Abstract
Encapsulation of a multimeric alcohol oxidase (AOx) in giant vesicles (GVs) was prepared by rapid evaporation method. The specific activity (477 U/mg-protein) of the GV encapsulated AOx was 3.5 fold higher than the free AOx. The half-life (˜59.4 hours) of the encapsulated AOx was 20-fold higher than the free enzyme at 30°C. Further, the GV was used to encapsulate AOx and HRP together as coupled reaction catalyst for chromogen-based optical detection of alcohols. The results confirmed potential application of the GV as micro-dimensional container for enhancing stability, activity and biosensing applications of AOx.
Introduction
The ability of liposomes to mimic the behavior of natural cell membranes has been exploited to develop liposome–based constructs for various applications (Walde and Ichikawa Citation2001). The liposomal encapsulated enzyme system protects the enzyme from external agents, such as proteases and also prevents the enzyme from denaturation (Nasseau et al. Citation2001). The enzyme-encapsulated liposomes can be used as a functional biocatalytic container in organic synthesis. Various liposome-encapsulated enzymes have been prepared and characterized for drug delivery, biocatalytic, and synthetic biology applications (Walde and Ichikawa Citation2001, Walde et al. Citation2009, Stano et al. Citation2011). Different methods, including rapid evaporation method, for the preparation of enzyme containing lipid vesicles have been reviewed by Walde and Ichikawa (Walde and Ichikawa Citation2001). We report here the multilameller giant vesicles (GVs) prepared by rapid evaporation method for encapsulating a broad substrate specific alcohol oxidase (AOx) isolated from Aspergillus terreus 6324 (Kumar and Goswami Citation2006). In general, the AOx is a complex enzyme protein consisting of many subunits as reported mostly from the fungal sources (Ozimek et al. Citation2005, van Dijk et al. Citation2002). The enzyme is highly sensitive to ionic strength and temperature (Kumar and Goswami Citation2008) and hence stabilization of AOx is a prerequisite for its application in biocatalytic process. We utilized the giant liposome system not only to stabilize the enzyme but also to use it as container to carry out a coupled reaction for detection of alcohol in the sample.
Experimental
Chemicals
L-α-Phosphatidylcholine (≥ 99% TLC), Stearylamine (≥ 99.0% GC), Cholesterol (≥ 99%), Green fluorescence protein (GFP), Peroxidase (˜200 units/mg), ABTS [2,2’- azino-di-(3-ethylbenzthiazoline-6-sulphonic acid)], were obtained from Sigma-Aldrich, India. DMSO (dimethylsulfoxide), BSA, and alcohol substrates were obtained from Merck, India.
Isolation of AOx
The AOx from Aspergillus terreus MTCC 6324 was isolated following the reported procedure (Kumar and Goswami Citation2008). In brief, the harvested cells in 50 mM Tris-HCl buffer, pH 8.0 (THB) was disrupted at 4°C. The AOx present in 20,000× g supernatant of the disrupted cells homogenate was precipitated by ammonium sulfate (35% w/v saturation) and desalted against THB. The membrane bound AOx was solubilized by CHAPS (0.5% w/v). The supernatant obtained at 114000× g was lyophilized to obtain powdered AOx containing 130 U/mg-protein.
Preparation of composite enzyme/GFP containing liposomes
Giant vesicles (GVs) were prepared by rapid evaporation method (Moscho et al. Citation1996). A lipid composition of Stearylamine/L-α-Phosphatidyl Choline/Cholesterol (1:4:5 mole ratio) was dissolved in 1 ml of chloroform in a 50 ml round bottom flask. The aqueous phase containing suitable concentration of AOx, or AOx, ABTS and HRP (composite enzyme) for coupled reaction or GFP in the respective buffer was then added along the flask walls. For GFP encapsulation THB were adjusted to 7.0. The organic solvent from the mixture was removed by vacuum evaporator at 30°C. An opalescent fluid containing GV obtained after the evaporation was subjected to 15,000× g for 45 min at 4°C to separate un-capsulated enzyme/proteins from the liposomes. The supernatant was removed and 5 mL THB was added to the pellet to re-suspend the liposomes. This washing process was repeated to ensure the prepared liposomes free from un-encapsulated proteins, which was further verified by analyzing protein concentration/enzyme activity in each extracting supernatant concomitantly. The encapsulated enzyme/protein liposome preparation was finally suspended in a 3 mL THB for storing at 4°C. The entrapment percent (EP) was calculated as follows:
EP = (TE-FE)/TE × 100%.
