Abstract
Abstract: Fluconazole-loaded niosomes were prepared by the film hydration method with different surfactants (Span and Brij series) and characterized for various parameters. Results showed that niosomes composed of Span 40, Span 60, and Brij 72 were most stable with smaller size, i.e. 0.378 ± 0.022 μm, 0.343 ± 0.063 μm, and 0.287 ± 0.012 μm, respectively, along with higher entrapment efficiency (approx. > 41%). In vitro skin permeation and retention studies suggested that cutaneous accumulation was affected by surfactant property and vesicle size. Therefore the niosomes consisting of Span 40, Span 60, and Brij 72 surfactant are seemingly accumulated and form localized drug depots in the skin, thereby releasing the contents in a sustained manner and able to greatly enhance cutaneous retention of the drug.
INTRODUCTION
During the past decades, niosomes (the vesicles composed of nonionic surfactant) have been developed as an alternative to conventional liposomes. In fact, they offer higher chemical stability, lower cost, and greater options of surfactant classes as compared to phospholipids vesicles [Citation1–3]. These vesicular systems are formed on hydration of a mixture of cholesterol (CH) and non-ionic surfactants such as alkyl ethers, alkyl esters, or alkyl amides [Citation4]. Moreover, alteration of the composition, i.e. type of surfactant used, can modulate the drug release rate and also the affinity of carriers to the target site [Citation5]. Niosomes also play an increasingly important role in drug delivery as they can reduce drug toxicity and modify drug pharmacokinetics and bioavailability. Interestingly, they emerged as an attractive drug delivery system, particularly in the treatment of dermatological disorders [Citation6]. Indeed topically applied niosomes can improve the penetration of drugs into the skin, and further increase the residence time of drug in the stratum corneum (SC) and epidermis, while reducing the systemic uptake of the drug [Citation6,Citation7]. Simultaneously, they serve as a local depot for the sustained and controlled release of dermally applied bioactive moiety [Citation7]. Previous studies demonstrated that, as compared to conventional formulations, niosomes depicted an enhanced cutaneous drug bioavailability [Citation3,Citation8].
In recent years, attention has been focused on niosomes made up of sorbitan ester (Span series) based surfactants for numerous identified applications. Therefore, in order to obtain a system composed of surfactants that are generally regarded as safe, the Span series has widely been investigated [Citation9]. The previous reports suggest that the caffeine incorporated in Span 60 based niosomes may be a promising carrier for caffeine, as compared to other Span 20, 40, and 80-based niosomes [Citation10]. In one of the reports, the Span 40 and 60 surfactant-based aceclofenac niosomes exhibited better stability and significant sustained anti-inflammatory activity as compared to the marketed product [Citation11]. Mokhtar et al. (Citation2008) prepared the niosomes of flurbiprofen using Span (20, 40, 60, 80) and concluded that type of surfactant could also influence the encapsulation efficiency as well as release profile of the drug [Citation12].
Currently, polyoxyethylene-alkyl-ethers-based niosomal systems are being investigated for specific drug delivery applications. These surfactants were studied to find a systematic correlation between variation in the length of alkyl chain and head group and drug entrapment efficiency and also the release kinetics [Citation13].
Hofland et al. (1991) introduced the vesicular systems composed of these surfactants for transdermal drug-carrier systems [Citation14]. They also studied permeation of estradiol from n-alkyl polyoxyethylene ether niosomes and observed that direct contact between niosomes and skin is an imperative to harness the highest effect on drug transport [Citation15]. Brij series (35, 52, 58, 72, 76, 92, and 97) based niosomes of recombinant human insulin were prepared. It was concluded that niosomes composed of Brij 92 were most stable with maximum protection activity, as they could effectively prolong the release of insulin in both SGF and SIF media [Citation10]. In one report, the incorporation of tretinoin in niosomes composed of Brij 30 and Span 40 and 60-based surfactants resulted in maximum protection of drug [Citation16]. Furthermore, Suwakul et al. demonstrated that propylthiouracil niosomes could readily be formed from Brij (52, 72, 76, 93, and 97) and Span (20, 40, 60, 80, and 83) based surfactants. The drug entrapment and the release rate recorded were seemingly dependent on the physical state of the bilayers [Citation17]. Minoxidil-loaded niosomal formulations were prepared by using CH and nonionic surfactants Brij 52, 76, and Span 20, 40, 60, and 80. The surfactant nature and vesicular size were found to play an important role in enhancing minoxidil bioavailability with topical delivery [Citation8].
Fluconazole, an antifungal drug, has effectiveness and potency against various raising pathogenic fungi, including C. albicans, which is a major contributory factor for cutaneous candidiasis [Citation18,Citation19]. It is commercially available in oral and parenteral dosage forms, which are largely confronted with well-known adverse effects including irritation of GIT as well as causing taste disturbances. Serious hepatotoxicity is generally precipitated in patients suffering from AIDS or malignancy [Citation20]. The use of topical fluconazole in the treatment of skin infection with various formulations including gel microemulsion [Citation21], gel formulation, [Citation22], lecithin-based organogel [Citation23], and hydrogel [Citation19] is recommended. Ideally, sustained drug release, cutaneous accumulation for localized effect in different strata of skin, and low extent of permeation of drug to the systemic circulation could be of obvious advantages for topical delivery. It has been reported that ketoconazole niosomes offer significant therapeutic advantage over plain drug solution [Citation24]. Ning et al. (Citation2005) also reported that clotrimazole-loaded liposomes and niosomes might be useful for skin targeting, providing localized effect [Citation25].
