Abstract
Topical photodynamic therapy (PDT) is a promising alternative for malignant skin diseases such as basal-cell carcinoma (BCC), due to its simplicity, enhanced patient compliance, and localization of the residual photosensitivity to the site of application. However, insufficient photosensitizer penetration into the skin is the major issue of concern with topical PDT. Therefore, the aim of the present study was to enable penetration of photosensitizer to the different strata of the skin using a lipid nanocarrier system. We have attempted to develop a nanostructured lipid carrier (NLC) for the topical delivery of second-generation photosensitizer, 5-amino levulinic acid (5-ALA), whose hydrophilicity and charge characteristic limit its percutaneous absorption. The microemulsion technique was used for preparing 5-ALA-loaded NLC. The mean particle size, polydispersity index, and entrapment efficiency of the optimized NLC of 5-ALA were found to be 185.2 ± 1.20, 0.156 ± 0.02, and 76.8 ± 2.58%, respectively. The results of in vitro release and in vitro skin permeation studies showed controlled drug release and enhanced penetration into the skin, respectively. Confocal laser scanning microscopy and cell line studies respectively demonstrated that encapsulation of 5-ALA in NLC enhanced its ability to reach deeper skin layers and consequently, increased cytotoxicity.
Introduction
Basal-cell carcinoma (BCC) is the most common form of skin cancer in the white population, with the incidence rate increasing at over 4% yearly in all countries and social classes. It has been estimated that approximately 50% of all fair-skinned individuals (40–60 years old) are developing this type of skin cancer (Lear et al., Citation1998; Leiter & Garbe, Citation2008). BCC ascends from the basal layer of epidermis and its appendages and is locally invasive, aggressive, and destructive of skin and the surrounding structures. For treating such cutaneous malignant skin lesions, topical photodynamic therapy (PDT) is the latest and extremely beneficial option; this comprises topical application of a photosensitizer at the site of skin disease, followed by illumination and consequent death of selected cells. It involves the use of a photosensitizing agent, oxygen, and light of a specific wavelength (comparable to the absorption characteristics of the photosensitizer) which results in the formation of the highly cytotoxic reactive oxygen species (ROS) that cause tumor cell death (Dougherty et al., Citation1998; Hopper, Citation2000; Biel, Citation2010). The main advantages of PDT are its simplicity, minimal invasive nature, lack of systemic toxicity, and selectivity to the site of application. However, the major challenge in using topical PDT is the insufficient photosensitizer penetration into the skin, which limits its use to superficial skin lesions. To address this hurdle, nanostructured lipid carriers (NLC) have been utilized as a carrier system to enhance the penetration of drug into the deeper layers of the skin. NLC is a promising approach for enhancing percutaneous drug absorption attributable to its unique composition of binary lipids (oil and solid lipids) which are spatially incompatible, as well as higher encapsulation of incorporated drug in disordered matrix structure. These characteristics of NLC make it a desirable carrier system for improving the efficacy of topical PDT due to improved adhesiveness, occlusion, and ultimately effective skin targeting (Muller et al., Citation2000).
Second-generation photosensitizers have provided better therapeutic prospects for PDT, allowing shorter periods of photosensitivity, higher quantum yields of singlet oxygen, and higher tumor to normal tissue concentration ratios (Kubler et al., Citation1999; Biel, Citation2010). Among these, 5-aminolevulinic acid (5-ALA) is a potential drug candidate as it is the precursor of the potent endogenous photosensitizer protoporphyrin IX (PpIX). Upon 5-ALA application, the cells generate PpIX through the heme biosynthetic pathway, as exposure of cells to excess of exogenous 5-ALA bypasses the first rate-limiting step in the biosynthesis of heme (i.e. synthesis of ALA from succinyl-CoA and glycine by the enzyme ALA synthetase) which results in synthesis and accumulation of PpIX. PpIX, which has marked photosensitizing ability, is activated upon exposure to light with a wavelength ranging from 405 to 635 nm (blue to red light), with peak tumor fluorescence occurring for 3–5 h after 5-ALA administration. The predominant benefit of PpIX in contrast to other photosensitizers is the short half-life of its photosensitizing effects, which means that PpIX is entirely cleared from the body within 24 h (Curic & Fahr, Citation2012).
The major problem associated with the conventional topical formulation of 5-ALA for PDT is insufficient drug penetration into the skin due to its hydrophilic nature. 5-ALA forms zwitter ions at physiological pH resulting in low penetration and nonhomogenous distribution of 5-ALA-mediated PpIX after topical application (Rosa et al., Citation2003).
Therefore, the aim of the present study was to develop and optimize a topical NLC formulation of 5-ALA to enhance its penetration in the skin to the basal cells layer, thereby enhancing its cytotoxic effect. The developed NLC of 5-ALA was assessed in vitro for drug release, skin permeation and effects on cell viability, and in vivo for penetration of the drug to the different strata of the skin (up to the basal layer) using confocal laser scanning microscopy (CLSM).
