Abstract
To study the adhesion, proliferation and expression of bone marrow mesenchymal stem cells (BMSCs) on nano-hydroxyapatite (Nano-HA) bone graft material after transfection of adenovirus-mediated human bone morphogenetic protein-2 expression vector (Ad-BMP-2). BMSCs were transfected using Ad-BMP-2. Immunohistochemistry and Western blot were used to detect BMP-2 expression in transfected cells. After transfection, BMP-2 protein was highly expressed in BMSCs; MTT test assay showed that the Nano-HA bone graft material could not inhibit in vitro proliferation of BMSCs. Ad-BMP-2-transfected BMSCs are well biocompatible with Nano-HA bone graft material, the transfected cells in material can secrete BMP-2 stably for a long time.
Introduction
For a long time, restoration of bone defect remains an important subject in biomaterial and orthopedics. However, even till now, restoration of large bone defect caused by trauma, infection and tumor excision is calling for effective solution. With the rapid development of tissue engineering and genetic engineering technologies, bone tissue engineering combining seed cell and biomaterial and cell genetic modification technologies with DNA Recombination at its core are embracing a bright future. Seed cells in bone tissue engineering may come from periosteum, cancellous bone, bone marrow and other tissues outside bone, among which bone marrow mesenchymal stem cells (BMSCs) are the top seed cells in bone tissue engineering due to its wide sources, little trauma in the process of isolation and rapid proliferation (Jiang et al. Citation2002, Grove et al. Citation2004). Bone morphogenetic protein-2 (BMP-2) accelerates osteocyte proliferation, matrix secretion and vascularization and induces in vitro and in vivo bone formulation (Rutherford et al. Citation2002, Santoni et al. Citation2007). However, as it is extremely difficult to isolate natural BMP-2 and rhBMP-2's failure to endure 37 °C in vivo environment, short half-life period as well as difficulty to maintain effective concentration, its application has been subject to strict limitation. BMP-2 transgenic-induced osteoblast could achieve continuous BMP-2 expression over a certain period of time, thus overcoming deficiencies of its direct application. At present, Viral and nonviral BMP-2 expression vectors have been used in studies of BMP-2 transgenic-induced osteoblast. In the studies, adenovirus-mediated BMP-2 transgenetic approach is found most effective of all (Alden et al. Citation2002). This experiment will explore feasibility of nano-hydroxyapatite (Nano-HA) bone graft material as transfected BMSCs vector by transfecting adenovirus-mediated human bone morphogenetic protein-2 expression vector (Ad-BMP-2) of BMSCs on Nano-HA bone graft material.
Material and method
Design
Cell-Material Science, in vitro observation experiment.
Time and place
The experiment is conducted at the central lab of The Second People's Hospital of Shenzhen in Oct, 2009.
Materials
Animals used in the experiment
Six rabbits from New Zealand aging between 3 and 5 months with the weight of 2.0–2.5 kg and without restriction in sexuality. They are brought from Laboratory Animal Center of Southern Medical University. The animals are treated according to Guidance to Treat Experimental Animals released by Ministry of Science and Technology in 2006.
Nano-HA bone graft material
A 7 × 7 × 15 mm cuboid-shaped bar jointly developed by the tissue engineering lab of the Second People's Hospital of Shenzhen and Powder Metallurgy Research Institute of Central South University, the porosity of which is around 90% with the pore diameter ranging from 100 to 250 μm. See and .
Method
Separate culture of rabbit BMSCs
After intravenous anesthesia with Ketamine, extract 4 mL of marrow fluids by puncture at the tibial tubercle of rabbit. Separate and culture BMSCs through density gradient centrifugation combined with adherent method (Lennon et al. Citation1996), when the 10-d cells are cultured up to 80% confluence, siphoning off the original medium with digestion with 0.25% trypsin and subculture the cells under the proportion of 1:2. Use the third generation of cells for the experiment below.
Ad-BMP-2/GFP transfected rabbit BMSCs
For the third generation cells with around 80% of fusion rate, replace the serum-free medium, and extract the medium after 4 hours of incubation. Infect BMSCs at an MOI (multiplicity of infection) of 50, 100, and 200 in accordance with the viral titer. After transfection, place BMSCs in the incubator without culture media for 1 hour and oscillate the culture flask slightly every 15 minutes. One hour later, replace the medium with normal serum (0.1 in volume fraction) and continue culturing. Transfect the proliferated cells with Ad-GFP under the same condition, and observe the expressions of BMSCs of GFP transfected with Ad-GFP 48 hours later under fluorescence microscope (Leica from German), thus indirectly indicating expression of mature peptide of BMP-2 and transfection efficiency so as to determine the appropriate infection MOI.