We have evaluated the surfactants for drug-loaded niosomes as topical delivery systems, which are capable of improving the cutaneous delivery of fluconazole. In this investigation, we report the ability of Span series and Brij series mixed with CH to form bilayered vesicles and encapsulate fluconazole. Furthermore, the niosomes were characterized for several parameters including shape and size, entrapment efficiency, release study, in vitro skin permeation, and retention studies of vesicles as well as their storage study.
MATERIALS AND METHODS
Materials
Fluconazole was provided as a gift from M/s Torrent Pharmaceuticals Ltd. (Ahmadabad, India). Span (20, 40, 60, and 80), Brij series (52, 72, 76), CH, and Dicetylphosphate (DCP) were purchased from Sigma Aldrich (St. Louis, USA). All other reagents and solvents were either of analytical or HPLC grade.
Preparation of Niosomes
Drug-loaded niosomes were prepared by using the thin film-hydration method as described previously with slight modifications [Citation5]. Accurately weighed quantities of the surfactant (Span or Brij) and CH in 7:3 molar ratio, with DCP in fixed amount 0.33 molar ratio, were dissolved in a chloroform: methanol mixture (2:1 v/v) in a round-bottom flask. The organic solvent was removed by using a rotary flash evaporator (Strike-102; Steroglass S.r.l., Perugia, Italy) under reduced pressure. The dried film was then hydrated with 5 ml phosphate buffered saline (PBS, pH 7.4) with a gentle rotation in a water bath maintained at 55°C for 30 minutes then subsequently left at room temperature for 6 hr for complete hydration. The niosomes were sonicated to reduce vesicles size using a probe sonicator (Soniweld, India) under an ice bath for 3 minutes. The final niosomal suspension was stored at refrigerator temperature for further studies.
Characterization of Fluconazole Niosomes
Prepared vesicles were characterized for shape by transmission electron microscopy (TEM; JEOL, Tokyo, Japan), using a copper grid coated with carbon film and with phosphotungstic acid (1%; w/v) as a negative stain. The vesicles size was determined by photon correlation spectroscopy using Zetasizer nano ZS90 (Malvern UK). The amount of drug entrapped in niosomal formulations was determined using the earlier reported method [Citation20]. Briefly, about 0.2 ml of niosomes was eluted with PBS through a Sephadex G-50 column to separate the unentrapped drug. The entrapped drug was determined by disrupting the vesicles using Triton X-100. The absorbance at 261 nm was measured by a UV spectrophotometer (Cintra 10, GBC Scientific).
Physical Stability of Vesicles at Storage Condition
Aggregation or fusion of the vesicles as a function of storage duration was determined as the changes in vesicle size and % entrapment efficiency were recorded. The vesicles were stored in glass vials in refrigerator (4 ± 1°C) for 2 months. Samples from each batch were withdrawn at definite time intervals and vesicle size and residual amounts of drug were determined as described previously.
In Vitro Release Study
The in vitro release study was performed with using the dialysis tube method as reported earlier with slight modification [Citation5]. Briefly, 1 ml of sample was taken into a dialysis tube (MWCO 10000, Sigma) and placed in a beaker containing 20 ml of PBS (pH 7.4) for a period of 12 hrs. The beaker was placed over a magnetic stirrer and the temperature of the assembly was maintained at 37 ± 1° C throughout the study. Samples were withdrawn at predetermined time intervals and assayed for drug content by measuring the absorbance at 261 nm against a reagent blank, using the UV spectrophotometer (Cintra 10, GBC Scientific). Sink conditions were maintained throughout the experiment. All the experiments were performed in triplicate (n = 3).