Materials and methods
The 5-aminolevulinic acid (5-ALA) was purchased from Genex life science Pvt Ltd (Bangalore, India); glyceryl dibehenate (Compritol ATO 888®) was received as a gift sample from Gattefosse (Saint Priest, Cedex France). Oleic acid, sodium taurocholate, Tween 20, stearic acid, glyceryl monostearate, cutina P®, casein, L-cysteine, groundnut oil, ethyl oleate, soyabean oil, castor oil, peanut oil, Precirol ATO 5®, Gelucire 44/14®, linseed oil, Labrafac®, caprylo caproyl macrogol-8-glyceride (Labrasol®), poloxamer 188, Tween 80, castor oil, and methanol (HPLC grade) were purchased from Merck (Schuchardh, Hokenbrunn, Germany). Carbopol 934, triethanolamine, mannitol, acetyl acetone, formaldehyde, and potassium dihydrogen orthophosphate were all purchased from S.D. Fine Chemicals, Ltd (Mumbai, India). Dialysis sacs (mol. wt. cutoff: 12 000 Da) was purchased from Sigma Aldrich Chemicals (St Louis, MO). All chemicals were of analytical grade.
Determination of 5-ALA by spectrofluorometry
To quantify 5-ALA in phosphate buffer 5, a simple, accurate, and sensitive spectrofluorometric method was developed and validated using Perkin Elmer LS-55 instrument (Waltham, MA). To enable the detection of 5-ALA by spectrofluorometer, the drug was derivatized to make the compound into a fluorophor. Derivatization was based on a modification of the Hantzsch reaction, in which amine compounds react with acetyl acetone and formaldehyde to form a fluorescent compound (Lee et al., Citation2004).The developed spectrofluorometric method was validated according to ICH guidelines, Q2 (R1) (ICH, 2005) with respect to linearity, range, precision, accuracy, limit of detection (LOD), and limit of quantitation (LOQ). All measurements were made in triplicate. A linear calibration curve (fluorescence intensity versus concentration [μg/ml]) was obtained for 5-ALA with R2 – 0.996 within the range of 0.1–1 μg/ml. The low values of inter- and intra-day coefficients of variation within ±5% suggested an excellent precision of the method. The percentage recovery was f 99.05–101.4%, indicative of high accuracy. LOD and LOQ determined by standard deviation method were 0.005 μg/ml and 0.015 μg/ml, respectively.
Selection of excipients
Selection of liquid lipids (oils) was based on their drug solubilization potential. An excess amount of 5-ALA was placed in 3 ml of oils (soybean oil, castor oil, peanut oil, oleic acid, and ethyl oleate) in small vials. The vials were tightly stoppered and were continuously shaken for 72 h at 25 °C in mechanical shaker to reach equilibrium. The mixtures were centrifuged using a high-speed centrifuge (3K30, SIGMA, Germany) at 5000 rpm for 30 min; the supernatant fluid was dissolved in methanol, and solubility was quantified by spectrofluorometry at a emission wavelength of 460 nm. The solubility studies were done in triplicate. For selecting solid lipids, solid and liquid lipids were mixed in different ratios of 90:10, 80:20, 70:30, 60:40, and kept at room temperature for 24 h. The samples were assessed visually for turbidity and phase separation. The lipid mixtures with good miscibility were used for preparing 5-ALA NLC. Surfactants were selected on the basis of observation of aqueous titration. Primary emulsions were prepared by melting and agitating the lipid mixture using a magnetic stirrer, to which different surfactants were added followed by drop-wise addition of double-distilled water. The preparations obtained were observed for clarity and homogeneity. Those formulations which were clear and transparent were selected for further experimentation.
Preparation and optimization of NLC
To prepare NLC using a microemulsion technique, the first step was to construct a pseudoternary diagram using different ratios of surfactant. NLCs were prepared using the method of Souza et al. (Citation2011) with slight modifications. The accurately weighed solid and liquid lipid mixture were heated above the melting point of the solid lipid followed by addition of the surfactant. To this mixture, 500 μl of water was added to form a strong, thermodynamically stable emulsion. Drug was added to this microemulsion. This hot microemulsion was then dispersed into cold water (2–4 °C) under vigorous stirring (using Remi Instruments Ltd., Mumbai, India) in 1:20 ratio (microemulsion: water) to form NLC dispersion (Souza et al., Citation2011). It has been reported that the NLC dispersions formed by this method contained large quantity of particles in the micron range; therefore, in this condition, the time of stirring, percentage of lipids, and amount of drug were optimized in order to obtain the appropriate size and a higher entrapment efficiency.