Examination of BMP-2 expressions of transfected cells through immunohistochemistry
Fix the cell-climbed film of transfected cells after digestion and examine the cells according to S-P Immunohistochemistry kit in DAB color.
Examination of BMP-2 expressions of transfected cells through Western-blot
Extract protein from cells with TRIzol 24, 48 and 72 hours after being transfected; 10% SDS-PAGE gel electrophoresis (Biorad vertical electrophoresis apparatus), 15 μL of sample, 120 V, and electrophoresis for 60 minutes; transfer 30 V voltage for 30 minutes (Biorad SD semi-dry transfer membrane instrument); close it overnight in 1% BSA under 4°C; use antibody and secondary antibody(in 1%BSA at a 1:1000 dilution) to culture the cells respectively for 1 hour, and wash it with 0.1% Tween-PBS for three times for 3 minutes each time; culture the cells in ECL light-emitting liquid (1 ml of A liquid: 20 μL of B liquid) under room temperature for 1 minute, and put the cells in a cassette and expose the film in the darkroom for 3 minutes. Obtain the same amount of untransfected cell proteins as controls.
BMSCs combined Nano-HA bone graft material
Make the Nano-HA into 7 × 7 × 7 mm cube, soak it in fetal bovine serum (0.1 in volume fraction) DMEM medium overnight after sterilization under high temperature and high voltage. Suck the cubic with sterile filter paper before using it. Gather the untransfected BMSCs and transfected BMSCs above through trypsin digestion, make them into single cell suspension and inoculate them respectively to Nano-HA material bars. Adhere the bars in incubator with 5% of CO2 under 37°C for 2 hours, and then put fetal bovine serum (0.1 in volume fraction) DMEM medium slowly into the incubator to continue culturing the bars and change the medium every 2d ().
Table I. MTT analysis of bone marrow mesenchymal stem cell proliferation (_x ± s, n = 12, A value).
Examine cell carrier complexes under scanning electron microscope
Take out the two groups of materials respectively on Day 3 and Day 5 when culturing the complexes of material and cell. Rinse the two groups in PBS and fix them with 2% glutaraldehyde, wash them with PBS liquid, dehydrate with acetone and ethanol, exchange with isoamyl acetate, dry them at the critical point and observe them after spraying metal. Observe the attachment, distribution and proliferation of cells on the surface of material through scanning electron microscope (Hitachi of Japan).
Examine expression of BMP-2 protein of compounded cells through Western Blot
Take the cell vector complex on Day 3, 5 and 7 after being compounded, wash it with PBS for three times, go through digestion in 0.25% trypsin for 5 minutes, add in DMEM to terminate digestion, transfer the cell suspension to centrifuge tube at 2500 r/min and centrifuge for 10 minutes. Dispose of the supernatant and add in TRIzol to extract protein of cells being digested and compounded. Use electrophoretic separation of the proteins with methods mentioned above, transfer the membrane and close it, and culture it with antibody and secondary antibody in ELC color.
Designer, implementor and evaluator
The experiment is designed by the corresponding author, implemented by the third author and evaluated by the first author.
Statistics analysis
Use SPSS 10.0 statistics software to analyze the experimental data. The data is represented by ̅x ± s and goes through t-test. p < 0.05 indicates significant difference.
Results
Separative culture and identification of BMSCs
Long spindle-shaped fibroblast-like phenotype, the BMSCs are arranged in a spiral shape (). It could differentiate into osteoblasts and form mineralized nodules through induction identification ().
Test of Ad-GFP transfection efficiency
Forty-eight hours after transfection of Ad-GFP, it is found to be in good state when transfected at MOI of 100 under the fluorescence microscope, with nearly all green fluorescence being visible and around 100% of transfection efficiency (); The cell is in the best status when transfected at MOI of 50; however, at the point it features few proteins of green fluorescence and poor transfection efficiency; there are a lot of proteins of green fluorescence at MOI of 200, yet under the microscope, morphology retraction is found in some of the adherent cells as well as a few floating cells, which might relate to cell infection by adenovirus; the results show that MOI of 100 is the most appropriate. There remains a few green fluorescence spotted under the fluorescence microscope 6 weeks later.
BMP-2 expression test through immunohistochemistry
The results of cell BMP-2 expression test through immunohistochemistry indicate that brown granules in the plasma of transfected BMSCs have positive signals (as shown in ) while the untransfected cells appear negative as shown in .
Figure 6. Transfection 72 hour, bone morphogenetic protein-2 immunohistochemical staining showed positive expression in cytoplasm.
