layers cells (Burd, Citation2003) in the cervix, vagina, urethra, and perianal skin. Podophyllotoxin (POD), a purified form isolated from resin of podophyllum (D. Versipoellis Hance M. Cheng) rhizome (Gordaliza et al., Citation2004), has been commonly used as the first-line agent in the treatment of genital warts (Syed et al., Citation1995; del Rio et al., Citation2012). In the clinic, POD is used in the form of POD tincture (5 mg/mL). However, the side effects of POD tincture are serious and common. Most patients using POD tincture bear serious side effects of POD including burning, skin irritation, itching, erythema or erosions (Hermanns-Le et al., Citation1998; Lacey et al., Citation2003, Citation2013). Thus, reducing the side effects of POD during its clinical use is very important and meaningful for patients of genital warts. Loading medicine in carrier materials is an effective way to attenuate the side effects of medicine. In recent decades, POD has been encapsuled by many types of carriers, such as stearic acid lipid nanoparticles (Xie et al., Citation2005), layered double hydroxide (LDHs) (Qin et al., Citation2010), poly-D, L-lactide nanoparticles (PLA NPs) (Yadav et al., Citation2014), and polyethylene glycol (PEG) (Roy et al., Citation2015). However, none of these POD preparations have been approved mainly due to the instabilities and unclear safeties of these formulations.
Glycyrrhizic acid (GA), the major active constituent of Licorice roots (Glycyrrhiza glabra L), has been used as an antidote (Lu et al., Citation2008; Tu et al., Citation2010) and demulcent in traditional Chinese medicine. GA is well known for its anti-inflammatory (Kao et al., Citation2010; Wang et al., Citation2011), anti-viral (Xie et al., Citation2011), and organ-protecting activities (Bi et al., Citation2009; Kao et al., Citation2009). GA is also proven to be effective and safe during application, and has been used in cosmetic products as flavoring or skin-conditioning agents (Cosmetic Ingredient Review Expert P, Citation2007). As shown in , GA is a type of triterpene saponin that contains both a hydrophobic region (one molecule of glycyrrhetinic acid) and a hydrophilic region (two molecules of glucuronic acid). In terms of structure, GA may form micelles in aqueous solutions. As encapsulating toxic drugs into carriers to form clathrate remains an effective way to reduce the side effects of them, we wonder whether POD could be encapsulated by GA to prepare POD-loaded GA micelles. If so, whether could this new POD delivery system attenuate the side effects of POD? It has been reported that GA could form the “host-guest” type (Polyakov et al., Citation2006) with some medicines such as nifedipine (Tolstikova et al., Citation2010), buspirone (Fomina et al., Citation2014), carotenoids (Polyakov et al., Citation2006), simvastatin (Vavilin et al., Citation2008), and lappaconitine (Polyakov et al., Citation2005), of which GA is the host while the medicine is the guest. Interestingly, it was demonstrated that applications of GA together with these medicines could strengthen their therapeutic actions (Selyutina et al., Citation2015) and reduce their side effects. Meanwhile, considering that POD has poor aqueous solubility, we wonder whether the addition of GA as the carrier could increase the solubility of POD and produce its therapeutic action.
To sum up, we speculated that the combination of POD and GA could form POD-loaded GA micelles, and the new formulation have therapeutic effect and may attenuate the side effects of POD. To verify this speculation, POD-loaded GA micelles were prepared and characterized. Subsequently, the attenuation effect of the new POD formulation on the side effects caused by POD was evaluated by the in vitro and in vivo assays. The aim of the present investigation was to form and evaluate the POD-loaded micelles preparation which using GA as a novel carrier. Compared with the POD tincture, the skin transportation characteristics and the inflamed skin improvements of the micelles preparation have also been studied.
Materials and methods
Materials
Podophyllotoxin standard drug (purity ≥ 98%, HPLC grade, lot number: 123309423007203) was obtained from Fluka Biochemika Co. (Buchs, Switzerland). Podophyllotoxin (purity ≥ 98%, HPLC grade, lot number: J1208009) was purchased from Aladdin Industrial Co., Ltd (Shanghai, China). Glycyrrhizic acid (purity ≥ 98%, lot number: O0615A) was from Meilun Biology Technology Co., Ltd. (Dalian, China). The podophyllotoxin tincture (5 mg/mL in 75% ethanol) was prepared based on the formula. Solvents, such as ethanol and methanol, were obtained from Merck Co. (Darmstadt, Germany). Distilled water was purified using a Milli-Q system (Millipore, Direct-Q, Millipore Corporation, Billerica, MA).
Animals
Male SD rats weighing about approximately 200 g were purchased from the Center of Experimental Animals, Southern Medical University (Guangzhou, China). All the animal experiments were performed according to the guidelines of the Experimental Animal Ethics Committee of Southern Medical University, and the protocol was approved (Permit number for rats: 44002100004873). All surgeries were performed using 10% chloral hydrate anesthesia.
Solubility studies
POD is a poorly water-soluble drug and is slightly soluble in ethanol, so the preparation available in the market contains high ethanol content. We quantitatively tested the POD solubility in ethanol. POD, in amounts that exceeded its solubility, was added to vials to which an ethanol/water mixture at different volume ratios was added. These vials were sealed and shaken in a VorTemp 56tm shaking incubator (Labnet International Inc., Edison, NJ) at 25 °C for 48 h to achieve solubility equilibrium. The samples of POD in the pure water, GA solution, combination solution of GA and ethanol were tested (the GA/POD was their weight ratios) and then filtered through 0.45-μm filters. The concentrations were detected by high performance liquid chromatography (LC-20A, Shimadzu, Tokyo, Japan).