Physicochemical characterization of NLC
Particle size, PDI, and morphology of NLC
Photon correlation spectroscopy using a Zetasizer Nano ZS (Malvern Instruments, Worcestershire, UK) was used to measure the hydrodynamic particle size and particles-size distribution (polydispersity index [PDI]) of NLC. The actual particle size and shape for the prepared NLC were determined by using transmission electron microscopy (TEM; Morgagni 268 TEM, MA, USA). A drop of dispersion was placed on a paraffin sheet and carbon-coated grid was put on the sample and left for 1 min to allow NLC to adhere. The remaining drug suspension was removed by adsorbing the drop with the corner of a piece of filter paper. The grid was then placed on the drop of phosphotungstate for 10 s. The remaining solution was removed by absorbing the liquid with a piece of filter paper, and the sample was air-dried. The sample was examined by TEM (Morgagni 268D TEM, MA, USA). The morphology was determined for the prepared nanoparticle using SEM. The NLC dispersion was lyophilized by adding 5% w/v mannitol and freezing at −78 °C for 10 h followed by lyophilization for 36 h. A sample of freeze-dried NLC was mounted on metal stubs; gold coated under vacuum and then examined using a Zeiss EVO40, Carl Zeiss NTS (North America).
Differential scanning calorimetry
The DSC thermograms of 5-ALA, Compritol, physical mixture, and freeze-dried NLC were obtained using a DSC-821 (Mettler toledo, Greifensse, Switzerland) equipped with Pyris 6 DSC version 4.0 software. The nitrogen gas flow was 60 ml/min. A sample (2–4 mg) was weighed and sealed in an aluminium pan of 40 μl capacity and equilibrated at 25 °C. This was subjected to DSC run over the temperature range of 100–200 °C, at a heating rate of 10 °C/min. The temperature was calibrated using pure indium with a melting point of 156.60 °C; an empty pan was used as a reference.
Drug entrapment efficiency
The 5-ALA-loaded NLCs were separated from the aqueous suspension medium by ultracentrifugation at 16 000 rpm and 4 °C for a period of 45 min and the supernatant was collected; the drug-loaded lipid formulation settled at the bottom. The quantity of free drug was measured in the clear supernatant using spectrofluorometry at an emission wavelength of 460 nm after derivatization. The supernatants of the formulations after centrifugation were taken without further processing and filtered through a 0.45-μm filter; entrapment efficiency was determined by spectrofluorometry. The pellets formed after centrifugation were dissolved to form a drug solution; this was filtered through 0.45-μm filter, and the drug content was determined by spectrofluorometry.
Preparation and characterization of 5-ALA NLC gel
The NLC dispersion of 5-ALA was converted into a gel by dispersing 0.5, 1, and 1.5% of Carbopol 934 in the selected NLC dispersion and subsequently neutralizing the carbopol using triethanolamine solution. The prepared gel was characterized for spreadability, viscosity, and pH. For determining the spreadability, gel (0.5 g) was placed within a circle of 1-cm diameter premarked on a glass plate, over which a second glass plate was placed. A weight of 500 g was allowed to rest on the upper glass plate for 5 min. The increase in the diameter due to gel spreading was noted. The viscosity of the NLC gel was studied using a Brookfield viscometer (Brookfield Engineering Laboratories, Inc., Middleboro, MA) with spindle no.6 at 10 rpm at temperature of 37 ± 0.5 °C. The pH of the developed NLC gel was determined by placing 100 mg of the gel in a 50-ml volumetric flask, and then the volume was made up with distilled water. The pH of the dispersion was measured using a pH meter (Microprocessor pH System Punjab, India).
In vitro drug release study
The in vitro release of 5-ALA from the lipid carrier was carried out using a dialysis membrane method. NLC gel equivalent to 0.5 mg of drug entrapped was transferred to a pretreated dialysis bag. The bag was suspended in 100 ml of phosphate buffer (PBS; pH 5) at 32 °C ± 0.5 °C and stirred using a magnetic stirrer at 100 rpm. At predetermined time intervals, aliquots were withdrawn from the release medium and replaced with same amount of PBS. The quantity of drug was determined by spectrofluorometry; in each experiment, the samples were analyzed in triplicate (Cirri et al., Citation2012).
Data obtained from the in vitro release studies of 5-ALA-loaded NLCs were fitted into various kinetics models like zero order (fraction drug release versus time), first order (log % drug remaining versus time), Higuchi mode (fraction drug release versus square root of time), and Peppas model (% R = Ktn, where %R is the percentage drug release, K is the kinetic constant, and n is the release exponent and is the measure of release mechanism) to interpret the mechanism and kinetics of drug release. R2 values were calculated for the linear curve obtained by regression analysis of the plots obtained. The n value is obtained from the slope of Peppas model. If the value of n is 0.43 or less, the release of drug from the formulation follows Fickian diffusion mechanism, while the higher value of n (0.43 < n < 0.85) indicates a non-Fickian mechanism of drug release. If the n value is 0.85, the drug release follows zero order and Case II transport (Ferdous et al., Citation2012).