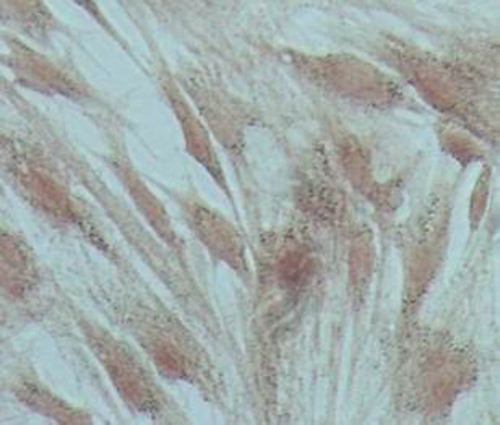
Figure 7. Untransfected cells were negative for bone morphogenetic protein-2 immunohistochemical staining.
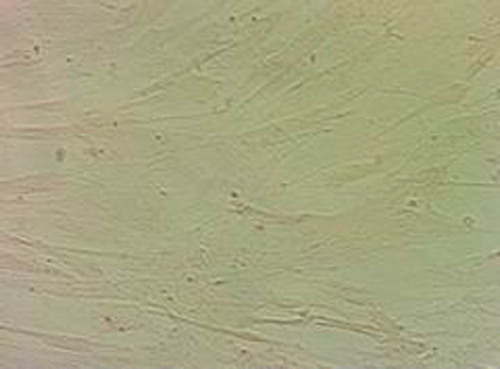
BMP-2 protein expression test through Western blot
The extracted proteins of BMSCs on Day 1, 2 and 3 after being transfected are found to have around Mr 30,000 sized positive bands under Western blot, indicating that the transfected group, after Ad-BMP-2 transfection, have BMP positive bands, which will become strong positive with the progress of time while the untransfected group is spotted with relatively weak band (), showing that BMSCs will have high expression at protein-level after being transfected by virus.
Observation of BMSCs combined
Nano-HA bone graft material through scanning electron microscope. On Day 3 of complex culture, the SEM found irregular surfaces on the vector, on which micropores are visible with part of them being covered. The transfected cells attaches well to the lacunae and gaps of Nano-HA materials, with the concentrated parts being connected to slices, featuring good attachment and extension capability as shown in . On Day 5 of complex culture, the cells were fully extended on the surface of material and stretched out pseudopodia. We could find in that the long pseudopodia is well attached to the surface. Calcium salt secretion and deposition were also found around the edge of cells, indicating high cell affinity of the material, which is condusive to the growth of cell.
BMP-2 protein expression test of complex cells through Western blot
The extracted proteins on Day 1, 2 and 3 after being compounded were found with high expression of BMP-2 protein in the transfection group through Western blot (), indicating the transfected cell vector complex is able to continue secretion of BMP-2.
Discussion
For a long time, restoration of bone defect, and large bone defect in particular, remains a difficult issue in orthopedics. The traditional autogenous bone graft, due to limited material and complications, is restricted in application while allogeneic bone or artificial bone achieves poor treatment effects due to the absence of life cells. The gene-enhanced tissue engineering offers an excellent approach for resolving this issue by combining tissue engineering and genetic engineering (Edwards et al. Citation2005). The tissue engineering is a cross-disciplinary boundary science which emerged in 1980s. It serves as the theoretical and technological foundation of commercial engineering and life sciences to develop biological substitutes and restore or rebuild functions of various tissue and organs. The tissue engineering includes three main elements, namely growth factors, seed cell, biomaterial of tissue engineered structure (Shen et al. Citation2006).
An ideal seed cell in the tissue engineering should have the following characteristics: easy access to material and few damages to the organism; strong in vitro extension capacity; prone to differentiate into osteoblasts; rapid adaptation to the physiological, pathological and stress environment and good maintenance of osteogenic activity after being implanted into the body. BMSCs, a kind of stem cells with multiple potentials, have a wide and convenient sources which also feature easy culture and rapid proliferation (Grove et al. Citation2004). It can differentiate into osteoblast, chondroblast, adipocyte and hematopoietic support matrix under certain conditions (Bianco et al. Citation2001), making it the most probable ideal seed cell for artificial bone grafting in tissue engineering. BMSC has a particular pattern of cell proliferation and division, enabling easy introduction and expression of foreign gene, making it a potential target cell for genetic treatment (Peng et al. Citation2009a). Therefore, this experiment conducted in vitro expansion of BMSCs and modify the gene by making the biomaterial vector into a complex of cell and material, and then implant the complex back into the body for the experiment of bone defection restoration.