Preparation of the POD-loaded GA micelles
The preparation of the POD-loaded GA micelles followed the ultrasonic dispersion method (Yang et al., Citation2015). GA was dissolved in preheated distilled water, and a solution of POD in ethanol was added (the weight ratio of POD: GA was 1:8) dropwise into the preheated aqueous GA solution at 60 °C. The resulting solutions contained 5 mg/mL POD, 40 mg/mL GA, and 25% (v/v) ethanol. The mixtures were incubation at 60 °C for 1 h by soaking them in a water bath to form micelles self-assembly, POD was loaded into the hydrophobic core of the micelles simultaneously. After the preparation were slowly cooled to room temperature, the obtained preparation were further dispersed using ultrasonic homogenizer with the amplitude set at 40% of the maximum for 30 min, and the stable POD-loaded GA micelle solution was made.
Differential scanning calorimetry measurement (DSC)
DSC analysis was carried out using TA Instruments (Q10DSC, New Castle, DE). Samples (5 mg), including the pure POD, pure GA, POD-GA physical complexes (the ratio of GA/POD was 8–1) and POD-loaded GA micelles, were weighed into aluminum pans. The samples were tested under a nitrogen atmosphere (40 mL/min) and heated in the range of 25–250 °C at a heating rate of 10 °C/min. Lyophilized powder of the POD-loaded GA micelles preparation was prepared for the DSC measurement and drug-loading contents analysis. The prepared micelle solution was frozen at −70 °C for 24 h in an ultra-low temperature freezer. The frozen samples were dried in a freeze dryer (SIM Corp., New York, NY) for 30, and then the sample powders were obtained.
High-performance liquid chromatography (HPLC) analysis of POD
HPLC was used to determine the amounts of POD in the preparation and tested samples. The analysis was performed using a Shimadzu High Performance Liquid Chromatograph (LC-20A, Shimadzu, Tokyo, Japan). A C18 column (5 μm, 100 nm × 4.6 nm) was used and maintained at 30 °C. The mobile phase was composed of methanol and water (63:37, v/v) at a flow rate of 1 mL/min. The injection volume was 20 μL, and the detection wavelength was 292 nm. The retention time was approximately 4.8 min. The recovery rate ranged from 98.5 to 100.5%, and the accuracy and precision values were always below 5%. The concentration range of 1.17–150 μg/mL (r > 0.999) was used for detecting the POD formulations. All of the samples were filtered through a 0.45-μm organic membrane filter before being detected. This method was specific because no interference from the formulation and skin factors was observed (.
Critical micelle concentration (CMC) of GA
The CMC value of GA was determined using a DDS-11A electrical conductivity meter (Condicto Metermodec, Tokyo, China). The GA aqueous solution CMC values at different ethanol concentrations were obtained from the breakpoint of the conductivity versus the concentration plot.
Micelle size analysis and morphology
The drug micelles average particle size (z-average size), polydispersity index (PDI), and size distribution were determined via dynamic light scattering (DLS) using a Malvern Zetasizer 3000HSA (Malvern, Worcs, UK). Morphological analyze of the micelles were carried out by transmission electron microscopy (TEM) using a JEOL-100CXII TEM (JEOL Inc., Tokyo, Japan). A drop of freshly prepared micelle solution, which had been diluted five times, was stratified onto a 200 mesh carbon-coated copper grid and left to adhere to the carbon substrate for approximately 1 min. The excess solution was removed by a piece of filter paper. Then the sample was stained by 2% (w/v) phosphotungstic acid and left to stand for 1 min. The excess staining agent was removed with filter paper and air-dried at room temperature.
Entrapment efficiency (EE) and drug loading contents (LC) analysis
Entrapped POD was removed from free POD using an ultrafiltration method. The micelles were isolated by ultrafiltration filters (Microcon, Millipore Corporation, Billerica, MA) using YM-3 membranes with a 3-kDa molecular mass cutoff and then centrifuged using a TGL-16B Centrifuge (Shanghai, Tokyo, China) for 15 min at 4000 rpm. Then, the free POD supernatant in the ultrafiltrate collection container was removed to determine the concentration by HPLC. Drug loading was evaluated using a direct method. Three milligrams of the lyophilized micelle powder was dissolved in 50 mL of methanol by sonication (SB25-12YDTD, Scientz, China) for 1 h to fully release the POD. One milliliter of the testing solution was then filtered through a 0.45-μm organic membrane filter before being detected by HPLC. The drug entrapment efficiency (EE) and drug loading contents (LC) were calculated using the following equations. Winitial drug is the amount of drug added in the formulation. Wfree drug is the amount of drug in the supernatant. Wactual drug is the amount of drug loaded in micelles. Wtotal drug is the amount of total micelles.
(1)
(2)
Stability
The POD-loaded GA micelles preparation (0.5%, w/v) were stored in 10-mL Eppendorf tubes at room temperature. Samples were transferred into a clear eppendorf tube for visual observation, and the particle size, clarity, entrapment efficiency, and drug loading contents were monitored after 0, 1, and 3 months. The experiments were performed in three replicates.
The permeation studies were carried out in vertical Franz diffusion cells for 12 h through rat skin. The receptor compartment was filled with 7 mL of receptor medium (ethanol-water 1:9, v/v), and the effective diffusion area was 3.14 cm2.