Animals and cell line experiments
All animal experiments were carried out in accordance with the approval obtained from Jamia Hamdard, Institutional Animal Ethics Committee (173/CPCSEA, 28 January 2000), and their guidelines were followed throughout the course of studies. All procedures were performed on sacrificed animals by giving high dose of general anesthesia with inhaled chloroform. Subsequently, animal skin was excised, washed with distilled water, and the surface hair was removed by shaving and depilating (Veet®) followed by cleaning with distilled water. Excess fat was removed using isopropyl alcohol and cleaned until a transparent skin was seen; care being taken to avoid disruption of the skin.
For in vitro skin permeation studies, albino Wistar rats (200–250 g) of either sex were used. Swiss albino mice (of either sex: 7–8-weeks old), weighing between 10 and 30 g, were used for the CLSM. The animal dose for conducting in vivo studies was calculated on the basis of body surface area ratio of animal model to that of a human being.
Cell viability analysis of optimized formulation was assessed on vero E6 cell lines using the MTT (3-[4,5-dimethylthiazol-2-yl]-2,5-diphenyltetrazolium bromide) assay. The cells were cultured in DMEM media supplemented with 10% fetal bovine serum, penicillin-G (100 units/ml), and streptomycin (100 μg/ml). A cell suspension of 100 μl (105 cells/ml) was added into a 96-well plate (Nest Biotech, China) and incubated for 24 h at 37 °C and 5% CO2 in a CO2 incubator (New Brunswick, NJ).
In vitro skin permeation study
The skin permeation of 5-ALA was measured using a Franz cell assembly (Logan Instrument Ltd., Somerset, NJ). Full-thickness dorsal abdominal skin of a rat was mounted between the donor and receptor compartments. The donor compartment consists of 1 g of the gel containing drug. The receptor medium consists of (10 ml) PBS pH 5. The drug concentration in the donor compartment (Cd) was 1250 μg/ml. The available diffusion area between compartments was 0.785 cm2. The stirring rate and temperature of the receptor compartment were respectively maintained at 600 rpm and 32 °C. At appropriate intervals, 500-μl aliquots of the receptor medium were withdrawn and immediately replaced with equal volumes of fresh buffer. The cumulative quantities of 5-ALA were determined using spectrofluorometry. The cumulative quantity of drug permeated through the skin (Q, μg/cm2) was plotted as a function of time (h). The drug flux (permeation rate) at steady state (J, μg/cm2/h) was calculated from the slope of linear portion of the curve (between cumulative amounts of drug permeated per cm2 versus time). The permeability coefficient (Pb) is equal to the flux/drug concentration in donor compartment (μg/ml).
At the end of the experiment (24 h), a glass transfer pipette was used to remove the formulation from the donor compartment. The surface of the skin was thoroughly washed with distilled water to remove any excess formulation (Tan et al., Citation2011) and allowed to dry at ambient temperature. The area of the skin in contact with the formulation (corresponding to the diffusion area of the Franz cell) was then punched out, weighed accurately, and cut into fine pieces; 5 ml of the PBS was added to the skin pieces and homogenized using High Speed Centrifuge (3K30, SIGMA, Germany). The homogenized residue was sonicated for 60 min using a VWR Ultrasonic B5500A-DTH sonicator (VWR International, LLC, Radnor, PA), followed by centrifugation at 4000 rpm for 30 min using a centrifuge (Remi Laboratory Mumbai, India). The supernatant obtained after centrifugation was collected and analyzed using Spectrofluorometry.
Cell viability studies
Serially diluted ALA-NLC formulations (200 μl) and pure drug were added into 96-well plate in triplicates and incubated for 24 h at the same conditions. MTT (5 mg/ml in DPBSA; 20 μl) was added to the 96-well plate to check the cell viability and incubated for 4 h at the same conditions. Then the solution was decanted and replaced with 200 μl of dimethyl sulphoxide (DMSO) to terminate the assay, and absorbance was measured at 570 nm using ELISA microplate reader (Biorad, Hercules, CA) at 60-sec mixing. Untreated cells were taken as positive control, and media only were taken as blank.