In Vitro Skin Permeation and Retention Studies
After cervical dislocation of the hairless rat skin, the abdominal and dorsal skin were excised surgically. The full thickness skin was used after removing underlying fat and subcutaneous tissues. The in vitro skin permeation study was carried out using a Franz diffusion cell, as described earlier with slight modification [Citation26]. The skin was mounted between the donor and receptor compartments of the diffusion cell with the SC facing upward (donar compartments). Each diffusion cell, with a diffusion area of 3.14 cm2 and the receptor medium, was filled with 25 ml of PBS (pH 7.4) thermostated at 37 ± 1°C, which was continuously stirred at 100 rpm throughout the experiment. 1 ml of formulation was placed on the donor compartment. At appropriate time intervals up to 12 hrs, samples of 200 μl from the receptor compartment were withdrawn and replaced by an equal volume of fresh medium to keep a stable receiver volume. The experiments were repeated in triplicate. The samples from the receptor compartment were analyzed by HPLC, as previously reported [Citation36]. In brief, the analytical column used was C18 (150 × 4.6 mm), protected by a C18 guard column (4.0 × 3.0 mm), the mobile phase was a mixture of 10 mM of sodium acetate buffer (adjusted to pH 5.0 with glacial acetic acid) and methanol (65:35) at a flow rate of 1 ml/min. The absorbance of the sample was detected at a wavelength of 210 nm. At the end of the permeation study, excess formulation was removed by wiping the test area with cotton swab, washed 3 times with PBS, and then dried with a filter paper. To determine the drug concentration in the SC, 20 tapes (CuDerm® Corporation, Adhesive Tape, New Delhi, India) were applied with constant pressure for 2 minutes and carefully peeled away following the method reported by CitationKim et al. (1997) [Citation27]. The tapes were pooled in a tube containing 2 ml of PBS and methanol mixture in 1:1 v/v ratio. Then, the tubes were vortexed for 20 minutes, sonicated for 30 minutes, and vortexed again for 1 hr to extract the drug from the tapes. The remaining skin was cut into small pieces to determine the amount of drug in the viable skin (epidermis and dermis). They were pooled in a tube containing 2 ml of PBS and methanol (1:1 v/v), vortexed for 20 minutes, and homogenized (York, Mumbai, India). The resulting solution was sonicated for 30 minutes, vortexed again for 30 minutes, and centrifuged at 10,000 g (Hitachi CPMax-100, Japan) for 30 minutes. The amount of drug extracted from tapes in remaining skin samples was determined by using HPLC.
Statistical Analysis
All the results are expressed as mean ± S.D. Statistically significant difference was determined using the Student's t-test and analysis of variance (ANOVA) with p < 0.05 as a minimal level of significance.
RESULTS AND DISCUSSION
Fluconazole is a potent antifungal agent. The drug-loaded niosomal formulations were prepared for topical delivery and investigated for the feasibility of skin drug localization and sustained drug delivery for local treatment. In the present study niosomes were prepared by the dry film hydration method by using different surfactants as Span and Brij series. The ability of the studied surfactants to form vesicles is summarized in . In this study, surfactant and CH were included at a fixed molar ratio and niosomes were stabilized by the addition of optimum quantity of a negative charged molecule such as DCP.
Table 1. Characterization of fluconazole niosomes prepared by thin film-hydration method at 7:3:0.33 molar ratio of surfactant: CH: DCP-vesicle size (μm), polydispersity index (PI), and percent entrapment efficiency (% EE) at different time intervals after preparation and storage at 4°C; each point represents the mean ± S.D. (n = 3).
Vesicle Shape and Size
TEM photographs suggest that vesicles obtained were of almost spherical shape and uniform in size, as shown in . The average size of the prepared niosomes is recorded in . Results indicate that size of vesicles is fully dependent on the composition of the bilayer and drug load. The results obtained with Span 20 (HLB 8.6), Span 40 (HLB 5.7), Span 60 (HLB 4.7), and Span 20 (HLB 4.3) based niosomes are shown in . The results reveal that the size of niosomes tended to increase with a progressive increase in the HLB value of Span used in the formulation. The smallest average size (0.319 μm) was measured in the case of Span 80 based vesicles while vesicles were of larger size, i.e. 0.448 μm in the case of Span 20 based niosomes. This might be due to surface-free energy as it decreases with increasing hydrophobicity [Citation9]. Khazaeli and Abbas Pardakhty (2007) [Citation5] reported that niosomes composed of sorbitan monoesters (Span 20, 40, and 60) were relatively larger in size as compared to Span 80 based niosomes. The similar results were reported for Span 85 (HLB 1.8) niosomes, which showed smaller size as compared to Span 20, 40, 60, and 80 based niosomes [Citation9]. Ruckmani et al. (Citation2000) [Citation28] showed that niosomes consisted of Span 60, Tween 80, and Tween 20 were bigger in size, while in the case of Span 80 niosomes the average size measured was relatively smaller, which may be due to lower HLB value.
Figure 1. TEM photograph of optimized fluconazole loaded systems vesicles prepared with (A) Span 40; (B) Span 60; (C) Brij 72.