Abdominal skin was surgically excised after shaving the hair from anesthetized SD rats. The integral skin was then rinsed with physiological saline. The stratum corneum faced the donor compartment, while the dermis faced the receptor. The diffusion cells were maintained at 37 °C using a re-circulating water bath, and the fluid in the receptor chambers was stirred at 300 rpm throughout the entire experiment. To simulate the real clinical treatment condition, the respective 0.5 mL formulations (0.5% POD tincture, 0.5% POD-loaded GA micelles preparation, 0.5% POD tincture include 40 mg/mL GA) were applied on the skin surface in the donor chambers without a parafilm covering. One millilitre of samples from the receiver were withdrawn at predetermined time intervals (1, 2, 4, 6, 8, and 12 h) and replaced with an equivalent volume of fresh receptor solution. The collected samples were filtered through aqueous 0.45 μm filters and analyzed by HPLC as previously described. The permeated amounts of POD through the skin were plotted against time. The POD steady–state flux (Jss, μg/cm2/h) across the skin was calculated by dividing the slope of the linear portion of the permeated cumulative amounts curve.
The in vitro release characteristics of the POD-loaded GA micelles were investigated using the dialysis method (Hazzah et al., Citation2015). Briefly, POD-loaded GA micelles solution (1 mL) containing 5 mg/mL POD was put into the swelled dialysis bag with a molecular weight cut off of 5000 Da. Then, the dialysis bag was tied and immersed into 100 mL release medium (PBS solution supplemented with 0.5% Tween-80). The paddle speed was set at 100 rpm. The bath temperature was set at 37 ± 0.5 °C. The release medium (0.5 mL) was withdrawn at different time intervals (10 min, 0.5 h, 1 h, 1.5 h, 2 h, 3 h, 4 h, 5 h, 6 h, 8 h, 10 h, and 12 h) and replaced with an equivalent volume of a previously warmed fresh medium. The POD samples were filtered using 0.22-μm filters and analyzed by HPLC method. Each experiment was performed in triplicate.
In vivo drug-deposition study and skin distribution
POD formulation samples were applied to the anesthetized rats. The drug application dosage (0.796 mg/cm2) was strictly controlled and was the equivalent of the dose in the in-vitro experiments. Skin samples in anesthetized rats to which POD-loaded GA micelles formulation or the POD tincture (1, 3, and 5 mg/mL) were applied were dismantled and evaluated at each predetermined time interval (2, 4, 8, and 12 h). After being rinsed with normal saline, the skin was cut into small 1 cm2 pieces. POD was extracted from the skin layers by homogenization in 1.5 mL of methanol followed by centrifugation (15 000 rpm; 30 min). The supernatants were then collected and analyzed using HPLC.
POD has fluorescent activity, with maximum excitation and emission wavelengths of 292 nm and 329 nm, respectively. The deposited drug in the skin slice was observed by fluorescence microscopy (Olympus DP71, Tokyo, Japan). The skin samples treated with the GA solution, POD tincture, and POD-loaded GA micelles formulation from in vivo permeation experiments (12 h) were rinsed by normal saline. Samples were frozen at −20 °C and fixed by a viscous gel (OPT). Slices were then cut using a freezing microtome (LEICA CM1850 UV, Nussloch, Germany). Horizontal sections were obtained so the fluorescence microscopy could be used for visualizing the distribution of POD.
HE staining and immunohistochemistry
HE staining and immunohistochemistry were carried out as previously described (Wang et al., Citation2015). Briefly, rats were divided into four groups, and formulation samples were applied twice daily for 3 d, which simulated the dosage and course of the clinical treatment (Lacey et al., Citation2003). The samples were physiological saline, 75% ethanol, POD tincture, and POD-loaded GA micelles formulation. The skin grafts were harvested and processed by 4% paraformaldehyde fixation and paraffin embedment (LEICA EG1160, Nussloch, Germany). Samples were cuts into 3-μm sections using a slicer (LEICA RM2235, Nussloch, Germany) and stained with hematoxylin and eosin (HE) for cutaneous histomorphological observation. The paraffin sections were deparaffinized and hydrated, then washed with PBS containing 1% bovine serum albumin. Background staining was blocked with powdered skim milk. Sections were incubated overnight at 4 °C with anti-TNF-α (Santa Cruz Biotechnology, Santa Cruz, CA) or anti-IL-6 (Bioworld Technology, Inc. St Louis Park, MN) and then washed three times for 5 min each in PBS plus 1% BSA. Samples were then incubated at room temperature for 45 min with a biotinylated antibody. Sections were incubated with the AB complex (Vector Laboratories, Burlingame, CA) for 45 min and then washed again, and the reaction was revealed by DAB (Sigma-Aldrich, St. Louis, MO) and counterstained with Mayer’s hematoxylin. The immunohistochemical assays were performed to test the expression of the inflammatory factors TNF-alpha and IL-6 in the treated skin. Microscopy (Olympus DP71, Tokyo, Japan) was used to observe the differences in these groups.
Statistical analysis
All the results are presented as the arithmetic mean values ± standard deviation (SD) of at least three replicates (n = 3). The treated groups were compared with the control groups by repeated measures analysis of variance, following a Fisher's Least Significant Difference (LSD). p value < 0.05 was considered significant. The software GraphPad Prism 5 (GraphPad Software, San Diego, CA) was used for data analysis.
Results
Solubility studies
The solubility diagram of POD with the GA solution is presented in . The plot () showed that the poor solubility of POD in water was 0.104 mg/mL. However, its solubility could be increased dramatically in ethanol aqueous solution as the proportion of ethanol rose. When the ethanol proportion was around 40% (v/v), the solubility of POD could reach 5 mg/mL (equaling to the traditional POD tincture used in the clinic). Considering that the concentration of POD used in the clinic is 5 mg/mL, the dosage was chosen to investigate the solubilizing effect of GA on POD in different proportions of ethanol aqueous solution. It is clearly observed in that the drug solubility in the ethanol/water solution increased greatly as GA was added. The POD concentration of the samples in 25% ethanol without GA was 1.060 ± 0.039 mg/mL, the concentration of the formulation reached 5 mg/mL with a high entrapment efficiency () when the GA/POD ratio up to 8:1. The POD concentration achieved 5 mg/mL when the samples’ GA/POD ratio was higher than 8:1 and the ethanol content higher than 25%. At this ratios condition, the micelles solution could be formed. To sum up, these results indicated that POD was poorly water soluble, while its solubility could be increased by adding GA into ethanol aqueous solution.