In vivo study using confocal laser scanning microscopy
A glass cylinder with an available area of 0.785 cm2 was placed on the dorsal skin of a mouse with glue. Drug solution gel and NLC gel were added to each cylinder, which was then be covered with parafilm. After being applied for 4 h, each skin site was wiped 10 times using a cotton-tipped stick with distilled water, and the mice were divided into two groups: irradiated and nonirradiated groups. At the end of the irradiative protocol, the treated area was excised. The amount of ALA-induced PpIX retained in the skin was measured by CLSM. All procedures were carried out in the dark to prevent the influence of ambient light. A normal LED bulb was used instead of a PDT instrument for the irradiation purpose (Enk & Levi, Citation2012). Skin samples obtained following in vivo treatment were examined for PpIX fluorescence images by CLSM. Each full skin sample was sliced in sections of 6–13 mm in thickness through the z-axis and examined with CLSM. Optical excitation was carried out with a 488-nm argon laser beam, and the fluorescence emission was detected at 590 nm (Fang et al., Citation2008).
Results and discussion
Selection of excipients
The 5-ALA is poor soluble in the majority of liquid lipids, which is probably due to its highly hydrophilic nature. Oleic acid was selected because the drug was soluble in this liquid lipid. In addition to being a liquid lipid, oleic acid is a known penetration enhancer and has been found to enhance the skin delivery of many drugs such as voriconazole (Song et al., Citation2012). Oleic acid was also found to be an optimizer in the topical enhancement of 5-ALA (Pierre et al., Citation2006). Compritol ATO 888 and oleic acid in the ratio of 70:30 showed good miscibility and transparency; therefore, Compritol ATO 888 was selected as the solid lipid. On the basis of clarity and stability of the primary emulsion formed by aqueous titration, Tween 20 was selected as a surfactant for the preparation of NLC of 5-ALA.
Preparation and optimization of NLC
The method used for active pharmaceutical ingredients (APIs), such as 5-ALA which shows low solubility in lipid, was first developed by Gasco (Citation1993) for the preparation of SLNs by the microemulsion method. This method utilized a basic microemulsion protocol followed by the transfer of the warm microemulsion into cold water, resulting in the precipitation of the lipid phase forming solid nanoparticles (Gasco, Citation1993). Dilution of the microemulsion and HPH techniques are considered to be the most feasible methods for preparing SLN of lipophilic drugs (Muchow et al., Citation2008). Another example of the incorporation of hydrophilic drugs into a lipid matrix was given by Aroujo et al. (Citation2010); here, the hydrophilic drug was incorporated into lipid matrix by using the microemulsion technique, producing satisfactory entrapment efficiency which is the major challenge for any hydrophilic drug (Aroujo et al., Citation2010). Furthermore, the incorporation of nitrosyl ruthenium complex into NLC for the topical treatment of skin cancer showed that conventional high pressure homogenization cannot be utilized due to the high hydrophilicity of the drug; therefore, the microemulsion method was adopted for the preparation of NLC (Oliveira et al., Citation2010). Based on these findings, 5-ALA NLCs were prepared and optimized using the microemulsion technique. Pseudoternary phase diagram was constructed to optimize the concentration of lipids and surfactants in NLC formulation (). On this basis, a lipid:surfactant ratio of 1:2 was selected for the preparation of microemulsions. However, different concentrations of lipids (1, 1.5, and 2%) were used, for which the surfactant concentration was 2% for formulation development, and the influence on particle size, PDI, and % drug entrapment was noted.
Physicochemical characterization of NLC
Particle size, PDI, and morphology of NLC
The optimized NLCs were in the nanometric size range with low PDI indicative of monodispersity and homogeneity of formulations. It was concluded that when the quantity of lipid (solid lipid + liquid lipid) was 1.5% with a stirring time of 30 min, the mean particle size was in the range of 165.2 ± 1.20 nm, respectively, and the mean PDI was 0.15 ± 0.026 for the placebo NLC. On the other hand, the drug-loaded formulation had a mean particle size of 194.16 ± 5.73 nm and a PDI of 0.24 ± 0.03; hence, it was concluded that there is an increase in the mean particle size from 165.2 ± 1.20 to 194.16 ± 8.15 nm with the incorporation of drug. The particle size studies also revealed that an increase in the lipid concentration leads to a considerable increase in particle size, whereas an increase in stirring time notably reduced the particle size (). The increase in lipid content resulted in a nonuniform distribution of lipid nanoparticles, as evident from higher values of PDI for increased lipid concentrations (). The particle size observed in TEM photomicrographs (around 100 nm) was less than that of the NLC1B determined using the particle-size analyzer (185.2 ± 1.20 nm). The apparent discrepancies between particle size measured by dynamic light scattering (DLS) by Zetasizer and TEM can be explained by the dehydration of NLC1B during sample preparation for the imaging of TEM due to which particle size is less as compared to the DLS (Fazil et al., Citation2012). The SEM photomicrograph revealed that the NLCs were spherical with a more less smooth surface. Occasional clumps were also observed in some of the images; these might arise from problem associated with the shrinkage of NLCs during drying or concentration of dispersion medium.
Table 1. Optimization parameter for drug-loaded NLC.