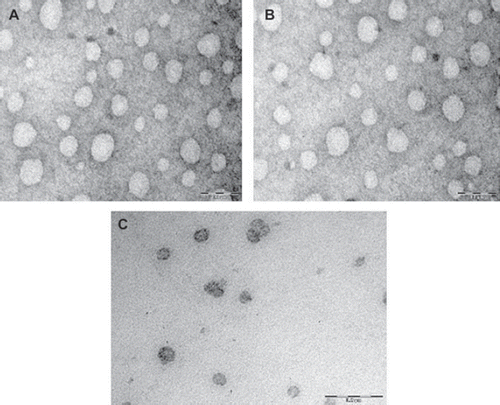
The results obtained with Brij 52 (HLB 5.3), Brij 72 (HLB 4.9), and Brij 76 (HLB 12.4) based niosomes are shown in . The lower vesicle size was measured in the case of Brij 72 niosomes (0.287 ± 0.012 μm), while in the case of Brij 52 and Brij 76 based niosomes the size measured was, i.e., 0.408 ± 0.054 μm, 0.443 ± 0.045 μm, respectively. The average smaller size in the case of Brij 72 based niosomes may be owing to lower HLB value. The data are indicative of regular increase in diameter as recorded for Brij 52 and Brij 76 based niosomes where the length of the alkyl chain of the used surfactant was sequentially of increased order. It may be because of thr constant polyoxyethylene head group with increasing alkyl chain length, which could have resulted in an increased vesicular size [Citation10,Citation15,Citation29]. Similar results were obtained in the case of vesicles composed of C16EO5/CH or C18EO5/CH [Citation30] and also vesicles composed of C12EO3, C14EO3, C16EO3, or C18EO3 [Citation29]. However, it was observed that Brij 76 based niosomes were not formed regular in shape and they were relatively larger in vesicle size. Inclusion of the negatively charged lipid DCP in the formulation, however, could prevent niosomes aggregation and hence imparted better stability to niosomes dispersions [Citation31]. The polydispersity index (PI) as shown in suggests a narrow size distribution. Among the formulations in the case of Span 40, Span 60, and Brij 72 based vesicles the PI was invariably low, 0.142, 0.121, and 0.134, respectively.
Entrapment Efficiency
The % drug entrapment efficiency is an important parameter and depends on nature of surfactants. Therefore, to explore the effect of chemical structure of surfactants on drug encapsulation efficiency, niosomal formulations using Span or Brij series were prepared using the same total lipid concentration. Results showed that % drug loading of Span series (20,40, 60, and 80) niosomes was 3.77%,4.44%, 4.41%, and 2.87%, respectively, while with Brij series (52, 72, and 76) niosomes the percent drug loading recorded was 4.78%, 5.05%, and 1.82%, respectively. The % drug entrapment efficiency recorded for various formulations was in the range of 24 to 43% as enlisted in . The data showed that Span 60 based niosomes has significantly higher entrapment efficiency, compared to Span 20 and 80 based formulations (P < 0.05). However, the insignificant difference in entrapment efficiency was in the case of Span 40 based niosomes (P > 0.05). This could be attributed to the chemical structure of surfactant, as increasing the alkyl chain length, which could have resulted in higher entrapment efficiency [Citation31]. The entrapment efficiency followed the trend Span 60(C18) > Span 40 (C16) > Span 20 (C12) > Span 80 (C18). It was previously reported that Span 20, Span 40, and Span 60 have the same head group and different alkyl chain, but Span 60 has the longest saturated alkyl chain, which could be responsible for highest entrapment efficiency. However, in the case of Span 80, it has an unsaturated alkyl chain with a double bond that causes the bend in chain. Therefore, the adjacent molecules are not packed compact, resulting in more permeable bilayer formation.
Later, the membrane may possibly explain low entrapment efficiency of the Span 80 based formulations. Therefore, the alkyl chain length plays an important role in the permeability behavior [Citation31]. In addition, Span 80 has the lowest transition temperature (Tc = − 12◦C) amongst all tested Spans (16°C for Span 20, 42°C for Span 40, and 53°C for Span 60) [Citation12]. The Span with the highest phase transition temperature thus provides the highest encapsulation for the drug and vice versa [Citation9,Citation31]. The similar results were also observed for Brij series based niosomes. The results showed that Brij 72 (Tc = 40°C) based niosomes have relatively higher encapsulation efficiency, while Brij 76 (Tc = 34°C) based niosomes showed comparatively low entrapment efficiency. This could be attributed to irregular structure(s), which are formed in the case of Brij 76 as well as its relatively low transition temperature [Citation4,Citation9,Citation29,Citation31].
Physical Stability Studies
Stable niosomal dispersion must maintain average particles size as well as drug content. In the present study, stability studies were conducted on selected formulations. They were stored at 4°C for a period of 2 months and observed for the leakage of encapsulated fluconazole and the change in average particle size of the vesicles ( and ).
Figure 2. Stability of drug-loaded niosomes stored at 4°C for 2 months. Values are expressed as mean ± S.D. (n = 3).
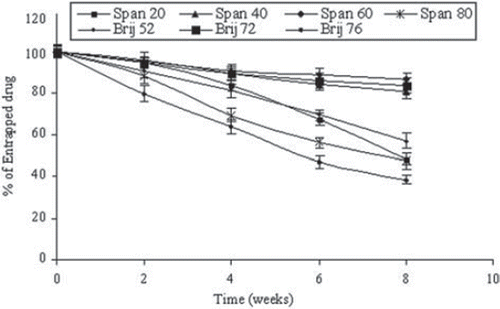
The data presented in showed the drug retained in niosomes stored at 4°C for 2 months. The % drug retained as measured was in the order Span 60 > Brij 72 > Span 40 > Brij 52 > Span 20 > Span 80 > Brij 76 (). It was observed that Span 60 niosomal formulations were most stable compared to other surfactant-based formulations. Our findings are in accordance with the findings of Gianasi et al. (Citation1997) [Citation32]. This may be attributed to the limited leakage of drug in the case of Span 60 surfactant-based formulation due to its high phase transition temperature and low permeability [Citation11]. The residual % drug content of niosomes formulations consisted of Span 20 and Span 80 was 32.52 ± 2.34% and 27.21 ± 2.06%, respectively, while average vesicle size was 0.448 ± 0.041 μm, 0.319 ± 0.026 μm, respectively. This could be due to their larger particle size and PI may have contributed to the lower stability of these systems [Citation1]. It was observed that in the case of Brij 72-based niosomes, the incorporation of charged molecules (DCP) in the bilayer composition or higher transition temperature of Brij72 resulted in an increased stability, as limited drug leakage during storage (as represented in and ) was recorded. It is previously reported that inclusion of charged molecules prevents the aggregation of niosomes and hence contributes to the stability of these dispersions [Citation2].
