Abstract
Crosslinked graft copolymers of CMC with N-vinyl-2-pyrrolidone (N-VP) were prepared in different grafting yields. Saccharomyces bayanus was immobilized in the graft copolymers of carboxymethylcellulose-g-poly(N-vinyl-2-pyrrolidone) (CMC-g-PVP) for ethanol fermentation. The effects of grafting yield, initial glucose concentration, and crosslinker concentration on the yield of ethanol process was investigated. Ethanol production increased when percentage of N-vinyl-2-pyrrolidone in the graft copolymer was increased. The maximum concentration, productivity, and yield of ethanol were 98.78 g L −1, 8.23 g L −1 h −1 and 0.494 g g −1, respectively. Reusability of the immobilized yeasts was found that the materials can be used seven times without losing their activity.
Introduction
People’s energy needs are increased every day with the rapid growth of world population and development of technology. In recent years, the global crude oil reserve has proved to be finite while the depletion is occurring much faster than previously predicted (Phisalaphong et al. Citation2007). Due to the diminishing fossil fuel reserves, alternative energy sources need to be renewable, cleaner, sustainable, efficient, cost-effective, convenient, and safe. In this context, the use of ethanol as an alternative energy source has widened. Ethanol is a useful energy source and has various beneficial properties, such as a trace amount of net CO2 accumulation into the atmosphere and lower emissions compared with oil. Bioethanol is the most commonly used biofuel in the transportation sector worldwide. Ethanol is the most widely used biofuel either as a fuel or in gasoline blends. Using bioethanol-blended gasoline fuel in vehicles can significantly reduce petroleum use and greenhouse gas emission (Balat and Balat Citation2009, Fukuda et al. Citation2009).
Bioethanol production by immobilized cultures has advantages according to the free culture that is shown in the literature. Cell immobilization is mainly due to the numerous advantages that this offers, including enhanced fermentation productivity, feasibility of continuous processing, ease of filtration, cell stability, and lower costs of recovery and recycling and downstream processing (Phisalaphong et al. Citation2007, Behera et al. Citation2011, Ghorbani et al. Citation2011, Kourkoutas et al. Citation2004, Zhang et al. Citation2009). Many methods, namely adsorption, covalent bonding, crosslinking and entrapment, are widely used for immobilization. Entrapment has been one of the most used methods for whole cell entrapment due to its simplicity and non-toxic character (Ghorbani et al. Citation2011). Until now, many types of natural and inorganic support materials such as sodium alginate beads (Najafpour et al. Citation2004), sorghum bagasse (Yu et al. Citation2007), zeolite (Shindo et al. Citation2001), grape skin (Mallouchos et al. Citation2002), glass beads (Arasaratnam and Balasubramaniam Citation1998), chrysotile (Joekes et al. Citation1998), and agar (Behera et al. Citation2010, Lebeau et al. Citation1997) for yeast immobilization have been used for ethanol production.
The most widely used microorganisms, yeasts and bacteria, can be used in ethanol fermentation. Yeasts of the Saccharomyces genus are the most suitable for achieving a high yield and due to its potential ability to hydrolyze into fermentable sugars (Fukuda et al. Citation2009, Rojey and Monot Citation2010). Saccharomyces cerevisiae and Saccharomyces bayanus were interspecific hybrids. These hybrids have a different mitochondrial DNA (Serra et al. Citation2005). The yeast species Saccharomyces cerevisiae, Saccharomyces bayanus, and Saccharomyces pastorianus are of industrial importance since they are involved in the production process of common beverages such as wine and lager beer (Dellaglio et al. Citation2003, Horn et al. Citation2000, Naumova et al. Citation2005).
Carboxymethylcellulose (CMC), the most popular and cheapest cellulose derivative, is an anionic linear polymer. Due to its good biocompatibility, biodegradability, high water absorption ability, good degradability, harmlessness and low cost, CMC has been widely used in many fields (Qiu et al. Citation2007, Yiğitoğlu et al. Citation2007).
N-vinyl-2-pyrrolidone (N-VP) is a hydrophilic and nonionic monomer, the polymerization of which is easily initiated through radicals (Liu et al. Citation2004). Poly(N-vinyl-2-pyrrolidone) (PVP) is a polymer with great potential applications in different biomedicines. The principal reason for successful PVP application is its good biocompatibility with living tissues and extremely low cytotoxicity (Yiğitoğlu et al. Citation2007).