Differential scanning calorimetry
DSC was used to elucidate the changes in the degree of crystallinity of the substance. DSC thermograms of Compritol ATO 888, 5-ALA, physical mixture, and 5-ALA-loaded freeze-dried NLC are shown in . Drug-loaded NLC showed an endothermic peak at 68.97 °C, the drug showed an endothermic peak at 157.048 °C, the solid lipid showed an endothermic peak at 63.59 °C, and the physical mixture showed both peaks of drug (152.7 °C) as well as the binary solid–liquid lipid (73.52 °C). Thus, the sharp peak at 157.048 °C exhibited by 5-ALA was not visible in the 5-ALA-loaded NLC, indicating that 5-ALA was completely encapsulated in the NLC. The absence of the characteristic peak of the drug suggests that the crystalline form may have transformed into the amorphous form. The shift in the peak of solid lipid from 63.59 °C to 68.97 °C in the NLC formulation might be due to interaction between the binary lipids and the drug, which might have influenced the melting and crystallization behavior of solid lipid.
Drug entrapment efficiency
NLC formulations were characterized for drug entrapment and loading efficiency. The observed drug entrapment efficiency values demonstrated that the developed lipid carriers had high entrapment efficiency; this had otherwise been a difficult task in the encapsulation of hydrophilic drugs into a lipid carrier. High entrapment efficiency may be related to the incorporation of the drug in the surfactant layer at the surface of the lipid carrier (Oliveira et al., Citation2010). During the microemulsion formation, the drug can partition from the oil phase to the aqueous phase. However, during the cooling of the O/W microemulsion, the solubility of the drug in the water phase can decrease, leading to the re-partitioning of the drug to the lipid phase. When the lipid recrystallization temperature is reached, a solid lipid core starts forming that includes the drug, which is present at this temperature in this lipid phase. The already-crystallized core is no longer accessible for the drug; consequently the drug concentrates in the still liquid outer shell of the NLC and/or on the surface of the particles (Oliveira et al., Citation2010).
During the characterization study of different drug-loaded NLC formulations, it was realized that increase in lipid concentration had no significant influence on the percentage drug entrapment. This could be explained by the poor lipophilicity of the drug resulting in the drug showing low affinity for the binary lipid matrix of NLC. The highest % drug entrapment was observed using a 1% lipid concentration for 30-min stirring time (). Therefore, NLC1B was selected as the final formulation for further investigation. The finalized formula for the optimized formulation NLC1B is summarized in . Based on the results of particle size, PDI, and percentage drug encapsulation efficiency (), 1% of the lipid mixture (solid lipid + liquid lipid), 2% of surfactant solution, and 0.1% of sodium taurocholate (stabilizer) were optimized for final formulation development.
Table 2. Optimized formula of 5-ALA NLC.
Preparation and characterization of 5-ALA NLC gel
The optimized NLC1B dispersion was formulated into a gel by using 0.5, 1, and 1.5% Carbopol 934. Among different percentage of Carbopol 934, the best gel formation was observed with 1% of gelling agent with good spreadability. Spreadability means the area to which gel readily spreads on application to skin or effected part. The increase in the diameter due to spreading of the gel preparation was 7.1 ± 0.05 cm, indicating a good spreadability of the obtained gel preparations. Spreadability is an essential property of topical formulations from the point of view of patient compliance. The viscosity of the optimized gel preparation was found to be 567 ± 5.3 cps. The apparent pH of the gel system was found to be 6.87 ± 0.03 which was found to be in optimum range with the skin pH.
In vitro drug release study
The in vitro drug release showed an initial rapid release followed by a slow release. The initial rapid release might be due to the un-entrapped drug released from the NLC1B, while the slow release was from its core. Hence, 5-ALA NLC exhibited a controlled drug release, with 54% of the drug released within 5 h; this was because of the presence of the solidified binary lipid of Compritol ATO 888 and oleic acid. In contrast, the drug release from the drug solution showed rapid release of 80% drug in 5 h (as shown in ).
The release kinetics were characterized by fitting the data from in vitro release studies of NLCs in to standard release equations (zero order, first order, Higuchi model, and Korsmeyer–Peppas model). The model that best fits the release data was as per the Higuchi model which has higher R2 value of 0.825. When the release mechanism was analyzed using Korsmeyer–Peppas model, the value of n was found to be 0.658 indicated that the release of drug from NLC1B followed non-Fickian diffusion.