In Vitro Release Study
shows an initial rapid release of the drug and a subsequent slower release phase is invariably recorded. The rapid initial phase may be accounted to the leached drug in the dispersion medium, whereas subsequent slower release may be due to slow and controlled diffusion of drug through the bilayers. As expected, quantitative drug diffusion across the dialysis membrane as measured for different formulations was slower as compared to the plain drug solution (). The release profile after 12 hrs was more than 90% from the plain solution; however, only up to 31% of the entrapped drug released from niosomes consisted of Span 40 or Span 60. Brij 72 containing vesicles approx. 28% of loaded drug released. Statistically, there were insignificant differences between the cumulative % drug release recorded from Span 40, 60, and Brij 72 based niosomal formulations (p > 0.05).
Figure 3. In vitro release of fluconazole in PBS (pH 7.4) for different surfactant-based niosomal formulations. Values are expressed as mean ± S.D. (n = 3).
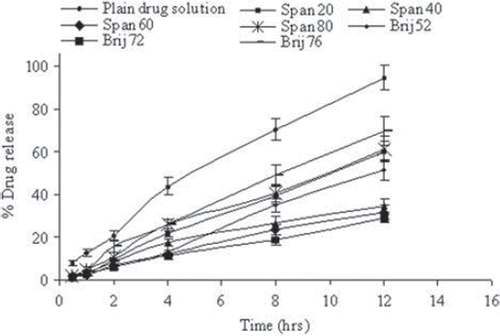
The release pattern revealed that drug release from Span 40 and Span 60 based niosomal formulations was a slower release than other Span-based formulations. It is worth noting that the Span 40 and Span 60 are ordered gel state systems while Span 20 and Span 80 are in the disordered liquid-crystalline state [Citation9,Citation33]. The release rate is also dependent on membrane fluidity, as a function of either acyl chain length and saturation or CH content [Citation34]. Similar results have been reported for insulin release [Citation35] as well as caffeine release [Citation5] from liquid state Span 20 and Span 80 based niosomes in comparison with gel state Span 40 and Span 60 niosomes. Brij 72 based niosomes, however, showed retarded drug release as compared to Brij 52 and Brij 76 based formulations. The faster drug release in the case of Brij 52 and Brij 76 based niosomes might be due to the shorter chain length and also relatively double bonds into the hydrocarbon chains cause a marked enhancement of vesicle permeability [Citation36]. It was observed that the release rate was dependent on the amount of entrapped drug in formulations. The formulation with higher fluconazole entrapment released the drug more slowly than the formulation with lower percent drug payload. The similar negative relationship between drug entrapment and drug release has been previously reported with propylthiouracil niosomes [Citation17], mitoxantrone liposomes, and acetazolamide niosomes [Citation37,Citation38].
In Vitro Skin Permeation and Retention Studies
To assess the influence of the drug carriers on the permeation and accumulation of drug into the skin, in vitro skin permeation and retention studies were performed using hairless rat skin by Franz diffusion cells. The permeation data obtained from different surfactant-based niosomal formulations were compared with a plain drug solution of equivalent drug concentration (i.e., 0.6 mg/ml). The results revealed that the amount of drug permeated through the skin in the initial period was higher. Afterwards with all vesicular formulations, permeation rate was slow (). Results seem to indicate that niosomes clearly delayed the drug permeation through the skin. This may be vesicular structure of niosomes, which may be responsible and rate limiting in free drug permeation across the skin [Citation29].
Figure 4. In vitro skin permeation of drug for different niosomal formulations. Values are expressed as mean ± S.D. (n = 3).
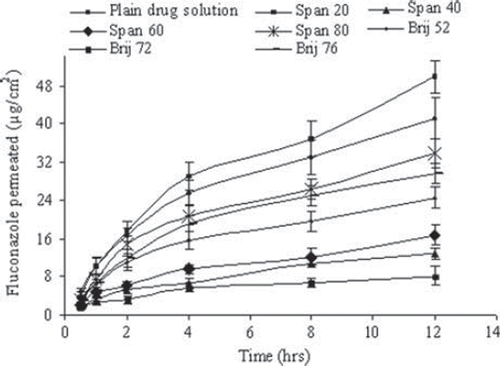
Results are recorded in and , which described the cumulative amounts of drug permeated in the receiver compartment at 12 hrs after the experiments. It is noticed that there has been significant differences in the cumulative amount of fluconazole permeated. The observed results indicated that the extent of permeated drug was dependent on the type of surfactant used in niosomal formulations. In fact, permeated amount increased in accordance with the order: Brij72 < Span40 < Span 60 < Brij52 < Brij 76 < Span 80 vesicles. One interesting fact was noticed: Span 20 based niosomes showed the higher extent of drug permeation as compared to control.