Figure 3. (A) Phase-solubility diagram of the POD solution at different ethanol–water ratios (0%, 10%, 20%, 25%, 30%, 35%, and 40%); (B) influence of the GA/POD ratios and ethanol contents (0%, 15%, 20%, 25%, and 30%) on the solubility of POD. (C) Influence of different GA/POD ratios on the encapsulation efficiency of POD as the ethanol content was set at 25% (v/v); Data are expressed as the mean ± SD (n = 3).
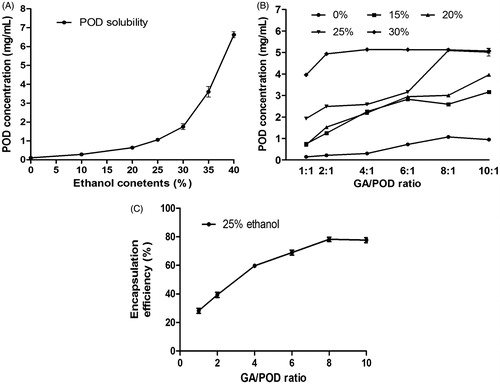
Differential scanning calorimetry measurement (DSC)
shows the DSC thermograms of the pure POD, pure GA, POD-GA physical complexes, and POD-loaded GA micelles. The physical complexes were formulated with the same composition and ratio of the micelles formulation, and two endothermic melting peaks of pure POD (approximately 180 °C) and pure GA (approximately 200 °C) were observed, while the height of the endotherm in the thermal scan of POD-loaded GA micelles showed no peaks. These results suggested that most of the POD was encapsulated by GA micelles in our prepared POD-loaded GA micelles.
Critical micelle concentration (CMC) of GA
As a saponin, GA has a series of CMCs in different solutions. POD-loaded GA micelles could only be formed when the concentration of GA achieved its CMC. The CMCs of GA in different proportions of ethanol aqueous solutions were determined by detecting the specific conductivity changes of the GA solutions. The results are displayed in , the intersection concentration (CMC) of the two fitted curves were 1.20 mM for the 0% ethanol group, 0.97 mM for the 15% ethanol group, 0.28 mM for the 25% ethanol group, 0.16 mM for the 35% ethanol group, and 0.06 mM for the 55% ethanol group. The CMCs of the GA solution decreased as the proportion of ethanol in the GA solutions increased.
Micelle size analysis and morphology
The diameters of micelles were measured by Dynamic Light Scattering (DLS). As shown in ) and , the average particle sizes of the blank micelles and the drug loaded micelles were approximately 6 nm and 10 nm, respectively. Compared with the blank micelles, the drug-loaded micelles had a larger size. In addition, only one peak was observed on the DLS diagram of POD-loaded GA micelles, and the polydispersity index (PDI) was lower than 0.5, indicating the uniform size and homogeneity of the micelles. Transmission electron microscopy (TEM) was used to observe the morphology of the micelles. As shown in , the drug-loaded GA micelles were spherical in shape with a narrow size distribution, indicating the homogeneity of these micelles.
Figure 6. Dynamic light scattering results of the POD-loaded GA micelles (A) and blank micelles (B); TEM images of the POD-loaded GA micelles preparation (×20 k and ×50 k) (C).
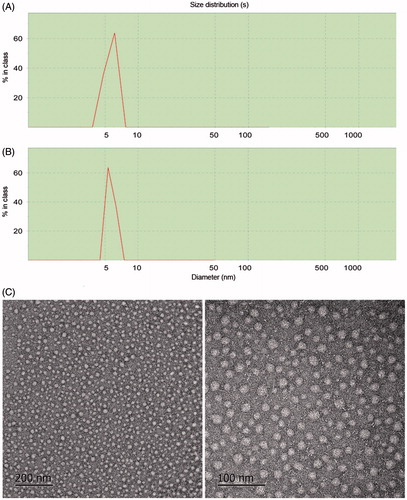
Table 1. The characteristic parameters of the POD-loaded GA micelles.
Entrapment efficiency, drug-loading contents, and stability
The final preparation’s entrapment efficiency and its drug loading contents were calculated according to EquationEquations (1)(1) and Equation(2)
(2) . The POD-loaded GA micelles had a relatively high entrapment efficiency of 78.53 ± 2.17% and a drug-loading contents of 7.293 ± 0.42%. In addition, the POD-loaded GA micelles were stable during storage at room temperature for 3 months. As shown in , no obvious changes in clarity and degradation were observed. The size of the micelles only slightly increased after storing for 3 months (10.83 nm versus 10 nm). The HPLC results also suggested that the concentration of POD in the micelles was stable.
In vitro skin permeation study and in vitro release assay
In formulations applied to the skin at room temperature, the in vitro release of the three types of POD samples is shown in . The cumulative penetration amounts of POD released form the POD-loaded GA micelles formulation and POD tincture added with GA included were lower than that of the POD tincture within 12 h. The lag time of the POD tincture, the POD-loaded GA micelles formulation, and the POD tincture (including GA) were 1.251 h, 2.06 h and 0.836 h, respectively. The steady-state flux (Jss) showed that the micelles could slow down the releasing rate of POD (). The release characteristics of POD from the POD-loaded GA micelles were consistent with zero kinetics (R2 = 0.9868). The in vitro release assay manifested that POD kept sustained release from the POD-loaded GA micelles in the first 5 h (). Thus, instead of the immediate release mode of the POD tincture solution, sustained release of the POD-loaded GA micelles could prolong drug action time. Moreover, these results indicated that the POD-loaded GA micelles formulation could slow down the transdermal permeation rate and decrease the penetration amounts of POD through skin layers.