Where, TE = Total enzyme, FE = Free enzyme
The AOx activity was measured using the spectrophotometric assay method (Kemp et al. Citation1988) using ABTS and n-heptanol as substrate. The HRP-coupled assay was used to monitor the production of H2O2 at 405 nm for ABTS radical at 30°C (ϵ405 = 18,400 μM−1cm−1). The stoichiometric equivalence of 1 mM ABTS with 0.5 mM oxidized substrate was considered to measure the product. One unit (IU) of enzyme was defined as the amount of enzyme activity producing 1 μmol of H202 per min under the assay conditions. The activity of the AOx in GV was measured by mixing an aliquot of 0.5 mL (2500 GVs/ml) of AOx-GV with 5 mL of THB containing HRP (1 mg/ml or 7 U/ml), ABTS (0.7 mg/ml) and n-Heptanol (10 mM). Liposome encapsulated with BSA was used as control for the experiments. Protein estimation was done following Bradford method.
Results and discussions
Initially, GFP was encapsulated in the GVs and the image of the GFP-GVs was captured by a camera (Nikon E8400) attached to a microscope (Nikon E-200) under bright field mode () and also fluorescence emission mode using a laser light of 488 nm (). The entrapment of GFP was clearly visible from the specific fluorescence emitted by the GVs. The majority of the vesicles were spherical with sizes distributed largely within 1–100 μm (dia). The GVs count [suspension chamber 1 cm length × 1 cm breath × 3 mm high) was 5 GVs/μl at an initial lipid concentration of 1.071 mg/ml-CHCl3. The number and sizes of the GFP encapsulated GVs were constant for at least three weeks when stored at 4°C. No aggregation of the GVs was also observed during the period.
Figure 1. The image of GFP-GVs captured through a 40 × objective in a (A) bright field mode and B: Fluorescence mode. C: Enzs-GV upon addition of n-heptanol. D: Image of multilamellar vesicle containing Enzs-GV. In 1C, 1D Scale Bar: 40 μm. Each camera pixel represents 0.2 μm in the images 1A, 1B.
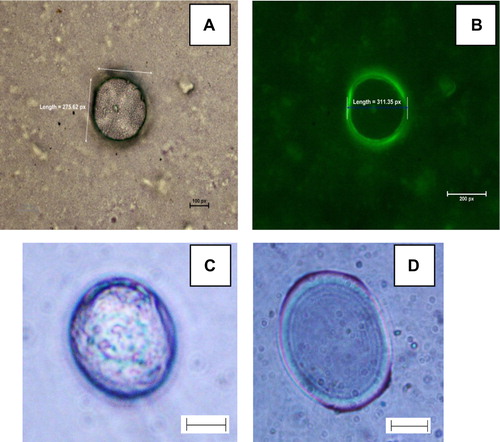
In our attempt to encapsulate AOx in GVs, an EP of 99.8 was achieved at the AOx protein of 0.84 mg/ml. The specific activity of 477 U/mg-protein of AOx in the AOx-GV with substrate n-heptanol was 3.5 fold higher than the specific activity of the free AOx (130 U/mg-protein). The reason for the increased specific activity is attributed to the supporting role of the lipoidic vesicle bilayer that provides the natural environment to the enzyme for enhanced catalysis. Notably, AOx is a membrane–bound enzyme that loses substantial activity upon its dissociation from the microsomal membrane. The efficiency of liposomal encapsulation of proteins is generally low ranging from ca. 5% to 20% for the enzymes of different sizes (Hwang et al. Citation2012). The exceptionally high level of entrapment of AOx in the GVs achieved through this investigation is thus a significant breakthrough in encapsulation of protein in liposomes. The high level of entrapment in the present case is attributed to the lipoidal nature of the AOx (Kumar and Goswami Citation2008) that facilitates avid binding of the protein with the liposome matrix. Polymeric membrane vesicles known as artificial cell, for the bio-encapsulating enzymes have also been