In vitro skin permeation studies
Insufficient penetration of 5-ALA into the skin resulting in low doses reaching the site of action, therefore restricting local activity, is the main issue of concern in its dermatopharmacokinetics. In order to overcome the problem of low 5-ALA-skin penetration with minimal or bypassed transdermal effects, topical 5-ALA-loaded NLCs were developed and investigated for their penetration enhancing ability. In this work, we have attempted to encapsulate 5-ALA, a hydrophilic compound into a lipid carrier which can easily penetrate the skin barrier and ultimately reach different strata of the skin, showing little or no transdermal effects. We found that the percentage of drug permeated through the skin was 10.5% of the total dose, and the flux was very low; therefore, it was necessary to quantify the drug in the skin. The cumulative amount of drug present in the skin was 668.4 μg/ml, which was about 53.4% of the total dose (). Thus, there was increased retention of 5-ALA in the target skin layers (basal cells layer of epidermis). Hence, it was concluded that the majority of the drug was present in the skin (Bose & Michniak-Kohn, Citation2013).
Cell viability study
There was a significant difference (p < 0.05) between the cytotoxic effect when NLC of 5-ALA was compared with 5-ALA solution (control; ). Notably, the NLC1B showed approximately four times higher cytotoxicity than did the drug solution which confirm the higher cellular uptake and considerably reduced cell viability via NLC1B formulation in contrast to drug solution. The multi-folds increased cytotoxicity of NLC1B was attributable to the incorporation of 5-ALA into NLC; this would have provided a higher concentration gradient for the drug to cross different layers of the epidermis and reach the basal cell layer in increased concentration. Therefore, the results of the MTT assay demonstrated great potential of NLC formulation for enhancing the PDT efficiency.
Confocal laser scanning microscopy
The increase in percutaneous absorption of 5-ALA and the ability of NLC1B to enhance penetration of the hydrophilic photosensitizer to deeper layers of skin was analyzed via CLSM ( and ). This revealed that the NLC1B-treated group showed higher penetration than the aqueous control group. The nonirradiated groups of both NLC1B and aqueous control showed higher fluorescence intensity than their irradiated counterparts () which was due to the photo-bleaching effect that occurred during the PDT-irradiation process. Photo-bleaching, or photochemical degradation, causes a loss of fluorescence intensity due to photon-induced chemical damage and irreversible covalent modifications by light exposure; this is in agreement with the work of Fang et al. (Citation2008).
Figure 6. (a) z-axis image of control-treated irradiated group (b): z-axis image of NLC-treated irradiated group (c) z-axis image of NLC-treated nonirradiated group.
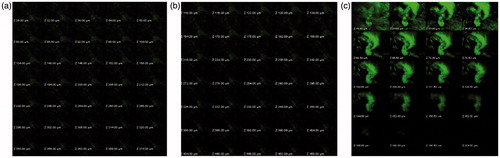
Figure 7. (a) CLSM image of control-treated irradiated group (b) CLSM image of NLC-treated nonirradiated group (c) CLSM image of NLC-treated irradiated group.
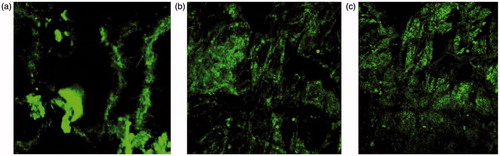
Table 3. Results of in vivo studies.
The substantial increase in the concentration of 5-ALA in deep skin layers could be explained by the sufficient hydration and occlusion of skin caused by the NLC formulation. The design of the NLC resulted in its binary lipids (oleic acid and Compritol ATO 888) providing good adhesion to the skin surface, while the presence of surfactant (Tween 20) enhanced the penetration of drug to the basal cell layer of the epidermis (). Furthermore, Carbopol 934 which is a cross-linked polyacrylate polymer, adds to the penetration enhancement of 5-ALA to the deeper skin layer by improving the adhesion and occlusion of the NLC system.
Due to the high lipophilicity of 5-ALA-loaded NLC1B (hybrid of oleic acid and Compritol ATO 888), the formulation exhibited an effective penetration into the deeper layers of skin as shown in and . The results seem to be promising for facilitating the treatment of BCC by enhancing the percutaneous absorption of the drug into the skin, this being a major challenge in topical PDT using 5-ALA. As the low lipid solubility of 5-ALA limits its clinical application in deeper skin lesions, its encapsulation into NLC serves to enhance its penetration into the skin and consequently increasing the PpIX accumulation in the skin.
Conclusion
In the present study, 5-ALA-loaded NLCs were successfully developed with increased 5-ALA concentration in the target skin layers. This may improve the therapeutic prospects of topical PDT, since the accumulation of a potent photosensitizer i.e. PpIX is directly related to the presence of 5-ALA in the skin. On the basis of CLSM showing enhanced drug penetration in deeper skin layers, and the demonstration of increased cytotoxicity of the 5-ALA NLC, it was concluded that NLC can act as a promising drug carrier for increasing the accumulation of PpIX by enhancing the penetration of hydrophilic drugs such as 5-ALA into deep skin layers, thereby improving the efficacy of topical PDT.
Acknowledgements
The authors are thankful to All India Council of Technical Education (AICTE), New Delhi, India for providing financial assistance to project. The authors also thank Professor Brian L. Furman (University of Strathclyde, Glasgow, UK) for critical reading of the manuscript and English editing.