Figure 7. (A) In vitro skin permeation studies of the skin applied with POD tincture, POD-loaded GA micelles preparation, and POD tincture (included GA). The POD concentration of these samples was 5 mg/mL (n = 5); (B) In vitro release profiles of the POD-loaded GA micelles in PBS at 37 ± 0.5 °C (n = 3); (C) In vivo drug-deposition studies of the skin applied with the POD-loaded GA micelles preparation (5 mg/mL) and the POD tincture of different drug concentrations (1, 3, and 5 mg/mL); fluorescent images of the rat skin treated with the GA solution (D), POD tincture (E), and POD-loaded GA micelles preparation (F).
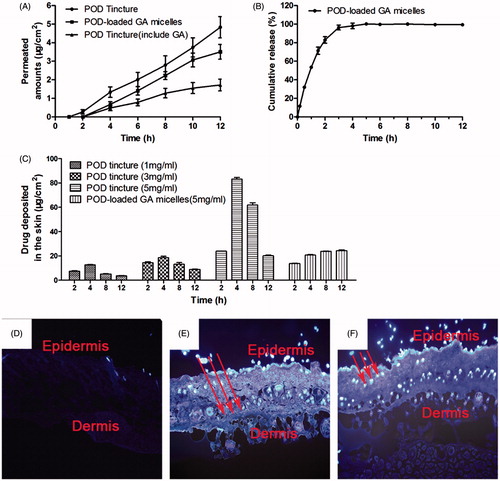
Table 2. The in vitro skin permeation study.
In vivo drug-deposition study and skin distribution
Skin samples for the analysis of the POD retention were from the anesthetized rats in the in vivo study which could be more factually. The amounts of POD deposited in the skin ( ) were from different formulations at different time intervals. As POD was released sustainably from the POD-loaded GA micelles, the POD retention amount in the skin was maintained at a stable level. As shown in , the skin deposition amounts of POD in four different concentrations of POD tincture groups (1, 3, and 5 mg/mL) all peaked at 4 h later after POD tincture administration, and decreased sharply in the following 4 h. Meanwhile, the skin deposition amounts of POD in the POD-loaded GA micelles group increased continuously during 12 h administration. More significantly, the final skin deposition amount of POD for the POD-loaded GA micelles group was higher than that for the POD tincture group at 12 h interval (24.37 ± 0.97 μg/cm2 versus 20.07 ± 1.02 μg/cm2).
Table 3. The amount of drug deposited in skin (μg/cm2).
In agreement with the drug retention observation, ) depicts the fluorescent images of the skin sections. The skin treated with GA () had no fluorescence under the fluorescent microscope as GA was not a fluorescent agent. The fluorescence of the skin treated with the POD tincture () distributed both in the epidermis and in the dermis region. More meaningfully, the fluorescence of the skin treated with the POD-loaded GA micelles () mainly distributed in the epidermis, which was the desired location and beneficial to the treatment of genital warts.
HE staining and immunohistochemistry
HE staining and immunohistochemistry (IHC) were carried out to observe the influence of the POD-loaded GA micelles on the skin inflammation caused by POD. As shown in (HE), the POD tincture caused an intense inflammatory reaction in the skin, including edema and leukocyte infiltration. The skin treated with ethanol had changes in the anatomical structure, which showed skin wrinkling and enlarged pores. These results demonstrated that high concentrations of ethanol could damage the structure of the skin and thus allow the POD tincture to penetrate into deeper skin layers. However, few changes of the anatomical structure and leukocyte infiltration were observed in the skin treated with the POD-loaded GA micelles. Few changes were observed in the anatomical structure and leukocyte infiltration in the skin treated with the POD-loaded GA micelles formulation in comparison with the control-untreated skin. The inflammatory leukocytes shown in were mainly secreted from the stratum basale and sebaceous gland. The POD-loaded GA micelles formulation induced less expression of TNF-alpha and IL-6 in the treated skin compared with the POD tincture.
Discussion
The POD tincture used in the clinic usually causes side effects such as burning, skin irritation and erythema. Encapsulating toxic drugs into carriers to form clathrate remains an effective way to reduce the side effects of them. As GA had amphipathic structures, we speculated that GA could form micelles at a concentration above its CMC. The DSC, TEM, and DLS were carried out to verify the formation of the POD-loaded GA micelles.
GA has been reported to have an influence on drug permeation in the skin (Sapra et al., Citation2008), and it has also been studied as a paclitaxel micelle carrier in our research group (Yang et al., Citation2015). In the present study, GA’s solubilization property improved the solubility of POD and the formulation could reach a concentration of 5 mg/mL. The solubility study showed that GA significantly improved the solubility of POD. The concentration of POD in the POD-loaded GA micelles could reach 5 mg/mL, which was equal to that of the POD tincture (5 mg/mL) used in the clinic. Furthermore, compared with the traditional POD tincture, the ethanol content of the POD-loaded GA micelles formulation was relatively lower (25% versus 75%). The lower ethanol content would bring a less POD distribution in the skin dermis as well as a less POD absorption in the blood (Longstaff & von Krogh, Citation2001), which meant less side effects of the new POD delivery system.
The DSC measurement gave an insight into the phase transition in POD-loaded GA micelles, which are responsible for the change in the physical state. The DSC data clearly suggested that POD was encapsulated in the GA micelles. These results were further confirmed by the TEM study.