In our previous study (Yiğitoğlu et al. Citation2007), carboxymethylcellulose-g-poly(N-vinyl-2-pyrrolidone) copolymers (CMC-g-PVP) were synthesized, and the reaction conditions for grafting were optimized.
Modified CMC or other grafted copolymers have been used in controlled drug delivery (Iş klan et al. 2011), enzyme immobilization (Dalla-Vecchia et al. Citation2005), removing toxic metal ions (Zohuriaan-Mehr et al. Citation2004), etc. It is considered worthwhile to investigate the immobilization of Saccharomyces bayanus on CMC-g-PVP for ethanol production.
Materials and Methods
Materials
All chemicals and media ingredients were of analytical grade and of bacteriological grade, respectively.
Sodium carboxymethylcellulose (CMC) with a viscosity of 400–800 cps (2% aqueous solution at 25°C) was purchased from Sigma (St. Louis, MO). Glucose and N-vinyl-2- pyrrolidone (N-VP) were supplied by Fluka Chemie AG (Buchs, Switzerland) and N-VP purified by vacuum distillation at 2 mmHg and 65°C. Peptone and agar were obtained from Lab M (England). Sodium potassium tartrate, yeast extract, azobisisobutyronitrile (AIBN), and other chemicals were obtained from Merck AG (Germany).
The yeast strain used in these studies was Saccharomyces bayanus (Food Engineering Culture Collection of Ankara University, Turkey). The culture was maintained by transferring to fresh agar medium each month and stored at 4°C. The agar medium contained (g/L) malt extract 3.0, yeast extract 3.0, peptone 5.0, glucose 10.0, and agar 15.0. A fresh slant culture (24 h) was used for the cultivation of yeast biomass in liquid culture for use in immobilization process.
Graft copolymerization
Grafting procedure was carried out as in our previous study (Yiğitoğlu et al. Citation2007). A specific amount of CMC was dissolved in distilled water (50 mL) and bubbling of a slow stream of nitrogen was passed for 30 min. N-VP was dissolved in 25 mL distilled water and mixed with the CMC solution. Then, AIBN at the required concentration in 2 mL of acetone was added slowly to the reaction mixture, and the total volume of the reaction mixture was made up to 100 mL with distilled water. Grafting reaction was carried out at 70°C for 2 h.
Elemental analysis
Elemental analysis (C, H, N) of the graft copolymers with various graft yields were performed with a Leco CHNS-932 (Leco Corporation, St. Joseph, MI, USA) analyzer. The sample (2 mg) was burned at 1000°C in the silver capsules in an oxygen-rich environment. The products of combustion in a CHNS analysis (CO2, H2O, and N2) were carried through the system by He carrier gas. The combustion products (CO2, H2O) were determined quantitatively by means of a non-dispersive IR absorption detection system, except for the N2, which was measured by the thermal conductivity detector.
Preparation of CMC beads and CMC-g-PVP beads
Solution of CMC (2%(w/v)) and solution of CMC-g-PVP (3%(w/v)) were prepared in deionized water. These solutions were added dropwise with pipette to aluminum chloride solutions of different concentrations separately. Polymer drops solidified upon contact with AlCl3, forming beads. The beads were allowed to harden for 30 min and then were washed with distilled water.
Characterization of beads
Swelling Assay
CMC beads and CMC-g-PVP beads were swelled in nutrient medium of yeast cells at 30°C to discover parameters of swelling. Swollen beads were dried perfunctorily with filter paper, weighed, and placed in the same bath. The percentage of swelling degrees (SD) of the beads of CMC and beads of CMC-g-PVP crosslinked with AlCl3 in the fermentation medium (at 30°C) were calculated as follows:
Where mt is the mass of swollen beads at time t and mo is the mass of the dry beads.
Scanning Electron Microscopy (SEM)
SEM photographs were taken with a JSM (Tokyo, Japan) 5600 scanning microscope to examine the morphology, surface structure, and inside structure of CMC and N-VP grafted CMC, yeast-immobilized CMC, and yeast-immobilized CMC-g-PVP at the required magnification at room temperature. The beads were deposited on a brass hold and sputtered with a thin coat of gold under vacuum. Acceleration voltage used was 20 kV with the secondary electron image as a detector.