Table 2. Results of in vitro permeation and skin retention study from different fluconazole niosomal formulations: amount permeated through the skin at 12 hr, percent fluconazole accumulated into the skin at the end of the permeation experiments (12 hr), and Locally Accumulation Efficiency (LAC) value; drug accumulated into SC/Drug permeated through the skin ratio; the data were expressed as percent dose applied per unit area; each point represents the mean ± S.D. (n = 3).
The vesicular formulations could enhance drug accumulation in rat skin (to a factor of 1–2.94) over control formulation. Moreover, the higher accumulation of fluconazole recorded in the case of Brij 72 and Span 40 and 60 based vesicles was statistically significant (P < 0.05) as compared against the control.
Superior fluconazole skin penetration was obtained with smaller size Span 60 and Span 40 than niosomes prepared from Span 80, with an exception of Span 20 niosomes. The similar results were also seen in the case of the smaller size of Brij 72 based niosomes, which showed higher skin accumulation (31.18% ± 3.54) as compared to Brij 52 (22.83% ± 2.93) and Brij 76 (18.7% ± 2.44) niosomes. It appeared that niosomal vesicles composition and smaller size of these niosomes may be well correlated with higher skin penetration [Citation3,Citation8,Citation9]. The smaller vesicles may rapidly disintegrate on the skin surface, resulting in close contact between skin lipids and vesicle constituents. Finally, material exchange between vesicles and intercellular lipids may occur that may allow the diffusion of free drug molecules as well as small membranal fragments into the SC [Citation3]. The percent of fluconazole permeated through the skin and accumulated into the SC, as well as in viable skin as measured for all formulations at the end of the experiments, has been compiled in . The drug retention was maximal in SC invariably for all the formulations. A significantly higher SC retention of drug was obtained in the case of Brij 72, Span 40, and Span 60 based niosomes as compared with the control (P < 0.05). In fact, in the case of other formulations, no significant difference in drug concentration, especially in SC, was recorded. In the case of viable skin, it was also observed that Span 40, 60, and Brij 72 niosomes resulted in higher accumulation among all the formulations (P < 0.05) evaluated.
Figure 5. Percent fluconazole for different niosomal formulations for in vitro permeation and skin retention studies as mean ± S.D. (n = 3).
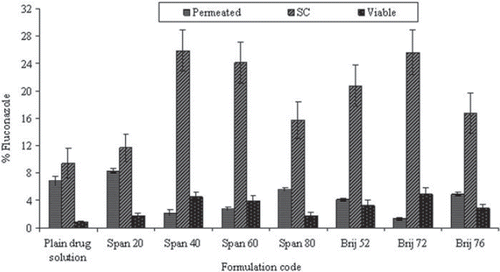
LAC value was calculated to determine the accumulation index of the drug [Citation39]. LAC values obtained from the several formulations are listed in . The LAC value of niosomes was higher by a factor of 1.4–18.7 than that of the control formulation (LAC = 1.4), suggesting that the main effect of these systems favors accumulating the drug in the skin. The only exception was Span 20, where the calculated LAC value (1.4) is almost equal to the control drug solution. It is interesting to appreciate the Span 20 based niosomal formulations showed the highest transdermal permeation as compared to the other vesicular preparations. Comparison of LAC values suggests that niosomes prepared using Span 40, 60, and Brij 72 were appreciably accumulated in the skin, hence may be considered as potential topical carriers for fluconazole, especially for the localized effect of the drug. The compa-rison of LAC values further suggests that the accumulation of the drug in the skin is affected by surfactant property as well as vesicle size. Therefore, the physico-chemical properties of amphiphiles transition temperature and HLB could be equally significant [Citation3].
Some interesting results obtained for Span 40 (HLB = 5.7), Span 60 (HLB = 4.7), and Brij 72 (HLB = 4.9) vesicles include highest retention in skin and lower permeation rate. However, Span 20 (HLB = 8.6), Brij 76 (HLB = 12.4), Brij 52 (HLB = 5.3), and Span 80 (HLB = 4.3) based niosomes showed higher permeation and as a consequence lower amount of drug accumulated in different strata of skin layers. Results of the study further confirmed that interaction between skin and vesicles mainly depends on physico-chemical properties of the major components of the vesicular bilayer.