The critical micelle concentration (CMC) was defined as the concentration at which micelles are formed. CMC is an important parameter to determine formulation stability, i.e., a lower CMC value would be more stable (Dahmani et al., Citation2012). At a concentration over the CMC, the GA in solution was in the form of micelles that might enhance the solubility of a poorly soluble drug. The contents of GA in the POD-loaded GA micelles exceeded its CMC, which means that GA formed micelles in the preparation solution. Ethanol in the formulation was characterized as a structure-creating solvent (Tolstikova et al., Citation2009).
The average diameter and the polydispersity index (PDI) obtained from the DLS measurement show the uniform size and homogeneity of the micelles. The TEM image shows that the POD-loaded GA micelles could finely form spherical shapes with good stability. It implies that transition of the dispersed micelles in formulation changed slowly because of their small size.
The in vitro skin permeation study suggested that the lag time of the POD-loaded GA micelles was longer than that of the POD tincture. This may result from the POD interaction pattern differences between them. POD kept sustained release from the POD-loaded GA micelles in the first 5 h of the in vitro release assay. The sustained release of the POD-loaded GA micelles was different from the immediate release mode of the POD tincture liquid. POD was released from the POD-loaded GA micelles’ hydrophobic cores first instead of interacting with the skin directly. Meanwhile, the in vitro skin permeation study also indicated that the release characteristics of POD from the POD-loaded GA micelles were consistent with zero-order desorption (Agren & Elofsson, Citation1967). Therefore, the POD concentration on the receiver side as well as the steady-state flux of POD was relatively constant. In addition, as a result of the lower penetration rate and the reduced cumulative penetration amount of POD, the POD amount penetrated into deeper skin layer reduced.
The high ethanol contents of the POD tincture permeate into the skin and alter the solubility properties of the tissue with an improvement of drug permeation into the skin (Megrab et al., Citation1995). Therefore, it is reasonable that the clinically used POD tincture is largely and rapidly distributed into both epidermis and dermis, and even absorbed in the blood (Chen et al., Citation2006). In the present study, it was also clearly observed under the fluorescence microscope that the POD tincture permeated through the skin and distributed richly in both epidermis and dermis. Interestingly, unlike the extensive distribution of the POD tincture throughout the skin tissue, POD released from the POD-loaded GA micelles are mainly located in the epidermis. The small size of the nanoparticles induced a higher accumulation in the follicular region (Alvarez-Roman et al., Citation2004). This phenomenon suggested that there may be a novel mechanism during the interaction between the prepared GA micelles and the skins, which need further researches. In consideration of that the genital warts mainly occurred in the epidermis, the difference of POD distribution between the POD-loaded GA micelles formulation and POD tincture was very meaningful.
In addition, the in vivo skin permeation study also revealed that the POD-loaded GA micelles could release POD sustainably and steadily. HPV penetrates the skin or mucosa and invades the basal membrane in the epidermis. Longer drug retention in the skin was important to treat genital warts. The phenomenon showed in the drug retention figure significantly revealed the advantage of the POD-loaded GA micelles, which was sustained, steady, and deposited longer than the POD tincture. More significantly, the final skin deposition amount of POD for the POD-loaded GA micelles group was higher than that for the POD tincture group at 12 h interval. Drug retention that is maintained for 12 h might account for the GA micelles that are occluded on the skin surface that increase tissue hydration (Williams & Barry, Citation2004). These differences showed in the in vivo and in vitro studies further indicated the advantages of the micelles formulation.
GA has been reported to have an influence on drug permeation in the skin (Imai et al., Citation1999). It might be a potential sustained-release carrier with an adequate association to the drug and might be important factor affecting skin transportation. GA tends to be widely regarded as safe and low toxic (Rossi et al., Citation2005) during application. This study was inspired by finding that glycyrrhizic acid could be used as a potential carrier due to its amphipathic structure. Meanwhile, due to the steroid-like structure of GA (Tamaya et al., Citation1986; Park et al., Citation2004), the drug-loaded GA micelles might also have an anti-inflammation effect (Fukai et al., Citation2003; Asl & Hosseinzadeh, Citation2008). Currently, it still remains difficult to culture HPVs in tissues and establish animal models to evaluate the therapeutic effect of different POD dosage forms. Hence, HE staining and immunohistochemistry of normal skin were performed to evaluate the anti-inflammation effect of POD-loaded GA micelles. Epidermal keratinocytes could produce a number of cytokines such as TNF-α and IL-6 when cultured in the presence of various stimuli. TNF-α is a cell cytokine involved in systemic inflammation (Cohen & Heidary, Citation2004). TNF-α staining is localized in cells in the upper layers of the epidermis, stratum granulosum, stratum spinosum, and stratum corneum (Oxholm et al., Citation1988). IL-6 is a pro-inflammatory cytokine paradoxically associated with both skin healing and inflammation. It acts in an anti-inflammatory manner during irritant dermatitis (Lee et al., Citation2013). The immunohistochemical results demonstrated that the POD-loaded GA micelles could attenuate the expression of these two inflammatory cytokines (TNF-α and IL-6) in skin tissue, indicating that it had an anti-inflammatory effect. Wang et al. (Citation2011) also used to report that GA might provide an anti-inflammatory effect by attenuating the expression of LPS-induced tumor necrosis factor-α (TNF-α) and interleukin (IL)-6. Our finding was in consistent with his study. As POD usually caused skin irritation and skin inflammation during its administration in genital warts patients, this finding also further verified the advantages of our POD-loaded GA micelles.