Physical Properties of Beads
In order to estimate the size of the beads before and after fermentation, 10 samples of the beads from different formulations were selected and their sizes were measured using an electronic digital caliper (Mitutoyo IP.65, Japan). The pore size of dry beads was determined by application of the Brunauer, Emmett and Teller (BET) method to the adsorption data by ASAP 2020M (USA) apparatus. In addition, pore volume was calculated from the amount of water adsorbed into the swollen beads.
Immobilization of Yeast Cells onto the Beads
The growth medium was prepared and sterilized at 121°C for 15 min, the pH of which was adjusted and contained: glucose 5%, yeast extract 1%, pepton 1%, and MgSO4 7H2O 1%. Then, Saccharomyces bayanus cells were grown in a growth medium at 30°C and 125 rpm for 18 hours on a rotary shaker. Yeast suspension was centrifuged (3500 rpm, 5min) and suspended in sterile saline solution (0.9%, NaCl).
CMC (2% w/v) or CMC-g-PVP (3% w/v) powders were dissolved in distilled water and sterilized in an autoclave for 10 min at 121°C. The yeast suspension was added to CMC and CMC-g-PVP copolymers, separately. The mixture with CMC and the mixture with CMC-g-PVP copolymers were added separately dropwise into 0.1 to 0.5 mol/L of AlCl3 solutions. Polymer drops solidified upon contact with AlCl3, forming beads. Thus the beads entrapped the yeast cells. The beads were allowed to harden for 30 min and then were washed in sterile saline solution (0.9%, NaCl) to remove excess aluminum ions and yeast cells. To increase the entrapped cell population, the beads were incubated overnight in the growth medium and under the same conditions.
Fermentation experiments
A synthetic fermentation medium containing 100.0 g/L of glucose, 5.0 g/L of yeast extract, 5.0 g/L of pepton, and 1.0 g/L of MgSO4.7H2O was sterilized and its pH was adjusted to 5.0 prior to fermentation in all cases (Liu et al. Citation2009, Liu and Shen Citation2008, Winkelhausen et al. Citation2010).
The fermentation was carried out using glucose concentrations ranging from 50 to 200 g L−1 to investigate the effect of glucose concentration on ethanol production.
Crosslinker concentration in the beads, ranging from 0.1 to 0.5 M, was used in fermentation to determine the effect of crosslinker concentration on ethanol production.
To determine the reuse availability of the yeast-immobilized beads, the beads were incubated in 100.0 g L−1 of glucose, 5.0 g L−1 of yeast extract, 5.0 g L−1 of pepton, and 1.0 g L−1 of MgSO4.7H2O containing fermentation medium for 12 hours at 30°C and 125 rpm. At the end of each fermentation process, the beads were washed with a sterile saline solution and the fermentation was repeated with fresh medium.
All the samples were taken during the fermentation process at certain time periods from the fermentation medium. These samples were used in the analysis of ethanol and glucose.
Analytical Procedures
The reducing glucose was analyzed by the DNS method (Miller Citation1959). Ethanol production in the fermentation process was detected through gas chromatography, HP 5890 series, (Hewlett-Packard, PA, USA) equipped with flame ionization detector (FID) and GC column DB-FFAP (30 m × 0.32 mm, 0.5µm film, 40–250°C) (Agilent Technologies, Inc., SC, USA). The oven and detector temperature were 190 and 200°C, respectively. Nitrogen was used as a carrier gas and its flow rate was 1.2 ml/min. The ethanol concentration was quantified using a calibration curve prepared by injecting different concentrations of ethanol standard.
Results and Discussion
Characterization of beads
The results of the elemental analysis for CMC and CMC-g-PVP with three different grafting yields (GY) are presented in . As is seen in , GY was increased with increase in the concentration of the monomer. Similar results have been reported by other researchers (Gao et al. Citation1998, Işiklan et al. Citation2008).
Table I. Grafting yield and elemental analysis results for CMC and grafted CMC.
The copolymers of three different grafting yields were selected from grafting experiments. The grafting yields of these copolymers were 20% (CMC-g-PVP1), 24% (CMC-g-PVP2), and 32% (CMC-g-PVP3). These copolymers and ungrafted CMC were used in all experiments.