Another important element in tissue engineering is the vector of seed cell. The experiment chooses Nano-HA bone graft material as the vector. HA is the most commonly used bioactive material. Its chemical composition and crystal structure are similar to apatite in human bones, featuring good bone conduction performance and bioactivity, conduciveness to bone development, stable phase state and nontoxicity. However, though the micron-level HA enjoys stable phase state, it is poor in bioabsorbability and mechanical property with high fragility and low antipressure and antibending strength, excluding it from the category of ideal bone tissue materials. With the application of nanoscale science and technology in medical science, R&D and utilization of Nano-HA material have received even more attention from domestic and overseas scholars. The nanoscale-level grain size and grain boundary width of Nano-HA help reduce inherent deficiencies of the material while bringing it surface effect and volume effect, giving it great advantages and potential in utilization in both mechanics and biology and making it an ideal material for tissue implant (Tan et al. Citation2001). Its resemblance to biological apatite in structure proves highly useful in integration of bone tissues, which is suitable for cell proliferation and has higher bone conduction performance and solubility than micron-level HA. The new Nano-HA bone graft material, which is jointly developed by the Institute and Powder Metallurgy Research Institute of Central South University, with various pore diameters through different model articrafts, proves in the animal experiment that Nano-HA material with porosity ranging from 100 to 250 μm has significantly higher capacity of osteoblast formulation than materials with pore diameter of 50–150 μm and 300–500 μm (CitationWang et al. 2007, Zhu et al. Citation2009). This experiment compounded transfected BMSCs with Nano-HA scaffold to construct BMP-2 genetic enhanced tissue engineered bone. Under SEM, we observed that BMSCs attached to and grown in/on the scaffold surface or pore of Nano-HA while secreting extracellular matrix. Meanwhile there was a large number of microvilli on the surface of the cell, indicating good growth situation of cell on the scaffold. The Western blot test found that the cell vector complex could continuously secrete BMP-2, laying experimental foundation for the utilization of BMP-2 genetic enhanced tissue engineered bone in restoration of bone defection.
Among numerous bone growth factors, BMP-2 is considered as the only factor that can induce bone growth alone with the highest activity (Wozney and Rosen Citation1998). The factor shows significant effect in restoration of bone defects and fracture healing. In the process of bone defect restoration, BMP-2 could improve cell proliferation, division and the matrix secretion while being able to induce in vitro and in vivo BMSCs to differentiate to osteoblast (Tsuchida et al. Citation2003, Lee et al. Citation2002). However, osteoblast induction by BMP-2 is a process in which events including chemotaxis, collecting and acceleration of precursor cell differentiation take place by order during different periods of time. The concentration of BMP-2 at different phases could be quite different, ranging from 10–15 mol/L to 10–3 mol/L (Reddi Citation1994). Direct utilization of BMP-2 in one-time medicine reveals rapid dilution and absorption by tissues of BMP-2 (Lee Citation2000), which is inconsistent with the natural discipline of BMP-2 inducted bone growth. To transfer coded BMP-2 gene segments to BMSCs by gene transfer techniques offers innovative idea and approach to resolve the issue above. Adenovirus expression vector also serves as a main vector for gene therapies due to high transfection efficiency, high gene expression, few immunological rejection and high security coefficient. We construct adenovirus vector by GatewayTM technology (Peng et al. Citation2009b) and use Ad-BMP-2/GFP to transfect BMSCs under the strategy of in vitro gene therapy. The experiment found that the BMP-2 gene could efficiently introduce cells and express BMP-2 gene products. Expression of GFP, a exogenous gene product was observed at just 2d of infected BMSCs, and diminished gradually 6 weeks later. Though the adenovirus vector is short in expression of target gene (the longest is subject to 6 weeks), the restoration of bone is a periodic process which has no need in long expression of target gene. Therefore, it is an ideal vector to introduce target gene into BMSCs for the purpose of bone recovery (Service Citation2000, Baltzer 2000) with no concern about continuous over expression which might cause excessive hyperosteogeny, affect bone reformulation or even lead to tumorigenicity (Yang 2007). The experiment shows that adenovirus mediated exogenous target gene expression is a highly efficient method of gene transfer, the transfection efficiency and protein expression of which fully suit the need of bone tissue engineering.
In conclusion, BMSCs transfected by Ad-BMP-2 is well biocompatible with Nano-HA bone graft material, where cells are able to stably and efficiently secrete BMP-2 and achieve satisfied effects in in vitro construction of new tissue engineered bone, offering new type of bone alternative for experiment on further restoration of bone defection.
Declaration of interest
The authors report no conflicts of interest. The authors alone are responsible for the content and writing of the paper.