CONCLUSION
In the present study, the various surfactant-based niosomal formulations for fluconazole were prepared. The findings revealed that fluconazole niosomes could readily form from various surfactants with suitable additives. The results concluded that the type of surfactant altered the entrapment efficiency and size as well as modified the drug release rate from niosomes. The in vitro skin permeation and drug retention in different strata of skin were also varying with use of different surfactant-based formulations. Permeation experiments have shown that the nature of surfactant and vesicular size play an important role in improving the cutaneous delivery of fluconazole. Niosomes composed of Span 40, 60, and Brij 72 surfactant are seemingly accumulated along with the drug into different strata of skin, thereby releasing the contents in a sustained manner. They appeared to penetrate and to form localized drug depots in the skin with subsequent sustained release of drug. Therefore, they have shown to be able to greatly enhance cutaneous retention of drug, and found to be most potentially useful for topical delivery providing localized effect.
Declarations of interest: The authors report no conflicts of interest. The authors alone are responsible for the content and writing of the paper.
REFERENCES
- Uchegbu, I.F., Florence, A.T. (1995). Non-ionic surfactant vesicles (niosomes)-physical and pharmaceutical chemistry. Adv Colloid Interface Sci, 58:1–55.
- Uchegbu, I.F., Vyas, S.P. (1998). Non-ionic surfactant based vesicles (niosomes) in drug delivery. Int J Pharm, 172:33–70.
- Manconi, M., Sinico, C., Valenti, D., Lai, F., Fadda, A.M. (2006). Niosomes as carriers for tretinoin III. A study into the in vitro cutaneous delivery of vesicle-incorporated tretinoin. Int J Pharm, 311:11–19.
- Manosroi, A., Wongtrakul, P., Manosroi, J., Saki, H., Sugawara, F., Yuasa, M., Abe, M. (2003). Characterization of vesicles prepared with various non-ionic surfactants mixed with cholesterol. Colloid Surf B: Biointerf, 30:129–138.
- Khazaeli, P., Pardakhty, A. (2007). Caffeine-loaded niosomes: Characterization and in vitro release studies. Drug Delivery, 14:447–452.
- Junginger, H.E., Hofland, H.E.J., Bouwstra, J.A. (1991). Liposomes and niosomes interactions with human skin. Cosmet Toil, 106: 45–50.
- Zakir, F., Vaidya, B., Goyal, A.K., Malik, B., Vyas, S.P. (2010). Development and characterization of oleic acid vesicles for the topical delivery of fluconazole. Drug Delivery, 17: 238–248.
- Balakrishnan, P., Shanmugam, S., Lee, W.S., Lee, W.M., Kim. J.O., Oh, D.H., Kim, D., Kim, J.S., Yoo. B.K., Choi, H.G., Woo, J.S., Yong, C.S. (2009). Formulation and in vitro assessment of minoxidil niosomes for enhanced skin delivery. Int J Pharm, 377: 1–8.
- Yoshioka, T., Strenberg, B., Florence, A.T. (1994). Preparation and properties of vesicles (niosomes) of sorbitan monoesters (Span 20, 40, 60, and 80) and a sorbitan triester (Span 85). Int J Pharm, 105:1–6.
- Pardakhty, A., Varshosaz, J., Rouholamini, A. (2007). In vitro study of polyoxyethylene alkyl ether niosomes for delivery of insulin. Int J Pharm, 328:130–141.
- Nasr, M., Mansour, S., Mortada, N.D., Elshamy, A.A. (2008). Vesicular aceclofenac systems: A comparative study between liposomes and niosomes. J Microencapsul, 25:499–512.
- Mokhtar, M., Sammour, O.A., Hammad, M.A., Megrab, N.A. (2008). Effect of some formulation parameters on flurbiprofen encapsulation and release rates of niosomes prepared from proniosomes. Int J Pharm, 361: 104–111.
- Bouwstra, J.A., van Hal, D.A., Hofland, H.E.J., Junginger, H.E. (1997). Preparation and characterization of nonionic surfactant vesicles. Colloids Surf B: Physicochem Eng Aspects, 123–124:71–80.
- Hofland, H.E.J., Bouwstra, J.A., Ponec, M., Boddé, H.E., Spies, F., Verhoef, J.C., Junginger, H.E. (1991). Interactions of non-ionic surfactant vesicles with cultured keratinocytes and human skin in vitro: A survey of toxicological aspects and ultrastructural changes in stratum corneum. J Cont Rel, 16:155–167.
- Hofland, H.E.J., Bouwstra, J.A., Gooris, G.S., Spies, F., Talsma, H., Juninger, H.E. (1993). Nonionic surfactant vesicles: A study of vesicle formation, characterization, and stability. J Colloid Interf Sci, 161: 366–376.
- Manconi, M., Valenti, D., Sinico, C., Lai, F., Loy, G., Fadda, A.M. (2003). Niosomes as carriers for tretinoin II. Influence of vesicular incorporation on tretinoin photostability. Int J Pharm, 260:261–272.
- Suwakul, W., Ongpipattanakul, B., Vardhanabhuti, N. (2006). Preparation and characterization of propylthiouracil niosomes. J Liposome Res, 16:391–401.
- Grant, S.M., Clissold, S.P. (1990). Fluconazole: A review of its pharmacodynamic and pharmacokinectic properties, and therapeutic potential in superficial and systemic mycoses. Drugs, 39:877–916.