The equilibrium swelling degree curves of CMC and CMC-g-PVP beads in the fermentation medium are shown in . The swelling degree increased with time and reached a constant value after a certain point. The highest swelling degree occurred in the CMC-g-PVP3 beads while the lowest swelling degree took place in the CMC beads (). It was determined that the swelling degree increased as the amount of the PVP in copolymer was increased. A highly hydrophilic polymer, PVP, facilitates the diffusion of the substrate and product. Similar observations have been found in other studies (Işiklan et al. Citation2011, Dalla-Vecchia et al. Citation2005). According to Katime et al. (Citation2001), equilibrium water content of poly(N-isopropylacrylamide-coitaconic acid) hydrogels increased from 68.33 to 97.64 (%) with percentage of itaconic acid increasing from 5 to 20%.
SEM photographs of a cross-section of Saccharomyces bayanus immobilized CMC and CMC-g-PVP beads taken at 500 times magnifications are shown in . By comparing the surface morphology of internal structure of CMC-g-PVP beads () with that of CMC beads (), it was found that the grafted chains drastically changed the morphology of CMC.
Table II. Physical properties and immobilization yield of cell immobilized beads.
Physical properties of beads were investigated, as shown in . Bead diameters of the CMC-g-PVP beads were larger than that of CMC beads. This increase is due to the PVP in copolymer. The swelling degree increased as the amount of the PVP in copolymer was increased. Thus, the bigger beads become consistent. In addition, the diameters of beads were increased with the increase of Saccharomyces bayanus cell density, which indicates that Saccharomyces bayanus had grown within the beads. Beads diameter increased after fermentation due to swelling of the beads.
The pore volume of beads was calculated from the amount of water adsorbed into the swollen beads. It was observed that pore volume of beads increased with increasing of GY.
Immobilization yield of beads was calculated after the fermentation as follows:
Immobilization yield was increased with the increased pore volume because of the greater amount of entrapped cells. The total dry cell concentration of the immobilized system with CMC-g-PVP3 was 7.8 g L−1 with an immobilization yield of 89%. This data supports the published report on the need for immobilization yield for fermentation by S. cerevisiae (Phisalaphong et al. Citation2007).
Fermentation experiments
CMC-g-PVP3 beads were used to determine the change of ethanol production with glucose consumption. Standard fermentation was carried out at pH 5.0 and 30°C temperature. The initial pH of culture madia was adjusted to 5.0 as the most suitable pH for the ethanol fermentation process in the literature (Liu et al. Citation2009, Winkelhausen et al. Citation2010).
When pH is below 4.5, the yeast cells failed to convert the carbon source to ethanol. When the pH of the extracellular environment is low to a certain extent, acetic acid would mainly exist in its undissociated form. Undissociated organic acid was more easily able to pass through cell membranes than its dissociated form; thus it would cause inhibition to the fermentability of microorganism (Liu and Shen Citation2008, Zhao and Xia Citation2010). Fluid samples were taken from the fermentation medium at particular times during the fermentation process. These samples were used in the determination of the amount of ethanol production and glucose consumption. Glucose was completely consumed in all fermentation processes.
The fermentation process consumes glucose and other fermentable sugars to produce ethanol, and this scenario was observed as shown in . Glucose (200 g L−1) was consumed in 12 hours and 99 g L−1 of ethanol was produced during this fermentation. Ethanol productivity and ethanol yield were obtained at 8.23 g L−1 h−1 and 0.494 g g−1, respectively. The reduction in glucose concentration occurred over time during the fermentation process.
Figure 3. Change of ethanol production with glucose consumption when CMC-g-PVP3 was used with the initial 200 g/L of glucose concentration.
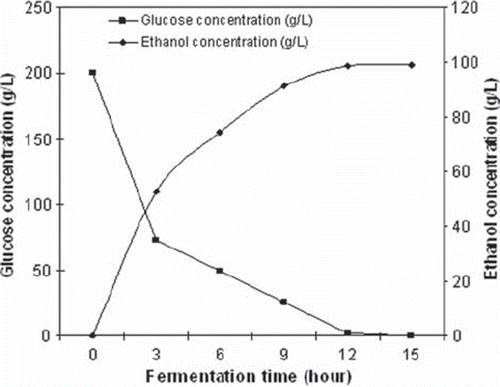
Table III. Ethanol production and cell concentration results of cell immobilized beads
Effect of grafting yield on ethanol production
The effect of N-VP percent in copolymer on ethanol production was investigated. When a CMC-g-PVP3 copolymer was used, ethanol production was found to be the highest. While maximum ethanol production was 92.12 g L−1 when CMC was used, the values were 94.43, 96.11, and 98.78 g L−1 when CMC-g-PVP1, CMC-g-PVP2, and CMC-g-PVP3 were used, respectively (). The hydrophilic groups of the CMC-g-PVP copolymers increased as PVP content was increased, which means an increase in the grafting yield. Thus, the swelling of the beads was increased. The higher the swelling of the beads, the higher the diffusion of the nutrient medium. In this way, the production of ethanol was increased as 7.6%.