Conclusion
Interest in the present study was inspired by the finding that glycyrrhizic acid could be used as a potential novel carrier. The effectiveness of applied therapeutic drugs is dependent on more drug retention in the ideal layer and less side effect. In this study, we prepared POD-loaded GA micelles formulation by utilizing the amphipathic and solubilizing properties of GA. More meaningfully, further studies indicated that POD-loaded GA micelles had a higher distribution in epidermis when compared with the clinically used POD tincture. Meanwhile, the POD-loaded GA micelles also caused less skin inflammation than traditional POD tincture. These advantages of the new POD delivery system indicated that it might be a superior and promising strategy for the treatment of genital warts. However, further studies are projected to evaluate the efficacy and mechanism of the formulation on skin.
Acknowledgements
We would like to sincerely thank the entire faculty in the Research Center of Clinical Medicine in Nanfang Hospital, where we performed this study.
Declaration of interest
The authors report no declarations of interest.
References
- Agren A, Elofsson R. (1967). Complex formation between macromolecules and drugs. I. Dialysis studies of phenol and polyethylene glycol (PEG). Acta Pharm Suec 4:281–92
- Alvarez-Roman R, Naik A, Kalia YN, et al. (2004). Skin penetration and distribution of polymeric nanoparticles. J Control Release 99:53–62
- Asl MN, Hosseinzadeh H. (2008). Review of pharmacological effects of Glycyrrhiza sp. and its bioactive compounds. Phytother Res 22:709–24
- Bi X, Zhu G, Han Z, et al. (2009). Protective effect of glycyrrhizin on nephrotic syndrome induced by adriamycin in rats. Clin Invest Med 32:E229–38
- Burd EM. (2003). Human papillomavirus and cervical cancer. Clin Microbiol Rev 16:1–17
- Chen H, Chang X, Du D, et al. (2006). Podophyllotoxin-loaded solid lipid nanoparticles for epidermal targeting. J Control Release 110:296–306
- Cohen DE, Heidary N. (2004). Treatment of irritant and allergic contact dermatitis. Dermatol Ther 17:334–40
- Cosmetic Ingredient Review Expert Panel. (2007). Final report on the safety assessment of Glycyrrhetinic Acid, Potassium Glycyrrhetinate, Disodium Succinoyl Glycyrrhetinate, Glyceryl Glycyrrhetinate, Glycyrrhetinyl Stearate, Stearyl Glycyrrhetinate, Glycyrrhizic Acid, Ammonium Glycyrrhizate, Dipotassium Glycyrrhizate, Disodium Glycyrrhizate, Trisodium Glycyrrhizate, Methyl Glycyrrhizate, and Potassium Glycyrrhizinate. Int J Toxicol 26:79–112
- Dahmani FZ, Yang H, Zhou J, et al. (2012). Enhanced oral bioavailability of paclitaxel in pluronic/LHR mixed polymeric micelles: preparation, in vitro and in vivo evaluation. Eur J Pharm Sci 47:179–89
- del Rio C, Hall G, Holmes K, et al. (2012). Update to CDC's sexually transmitted diseases treatment guidelines, 2010: oral cephalosporins no longer a recommended treatment for gonococcal infections (Reprinted from MMWR, vol 61, pg 590, 2012). J Am Med Assoc 308:1850–3
- Fomina MK, Avgustinovich DF, Tolstikova TG. (2014). Effects of buspirone complex with glycyrrhizic acid on behavior of mice with anxious-depressive state. Ross Fiziol Zh Im I M Sechenova 100:808–19
- Fukai T, Satoh K, Nomura T, Sakagami H. (2003). Preliminary evaluation of antinephritis and radical scavenging activities of glabridin from Glycyrrhiza glabra. Fitoterapia 74:624–9
- Gordaliza M, Garcia PA, del Corral JM, et al. (2004). Podophyllotoxin: distribution, sources, applications and new cytotoxic derivatives. Toxicon 44:441–59
- Hazzah HA, Farid RM, Nasra MM, et al. (2015). A new approach for treatment of precancerous lesions with curcumin solid-lipid nanoparticle-loaded gels: in vitro and clinical evaluation. Drug Deliv. [Epub ahead of print]. doi: 10.3109/10717544.2015.1065524
- Hermanns-Le T, Arrese JE, Goffin V, Pierard GE. (1998). Podophyllotoxin-induced acantholysis and cytolysis in a skin equivalent model. Eur J Morphol 36:183–7
- Imai T, Sakai M, Ohtake H, et al. (1999). In vitro and in vivo evaluation of the enhancing activity of glycyrrhizin on the intestinal absorption of drugs. Pharm Res 16:80–6
- Kao TC, Shyu MH, Yen GC. (2009). Neuroprotective effects of glycyrrhizic acid and 18beta-glycyrrhetinic acid in PC12 cells via modulation of the PI3K/Akt pathway. J Agric Food Chem 57:754–61
- Kao TC, Shyu MH, Yen GC. (2010). Glycyrrhizic acid and 18beta-glycyrrhetinic acid inhibit inflammation via PI3K/Akt/GSK3beta signaling and glucocorticoid receptor activation. J Agric Food Chem 58:8623–9
- Lacey CJ, Goodall RL, Tennvall GR, et al. (2003). Randomised controlled trial and economic evaluation of podophyllotoxin solution, podophyllotoxin cream, and podophyllin in the treatment of genital warts. Sex Transm Infect 79:270–5
- Lacey CJ, Woodhall SC, Wikstrom A, Ross J. (2013). 2012 European guideline for the management of anogenital warts. J Eur Acad Dermatol Venereol 27:e263–70