- Abdel-Mottaleb, M.M.A., Mortada, N.D., El-Shamy, A.A., Awad, G.A.S. (2009). Physically cross-linked polyvinyl alcohol for the topical delivery of fluconazole. Drug Dev Ind Pharm, 35:311–320.
- Gupta, M., Goyal, A.K., Paliwal, S.R., Paliwal, R., Mishra, N., Vaidya, B., Dube, D., Jain, S.K., Vyas, S.P. (2010). Development and characterization of effective topical liposomal system for localized treatment of cutaneous candidiasis. J Liposome Res, 20:341–350.
- Laithy, H.M.E.I., EI-Shaboury, K.M.F. (2002). The development of cutina lipogels and gel microemulsion for topical administration of fluconazole. AAPS Pharmsci, 3:1–9.
- Bidkar, S., Jain, D., Padsalg, A., Patel, K., Mokale, V. (2007). Formulation development and evaluation of fluconazole gel in various polymer bases. As J Pharm, 1:63–68.
- Jadhav, K.R., Kadam, V.J., Pisal, S.S. (2009). Formulation and evaluation of lecithin organogel for topical delivery of fluconazole. Curr Drug Deliv, 6:174–183.
- Satturwar, P.M., Khandare, J.N., Nande, V.S. (2001). Niosomal delivery of ketoconazole. Ind Drugs, 38:620–624.
- Ning, M., Guo, Y., Pan, H., Chen, X., Zhongwei, G. (2005). Preparation, in vitro and in vivo evaluation of liposomal/niosomal gel delivery systems for clotrimazole. Drug Dev Ind Pharm, 31:375–383.
- Qiu, Y., Gao, Y., Hu, K., Li, F. (2008). Enhancement of skin permeation of docetaxel: A novel approach combining microneedle and elastic liposomes. J Cont Rel, 129:144–150.
- Kim, M.K., Chung, S.J., Lee, M.H., Cho, A.R, Shim, C.K. (1997). Targeted and sustained delivery of hydrocortisone to normal and stratum corneum-removed skin without enhanced skin absorption using a liposome gel. J Control Rel, 46:243–251.
- Ruckmani, K., Jayakar, B., Ghosal, S.K. (2000). Nonionic surfactant vesicles (niosomes) of cytarabine hydrochloride for effective treatment of leukemias: Encapsulation, storage, and in vitro release. Drug Dev Indus Pharm, 26:217–222.
- Tabbakhian, M., Tavakoli, N., Jaafari, M.R., Daneshamouz, S. (2006). Enhancement of follicular delivery of finasteride by liposomes and niosomes: 1. In vitro permeation and in vivo deposition studies using hamster flank and ear models. Int J Pharm, 323:1–10.
- Stafford, S., Baillie, A.J., Florence, A.T. (1988). Drug effects on the size of chemically defined non-ionic surfactant vesicles. J. Pharm. Phamracol, 40:26.
- Hao, Y., Zhao, F., Li, N., Yang, Y., Li, K. (2002). Studies on a high encapsulation of colchicine by a niosome system. Int J Pharm, 244:73–80.
- Gianasi, E., Cociancich, F., Uchegbu, I.F., Florence, A.T., Duncan, R. (1997). Pharmaceutical and biological characterization of a doxorubicin polymer conjugate (PK1) entrapped in sorbitan Span 60 niosomes. Int J Pharm, 148:139–148.
- Ladbrooke, B.D., Williams, R.M., Chapman, D. (1968). Studies on lecithin-cholesterol-water interactions by differential scanning calorimetry and X-ray diffraction. Biochem Biophys Acta, 150:333–340.
- Weiner, N.D., Williams, N., Birch, G., Ramachandran, C., Shipman, C., Flynn, G.L. (1989). Topical delivery of liposomally encapsulated interferone evaluated in a cutaneous herpes guinea pig model. Antimicrob Agents Chemother, 33:1217–1221.
- Varshosaz, J., Pardakhty, A., Hajhashemi, V., Najafabadi, A.R. (2003). Development and physical characterization of sorbitan monoester niosomes for insulin oral delivery. Drug Deliv, 10:251–262.
- De Gier, J., Mandersloot, J.G., Van deenen, L.L.M. (1968). Lipid composition and permeability of liposomes. Biochim Biophys Acta, 150:666–675.
- Law, S.L., Jang, T.F., Chang, P., Lin, C.H. (1994). Release characteristics of mitoxantrone-containing liposomes. Int J Pharm, 103:81–85.
- Guinedi, A.S., Mortada, N.D., Hathout, R.M. (2005). Preparation and evaluation of reverse-phase evaporation and multilamellar niosomes as ophthalmic carriers of acetazolamide. Int J Pharm, 306:71–82.
- Sinico, C., Manconi, M., Peppi, M., Lai, F., Valenti, D., Fadda, A.M. (2005). Liposomes as carriers for dermal delivery of tretinoin: Invitro evaluation of drug permeation and vesicle-skin interaction. J Control Rel, 103:123–136.