The final ethanol concentration, yields, and productivity of ethanol are presented in . Ethanol productivity and ethanol yield calculations made according to the following equations:
The ethanol productivity of cell immobilized beads was shown to be approximately in the range of 7.87–8.23 g L−1 h−1 for the grafted copolymers and 7.60 g L−1 h−1 for the CMC. Ethanol productivity was accelerated because the CMC polymer was modified with N-vinyl-2-pyrrolidone. The degree of swelling was increased with grafting of N-vinyl-2-pyrrolidone onto CMC and, as a result of this, easier diffusion of nutrients into the porous beads was enabled, which promoted growth of yeast cells and increased ethanol productivity.
Effect of initial glucose concentration on ethanol production
The fermentation processes were carried out using initial glucose concentrations ranging from 50 to 200 g L−1 to investigate the effect of initial glucose concentration on ethanol production. When the initial glucose concentration was used at 50, 100, 150, 200 g L−1, glucose was consumed in 3, 6, 9, and 12h (fermentation time), respectively. The maximum ethanol concentration produced increased linearly with increasing initial glucose concentration (from 22.8 g L−1 ethanol with 50 g L−1 glucose to 98.78 g L−1 ethanol with 200 g L−1 glucose for yeast immobilized on CMC-g-PVP3 beads). There is the same rising for other yeast immobilized on beads. The results of fermentations are summarized in .
Table IV. The effect of crosslinker concentration on ethanol productivity.
The ethanol productivity increased with increasing GY of copolymers (). The highest ethanol productivity was found to be 8.23 g L−1 h−1 for CMC-g-PVP3 beads; the lowest ethanol productivity was found to be 7.27 g L−1 h−1 for CMC beads. The ethanol productivity of all grafted CMC was higher than ungrafted CMC. A similar observation was also found in the previous report with S. cerevisiae (Behera et al. Citation2010, Nikolic et al. Citation2009).
Grafted CMC beads provided greater ethanol production than ungrafted beads. As the amount of N-VP in copolymers increased, the swelling degree also increased, thus increasing the ethanol production. In other words, PVP-grafted CMC beads gave higher yields than CMC beads. Bead size increased with the increasing of GY in CMC-g-PVP copolymers. The size of the beads increased also because the yeast grown within the solid beads caused an increase in the density of yeast cells.
In our previous study (Gökgöz and Yiğitoğlu Citation2011), we immobilized S. cerevisiae onto CMC-g-PVP beads for ethanol production. While the ethanol production was 59.3 g L−1, the highest ethanol productivity was found to be 2.25 g L−1 h−1. In this study, when we used a different microorganism (Saccharomyces bayanus), ethanol productivity was increased from 2.25 to 8.23 g L−1 h−1 and fermentation time was decreased from 27h to 6h for 100 gL−1 of glucose concentration. Therefore, we can say that Saccharomyces bayanus was more productive than S. cerevisiae for ethanol production in our condition.