References
- Alden TD, Varady P, Kallmes DF, Jane JA Jr., Helm GA. 2002.Bone morphogenetic protein gene therapy.Spine (Phila Pa 1976).27(16 Suppl 1):S87–S93.
- Baltzer AW, Lattermann C, Whalen JD, Wooley P, Weiss K, Grimm M, . 2000.Genetic enhancement of fracture repair: healing of an experimental segmental defect by adenoviral transfer of the BMP-2 gene. Gene Ther. 7:734–739.
- Bianco P, Riminucci M, Gronthos S, Robey PG. 2001.Bone marrow stromal stem cells: nature, biology, and potential applications. Stem Cells. 19:180–192.
- Edwards PC, Ruggiero S, Fantasia J, Burakoff R, Moorji SM, Paric E, . 2005.Sonic hedgehog gene-enhanced tissue engineering for bone regeneration. Gene Ther. 12:75–86.
- Grove JE, Bruscia E, Krause DS. 2004.Plasticity of bone marrow- derived stem cells. Stem Cells. 22:487–500.
- Jiang Y, Jahagirdar BN, Reinhardt RL, Schwartz RE, Keene CD, Ortiz-Gonzalez XR, . 2002.Pluripotency of mesenchymal stem cells derived from adult marrow. Nature. 418:41–49.
- Lee FY, Storer S, Hazan EJ, Gebhardt MC, Mankin HJ. 2002.Repair of bone allograft fracture using bone morphogenetic protein-2. Clin Orthop Relat Res.119–126.
- Lee SJ. 2000.Cytokine delivery and tissue engineering. Yonsei Med J. 41:704–719.
- Lennon DP, Haynesworth SE, Bruder SP, Jaiswal N, Caplan AI. 1996.Development of a serum screen for mesenchymal progenitor cells from bone marrow. In Vitro Cell Dev Biol Anim. 32:602–611.
- Peng LQ, Wang DP, He CL, Zhe WM. 2009a. Zhongguo Linchuang Jiepaoxue Zazhi. 27:321–324.
- Peng LQ, Wang DP, Zhou K, Huang JF. 2009b. Zhongguo Zuzhi Gongcheng Yanjiu yu Linchuang Kangfu. 13:3942–3946.
- Reddi AH. 1994. Bone and cartilage differentiation. Curr Opin Genet Dev. 4:737–744.
- Rutherford RB, Moalli M, Franceschi RT, Wang D, Gu K, Krebsbach PH. 2002. Bone morphogenetic protein-transduced human fibroblasts convert to osteoblasts and form bone in vivo. Tissue Eng. 8:441–452.
- Santoni BG, Pluhar GE, Motta T, Wheeler DL. 2007. Hollow calcium phosphate microcarriers for bone regeneration: in vitro osteoproduction and ex vivo mechanical assessment. Biomed Mater Eng. 17:277–289.
- Service RF. 2000. Tissue engineers build new bone. Science. 289: 1498–1500.
- Shen JY, Chan-Park MB, He B, Zhu AP, Zhu X, Beuerman RW, . 2006. Three-dimensional microchannels in biodegradable polymeric films for control orientation and phenotype of vascular smooth muscle cells. Tissue Eng. 12:2229–2240.
- Tan W, Krishnaraj R, Desai TA. 2001. Evaluation of nanostructured composite collagen–chitosan matrices for tissue engineering. Tissue Eng. 7:203–210.
- The Ministry of Science and Technology of the People's Republic of China. Guidance Suggestions for the care and use of laboratory animals. 2006–09–30.
- Tsuchida H, Hashimoto J, Crawford E, Manske P, Lou J. 2003. Engineered allogeneic mesenchymal stem cells repair femoral segmental defect in rats. J Orthop Res. 21:44–53.
- Wang DP, Han Y, Zhu W, Lu W, Liu H, Jiang H, Xiong J, Liu J, Ou Y, Liu W. Effects of Bone Defect Repair by Nano-hydroxyapatite Artificial Bone with Different Pore Diameters [J]. J Clin Rehabil Tissue Eng Res. 11:9641–9645.
- Wozney JM, Rosen V. 1998. Bone morphogenetic protein and bone morphogenetic protein gene family in bone formation and repair. Clin Orthop Relat Res. 26–37.
- Yang ZR, Wang HF, Zhao J, Peng YY, Wang J, Guinn BA, Huang LQ. 2007. Recent developments in the use of adenoviruses and immunotoxins in cancer gene therapy. Cancer Gene Ther. 14:599–615.
- Zhu W, Xiao J, Wang D, Liu J, Xiong J, Liu L, . 2009. Experimental study of nano-HA artificial bone with different pore sizes for repairing the radial defect. Int Orthop. 33:567–571.