- Lee EG, Mickle-Kawar BM, Gallucci RM. (2013). IL-6 deficiency exacerbates skin inflammation in a murine model of irritant dermatitis. J Immunotoxicol 10:192–200
- Longstaff E, von Krogh G. (2001). Condyloma eradication: self-therapy with 0.15–0.5% podophyllotoxin versus 20–25% podophyllin preparations–an integrated safety assessment. Regul Toxicol Pharmacol 33:117–37
- Lu Y, Meng Q, Zhang G, Bei X. (2008). Clozapine-induced hepatotoxicity in rat hepatocytes by gel entrapment and monolayer culture. Toxicol In Vitro 22:1754–60
- Megrab NA, Williams AC, Barry BW. (1995). Oestradiol permeation across human skin, silastic and snake skin membranes: the effects of ethanol/water co-solvent systems. Int J Pharm 116:101–12
- Oxholm A, Oxholm P, Staberg B, Bendtzen K. (1988). Immunohistological detection of interleukin I-like molecules and tumour necrosis factor in human epidermis before and after UVB-irradiation in vivo. Br J Dermatol 118:369–76
- Park HY, Park SH, Yoon HK, et al. (2004). Anti-allergic activity of 18beta-glycyrrhetinic acid-3-O-beta-D-glucuronide. Arch Pharm Res 27:57–60
- Polyakov NE, Khan VK, Taraban MB, et al. (2005). Complexation of lappaconitine with glycyrrhizic acid: stability and reactivity studies. J Phys Chem B 109:24526–30
- Polyakov NE, Leshina TV, Salakhutdinov NF, Kispert LD. (2006). Host-guest complexes of carotenoids with beta-glycyrrhizic acid. J Phys Chem B 110:6991–8
- Qin L, Xue M, Wang W, et al. (2010). The in vitro and in vivo anti-tumor effect of layered double hydroxides nanoparticles as delivery for podophyllotoxin. Int J Pharm 388:223–30
- Rossi T, Benassi L, Magnoni C, et al. (2005). Effects of glycyrrhizin on UVB-irradiated melanoma cells. In Vivo 19:319–22
- Roy A, Ernsting MJ, Undzys E, Li SD. (2015). A highly tumor-targeted nanoparticle of podophyllotoxin penetrated tumor core and regressed multidrug resistant tumors. Biomaterials 52:335–46
- Sapra B, Jain S, Tiwary AK. (2008). Transdermal delivery of carvedilol containing glycyrrhizin and chitosan as permeation enhancers: biochemical, biophysical, microscopic and pharmacodynamic evaluation. Drug Deliv 15:443–54
- Selyutina OY, Polyakov NE, Korneev DV, Zaitsev BN. (2015). Influence of glycyrrhizin on permeability and elasticity of cell membrane: perspectives for drugs delivery. Drug Deliv. [Epub ahead of print]. doi: 10.3109/10717544.2014.919544
- Syed TA, Cheema KM, Khayyami M, et al. (1995). Human leukocyte interferon-alpha versus podophyllotoxin in cream for the treatment of genital warts in males. A placebo-controlled, double-blind, comparative study. Dermatology 191:129–32
- Tamaya T, Sato S, Okada HH. (1986). Possible mechanism of steroid action of the plant herb extracts glycyrrhizin, glycyrrhetinic acid, and paeoniflorin: inhibition by plant herb extracts of steroid protein binding in the rabbit. Am J Obstet Gynecol 155:1134–9
- Tolstikova TG, Khvostov MV, Bryzgalov AO. (2009). The complexes of drugs with carbohydrate-containing plant metabolites as pharmacologically promising agents. Mini Rev Med Chem 9:1317–28
- Tolstikova TG, Khvostov MV, Bryzgalov AO, et al. (2010). Improvement of the pharmacological activity of nifedipine by mechanochemical complexation with glycyrrhizic acid. Biomed Khim 56:187–94
- Tu JH, Hu DL, Dai LL, et al. (2010). Effect of glycyrrhizin on CYP2C19 and CYP3A4 activity in healthy volunteers with different CYP2C19 genotypes. Xenobiotica 40:393–9
- Vavilin VA, Salakhutdinov NF, Ragino Iu I, et al. (2008). Cholesterol lowering properties of a complex compound simvastatin with glycyrrhizic acid (simvaglyzin) in experimental models. Biomed Khim 54:301–13
- Wang CY, Kao TC, Lo WH, Yen GC. (2011). Glycyrrhizic acid and 18beta-glycyrrhetinic acid modulate lipopolysaccharide-induced inflammatory response by suppression of NF-kappaB through PI3K p110delta and p110gamma inhibitions. J Agric Food Chem 59:7726–33
- Wang SQ, Liu ST, Zhao BX, et al. (2015). Afatinib reverses multidrug resistance in ovarian cancer via dually inhibiting ATP binding cassette subfamily B member 1. Oncotarget 6:26142–60
- Williams AC, Barry BW. (2004). Penetration enhancers. Adv Drug Deliv Rev 56:603–18
- Xie FM, Zeng K, Li GF, et al. (2005). Preparation of stearic acid solid lipid nanoparticles containing podophyllotoxin. Di Yi Jun Yi Da Xue Xue Bao 25:99–101
- Xie Y, Ruan B, Chen Y, et al. (2011). Kaposi's sarcoma-associated herpesvirus infection in Chinese patients with chronic hepatitis B. J Med Virol 83:879–83
- Yadav R, Kumar D, Kumari A, Yadav SK. (2014). Encapsulation of podophyllotoxin and etoposide in biodegradable poly-D,L-lactide nanoparticles improved their anticancer activity. J Microencapsul 31:211–19
- Yang FH, Zhang Q, Liang QY, et al. (2015). Bioavailability enhancement of paclitaxel via a novel oral drug delivery system: paclitaxel-loaded glycyrrhizic acid micelles. Molecules 20:4337–56