Similar results have been reported in the literature. Nabais et al. (Citation1988) investigated the effect of calcium ion on ethanol production of Saccharomyces bayanus and alcoholic fermentation by yeasts. While optimal calcium ion concentration was 0.75 M, they had produced 13–17% (v/v) ethanol from 300 g L−1 of glucose. Barros et al. (Citation1987) immobilized Saccharomyces bayanus on teflon for ethanol production. They had produced a range of 61–75 g/L ethanol. The ethanol productivity and ethanol yield were obtained as 1.2 g L−1 h−1 and 0.46 g g−1, respectively. Castellar et al. (Citation1998) studiedthe ethanol production by Saccharomyces bayanus of the effect of zeolite concentration. Zeolite NaY at 5 g L−1 concentration was selected to improve the production of ethanol fermentation from high glucose concentration media. The highest ethanol productivity (3.07 g L−1 h−1) and ethanol yield (0,43 g g−1) were obtained from a 220 g L−1 initial glucose concentration, while the highest ethanol concentration (130 g L−1) was obtained from a 350 g L−1 glucose medium. Nikolic et al. (Citation2009) investigated the batch fermentation of corn meal hydrolizates by free and immobilized cell of S. cerevisiae with different initial glucose concentration. They reported that ethanol pruduction was 8.9% (w/w) using 176 g L−1 initial glucose concentration by Ca-alginate immobilized S. cerevisiae. Ethanol productivity was determined in the range of 1.19–2.26 g L−1 h−1 while fermentation time was in the range of 26–74 h. Zhao and Xia (Citation2010) immobilized recombinant S. cerevisiae on Ca-alginate beads for production of ethanol. In this study, ethanol was produced at 21.8 and 31.1 g L−1 from 50.4 and 71.8 g L−1 of initial xylose concentration, respectively. Laouni et al. (2007) immobilized S. cerevisiae on Ca-alginate-chitosan beads. They had produced 5.83% (v/v) and 11.61% (v/v) ethanol from 100 g L−1 and 200 g L−1 of glucose, respectively.
Effect of crosslinker concentration on ethanol production
The yeast blended with CMC and the yeast blended with CMC-g-PVP copolymers were crosslinked with different concentrations of AlCl3 (0.1, 0.3, and 0.5 M). Yeast immobilized beads were incubated in fermentation medium (containing 100 g L−1 glucose) under the same conditions.
The crosslinker concentration has a great effect on pore size of the beads. When its concentration increases, polymer chains attach to each other more strongly and the size of pores during bead formation is smaller. Furthermore, chain flexibility decreases, resulting in reduced swelling capacity of the copolymer. Consequently, as shown in , the lower the amount of crosslinker concentration in the beads is, the higher the ethanol productivity will be.
Reusability of Saccharomyces bayanus immobilized beads
To investigate reusability, Saccharomyces bayanus immobilized CMC-g-PVP3 beads were reused in a fermentation medium. Fermentation duration was limited to 15h. The beads were washed with sterile isotonic water and were added to a new fermentation medium. Ethanol concentration was measured in the medium after each fermentation process. The results of reusability are summarized in . In the seven fermentation processes, the decrease in the production of ethanol is not very significant and the ethanol production decreased to about 4–6%. Similar results were found in all fermentation processes with different GY of beads. There is a good agreement between the reusability of the present work with the published paper (Behera et al. Citation2010, Zhao and Xia Citation2010).
Conclusions
In this study, Saccharomyces bayanus, immobilized by entrapping them in the beads of CMC and CMC-g-PVP copolymers, were successfully developed and applied to ethanol fermentation. The hydrophilic characteristic of CMC was increased by the addition of N-VP into the structure of CMC and the new polymeric matrix was compared with CMC and showed better mass transfer characteristics. This is because the equilibrium swelling degree of the new copolymer is higher than that of CMC. This property of the CMC-g-PVP matrix facilitated the growth of cells and diffusion of substrate and products and so, compared with CMC, it increased productivity and yield of ethanol. The highest ethanol production was obtained as 99 g L21 with the yeast immobilized on CMC-g-PVP3 supports when the medium contained 200 g L−1 glucose. The ethanol productivity and ethanol yield were found to be 8.23 g L−1 h−1 and 0.494 g g−1, respectively. Yeast immobilized beads may be reused seven times without losing their activity.
To sum up, these results show that CMC-g-PVP copolymers of different grafting yields can be used as support materials for production of ethyl alcohol by immobilization of yeast cells. These immobilized systems could be a useful model for ethanol production in commercial reactors as an alternative energy source.
Declaration of interest
The authors report no conflicts of interest. The authors alone are responsible for the content and writing of the paper.
References
- Arasaratnam V, Balasubramaniam K. 1998. The use of monochloroacetic acid for improved ethanol production by immobilized Saccharomyces cerevisiae. World J. Microb. Biot. 14: 107–111.
- Balat M, Balat H. 2009. Recent trends in global production and utilization of bio-ethanol fuel. Appl. Energ. 86: 2273–2282.
- Barros MR, Cabral JMS, Novais JM. 1987. Production of ethanol by immobilised Saccharomyces bayanus in an extractive fermentation process. Biotechnol. Bioeng. 29: 1097–1104.