Declaration of interest
The authors report no conflicts of interest. The authors are responsible for the content and writing of the paper.
References
- Aroujo LM, Thomazine JA, Lopez RFV. (2010). Development of microemulsions to topically deliver 5-aminolevulinic acid in photodynamic therapy. Eur J Pharm Biopharm 75:48–55
- Biel MA. (2010). Photodynamic therapy in head and neck cancer. Curr Oncol Reports 4:87–96
- Bose S, Michniak-Kohn B. (2013). Preparation and characterization of lipid based nanosystems for topical delivery of quercetin. Eur J Pharm Sci 12:1–43
- Cirri M, Bragagni M, Mennini N, Mura P. (2012). Development of a new delivery system consisting in “drug – in cyclodextrin in nanostructured lipid carriers” for ketoprofen topical delivery. Eur J Pharm Biopharm 80:46–53
- Curic ND, Fahr A. (2012). Liposomes in topical photodynamic therapy. Expert Opin Drug Deliv 9:1015–32
- Dougherty TJ, Gomer CJ, Henderson BW, et al. (1998). Photodynamic therapy. J Natl Cancer Inst 90:889–905
- Enk CD, Levi A. (2012). Low-irradiance red LED traffic lamps as light source in PDT for actinic keratoses. Photodermatol Photoimmunol Photomed 28:332–4
- Fang YP, Tsai YH, Wu PC, Huang YB. (2008). Comparison of 5-aminolevulinicacid-encapsulated liposome versus ethosome for skin delivery for photodynamic therapy. Int J Pharm 365 144–52
- Fazil M, Shadab M, Haque S, et al. (2012). Development and evaluation of rivastigmine loaded chitosan nanoparticles for brain targeting. Eur J Pharm Sci 47:6–15
- Ferdous R, Anisuzzaman SM, Paul AK, et al. (2012). In vitro release kinetic study of gliclazide from methocel k 100 mcr and methocel k100lvcr matrix tablets. Int J PharmTech Res 4:883–8
- Gasco MR. (1993). Method of producing solid lipid microspheres having narrow size distribution. US Patent 5250:236
- Hopper C. (2000). Photodynamic therapy: a clinical reality in the treatment of cancer. Lancet Oncol 1:212–19
- Kubler AC, Haase T, Staff C. (1999). Photodynamic therapy of primary nonmelanomatous skin tumours of the head and neck. Lasers Surg Med 25:60–8
- Lear JT, Harvey I, de Berker D, et al. (1998). Basal cell carcinoma. J R Soc Med 91:585–8
- Lee C, Qiao X, Goeger DK, Anderson KE. (2004). Fluorometric measurement of 5-aminolevulinic acid in serum. Clin Chim Acta 347:183–8
- Leiter U, Garbe C. (2008). Epidemiology of melanoma and nonmelanoma skin cancer – the role of sunlight. Adv Exp Med Biol 624:89–103
- Muchow M, Maincent P, Muller RH. (2008). Lipid nanoparticles with a solid matrix (SLN, NLC, LDC) for oral drug delivery. Drug Dev Ind Pharm 34:1394–405
- Muller RH, Mader K, Gohla S. (2000). Solid lipid nanoparticles (SLN) for controlled drug delivery – a review of the state of the art. Eur J Pharm Biopharm 50:161–77
- Oliveira FM, Santana DCA, Taveira SF, et al. (2010). Development of nitrosyl ruthenium complex loaded lipid carriers for topical administration: improvement in skin stability and nitric oxide release by visible light irradiation. J Pharm Biomed Anal 53:843–51
- Pierre MB, Ricci E, Jr, Tedesco AC, Bentley MV. (2006). Oleic acid as optimizer of the skin delivery of 5-aminolevulinic acid in photodynamic therapy. Pharm Res 23:360–6
- Rosa FSD, Tedesco AC, Lopez RFV, et al. (2003). In vitro skin permeation and retention of 5-aminolevulinic acid ester derivatives for photodynamic therapy. J Control Rel 89:261–9
- Song CK, Balakrishnan P, Shim CK, et al. (2012). A novel vesicular carrier, transethosome, for enhanced skin delivery of voriconazole: characterization and in vitro/in vivo evaluation. Colloids Surf B Biointerfaces 92:299–304
- Souza LG, Silva EJ, Martins ALL, Mota MF. (2011). Development of topotecan loaded lipid nanoparticles for chemical stabilization and prolonged release. Eur J Pharm Biopharm 79:189–96
- Tan Q, Liu W, Guo C, Zhai G. (2011). Preparation and evaluation of quercetin-loaded lecithin chitosan nanoparticles for topical delivery. Int J Nanomed 6:1621–30