- Behera S, Kar S, Mohanty RC, Ray RC. 2010. Comparative of bio-ethanol production from mahula (Madhuca latifolia L.) flowers by Saccharomyces cerevisiae cells immobilized agar agar and Ca-alginate matices. Appl. Energ. 87: 96–100.
- Behera S, Mohanty RC, Ray RC. 2011. Ethanol production from mahula (Madhuca latifolia L.) flowers with immobilized cells of Saccharomyces cerevisiae in Luffa cylindrica L. Sponge discs. Appl. Energ. 88: 22–215.
- Castellar R, Barros MR, Cabral JMS, Iborra JL. 1998. Effect of zeolite addition on ethanol production from glucose by saccharomyces bayanus. J. Chem. Technol. Biotechnol. 73: 377–384.
- Dalla-Vecchia R, Sebrão D, Dascimento MG, Soldi V. 2005. Carboxymethylcellulose and poly(vinyl alcohol) used as a film support for lipases immobilization. Process Biochem. 40: 2677–2682.
- Dellaglio F, Zapparoli G, Malacrinò P, Suzzi G, Torriani S. 2003. Saccharomyces bayanus var. uvarum and Saccharomyces cerevisiae succession during spontaneous fermentations of Recioto and Amarone wines. Ann. Microbiol. 53: 411–425.
- Fukuda H, Kondo A, Tamalampudi S. 2009. Bioenergy: Sustainable fuels from biomass by yeast and fungal whole-cell biocatalysts. Biochem. Eng. J. 44: 2–12.
- Gao J, Li Z, Wang W, Huang M. 1998. Preparation of gelatin– Nvinylpyrrolidone graft copolymer. J. Appl. Polym. Sci. 68: 1485–1492.
- Ghorbani F, Younesi H, Sari AE, Najafpour G. 2011. Cane molasses fermentation for continuous ethanol production in an immobilized cell reactor by Saccharomyces cerevisiae. Renew. Energ. 36: 503–509.
- Gökgöz M, Yiğitoğlu M. 2011. Immobilization of Saccharomyces cerevisiae on to modified carboxymethylcellulose for production of ethanol. Bioprocess Biosyst. Eng. 34: 849–857.
- Horn SJ, Aasen IM, Ostgaard K. 2000. Ethanol production from seaweed extract. J. Ind. Microbiol. Biot. 25: 249–254.
- Işiklan N, İnal M, Kurşun F, Ercan G. 2011. pH responsive itaconic acid grafted alginate microspheres for the controlled release of nifedipine. Carbohyd. Polym. 84: 933–943.
- Iş klan N, İnal M, Yiğitoğlu M. 2008. Synthesis and characterization of poly(N-vinyl-2- pyrrolidone) grafted sodium alginate hydrogel beads for the controlled release of indomethacin. J. Appl. Polym. Sci. 110: 481–493.
- Joekes I, Moran PJL, Rodrigues JAR, Wendhausen R, Tonella E, Cassiola F. 1998. Characterization of Saccharomyces cerevisiae immobilized onto chrysotile for ethanol production. J. Chem. Technol. Biotechnol. 73: 54–58.
- Katime I, Valderruten N, Quintana JR. 2001. Controlled release of aminophylline from poly(N-isopropylacrylamide-co-itaconicacid) hydrogels. Polym. Int. 50: 869–874.
- Kourkoutas Y, Bekatorou A, Banat IM, Marchant R, Koutinas AA. 2004. Immobilization technologies and support materials suitable in alcohol beverages production: A review. Food Microbiol. 21: 377–397.
- Lebeau T, Jouenne T, Junter G. 1997. Fermentation of D-xylose by free and immobilized Saccharomyces cerevisiae. Biotechnol. Lett. 19: 615–618.
- Liouni M, Drichoutis P, Nerantzis ET. 2007. Studies of the mechanical properties and the fermentation behavior of double layer alginate-chitosan beads, using Saccharomyces cerevisiae entrapped cells. World J. Microbiol. Biotechnol. 24: 281–288.
- Liu C, Wang F, Ou-Yang F. 2009. Ethanol fermentation in a magnetically fliized bed reactor with immobilized Saccharomyces cerevisiae in magnetic particles. Bioresource Technol. 100: 878–882.
- Liu M, Xu ZK, Wang JQ, Wu J, Fu JJ. 2004. Surface modification of polypropylene microfiltration membranes by graft polymerization of N-vinyl-2-pyrrolidone. Eur. Polym. J. 40: 2077–2087.
- Liu R, Shen F. 2008. Impacts of main factors on bioethanol fermentation from stalk juice of sweet sorghum by immobilized Saccharomyces cerevisiae (CICC 1308). Bioresource Technol. 99: 847–854.
- Mallouchos A, Reppa P, Aggelis G, Kanellaki M, Koutinas AA, Komaitis M. 2002. Grape skins as a natural support for yeast immobilization. Biotechnol. Lett. 24: 1331–1335.
- Miller GL. 1959. Use of dinitrosalicylic acid reagent for determination of reducing sugar. Anal. Chem. 31: 426–428.
- Nabais RC, Correia I, Viegas CA, Novais JM. 1988. Influence of calcium ion on ethanol tolerance of saccharomyces bayanus and alcoholic fermentation by yeasts. Appl. Environ. Microb. 54: 2439–2446.
- Najafpour G, Younesi H, I smail K. 2004. Ethanol fermentation in an immobilized cell reactor using Saccharomyces cerevisiae. Bioresour. Technol. 92: 251–260.
- Naumova ES, Naumov GL, Masneuf-Pomarède I, Aigle M, Dubourdieu D. 2005. Molecular genetic study of introgression between Saccharomyces bayanus and S. Cerevisiae. Yeast 22: 1099–1115.
- Nikolic S, Mjovic L, Rakin M, Pejin D, Nedovic V. 2009. Effect of different fermentation parameters on bioethanol production from corn meal hydrolyzates by free and immobilized cell of S. cerevisiae var. ellipsoideus. J. Chem. Technol. Biotechnol. 84: 497–503.
- Phisalaphong M, Budiraharjo R, Bangrak P, Mongkolkajit J, Limtong S. 2007. Alginate-Loofa as carrier matrix for ethanol production. J. Biosci. Bioeng. 104: 214–217.
- Qiu J, Xu L, Peng J, Zhai M, Zhao L, Li J, Wei G. 2007. Effect of activated carbon on the properties of carboxymethylcellulose/activated carbon hybrid hydrogels synthesized by γ-radiation technique. Carbohyd. Polym. 70: 236–242.
- Rojey A, Monot F. 2010. Biofuels: Production and applications. In: W. Soetaert and E. Vandamme (eds.), Industrial Biotechnology, Wiley, New York, 413–432.
- Serra A, Strehaiano P, Taillandier P. 2005. Influence of temperature and pH on Saccharomyces bayanus var. uvarum growth: Impact of a wine yeast interspecific hybridization on these parameters. Int. J. Food Microbiol. 104: 257–265.
- Shindo S, Tanaka S, Taguchi H, Yoshimura N. 2001. Development of novel carrier using natural zeolite and continuous ethanol fermentation with immobilized Saccharomyces cerevisiae in a bioreactor. Biotechnol. Lett. 23: 2001–2004.
- Winkelhausen E, Velickova E, Amartey SA, Kuzmanova S. 2010. Ethanol production using immobilized Saccharomyces cerevisiae in lyophilized cellulose gel. Appl. Biochem. Biotechnol. 162: 2214–2220.
- Yiğitoğlu M, Işiklan N, Özmen R. 2007. Graft copolymerization of N-vinyl-2-pyrrolidone onto sodium carboxymethylcelulose with azobisisobutyronitrile as the initiator. J. Appl. Polym. Sci. 104: 936–943.
- Yu J, Zhang X, Tan T. 2007. A novel immobilization method of Saccharomyces cerevisiae to sorghum bagasse for ethanol production. J. Biotechnol. 129: 415–420.
- Zhang Y, Ma Y, Yang F, Zhang C. 2009. Continuous acetone–butanol–ethanol production by corn stalk immobilized cells. J. Ind. Microbiol. Biot. 36: 1117–1121.
- Zhao J, Xia L. 2010. Ethanol production from corn stover hemicellulosic using immobilized recombinant yeast cells. Biochem. Eng. J. 49: 28–32.
- Zohuriaan-Mehr MJ, Pourjavadi A, Salehi-Rad M. 2004. Modified CMC. 2. Novel carboxymethylcellulose- based poly (amidoxime) chelating resin with high metal sorption capacity. React. Funct. Polym. 61: 23–31.