reported (Chang Citation2007). However, studies on interaction between polymeric membranes and the enzyme Alcohol oxidase need to be explored to understand the stability of this enzyme within the polymeric vesicles. Our attempt is to stabilize a massive multimeric enzyme in a membrane that mimics native microsomal membrane, hence an environment that would be the closest mimic to the biological cell membrane i.e. a simple bilayer lipid membrane was used. The half life (t1/2) of GV encapsulated AOx (calculated from 0.693/k where ‘k’ is the first order rate constant) was ˜59.4 hours, whereas for the free enzyme it was ˜ 3 hours at 30°C and pH 8.0. While monitoring the half-life of the GV encapsulated AOx the GVs count (through microscopic observation) of the preparation was compared with the original value and confirmed that there was no significant degradation of the GVs, which further indicates the high stability of the GVs used for the analysis.Encapsulation of AOx within the vesicles led to localization of the enzyme in hydrophobic bilayers that may protect and stabilize the enzyme from rapid denaturation.
The Enzs-GVs were examined for their AOx catalytic activity using n-heptanol as the sole substrate. On addition of substrate n-heptanol the internal phase of the GVs turned green with an intense bluish-green layer at the boundary of the vesicle as shown in . The formation of the green color is attributed to the production of ABTS.+ radical as a result of the AOx catalytic oxidation of the substrate alcohol sequestered through the GV membrane. No such boundary could be observed in control GV samples. Formation of multilamellar Enzs-GVs was also observed as shown in . From the results obtained it can be inferred that AOx gets entrapped in the hydrophobic bilayer of the vesicle and the bilayer is permeable to the long chain alcohol substrate. The ABTS cation radical generally shows an absorption spectrum with five maxima, at 214, 394, 414, 646 and 728 nm (Solís-Oba et al. Citation2005, Johannes and Majcherczyk Citation2000). With increasing Enzs-GV concentration in the reaction mixture containing n-heptanol, the absorbance of the ABTS-specific peak at λ340 nm was decreased with a concomitant increase in the ABTS radical-specific absorption at λ 394, 414, 646, 728 nm (). An almost linear dependence of concentration of Enzs-GV to [ABTS.·] could be observed from the inset of . Using ϵ414 = 36000 M−1.cm−1 for ABTS.·, the sensitivity of the response was found to be 6.82 nM ABTS.· per Enzs-GV count. The findings demonstrated the occurrence of AOx-HRP coupled redox reaction inside the GVs and hence provide evidence on the retention of HRP and ABTS in the liposome matrix. ABTS cation radical is impermeable to bilayers and so is ABTS (Frimer Citation1985).
Figure 2. Spectrophotometric monitoring of the Enzs-GV catalyzed oxidation of n-heptanol. The Enzs-GV concentrations used were A = 100 μl, B = 200 μl, C = 400 μl, D = 600 μl, where the count of GV was 5/μl in THB. The concentrations of n-heptanol for the entire sample were fixed (100 μl) and the stock was prepared in DMSO.
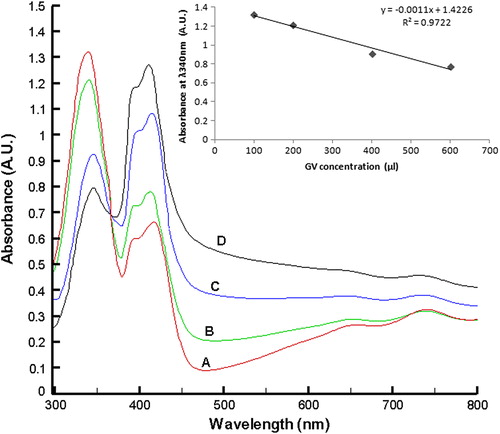
AOx is reported to oxidize a broad range of alcohol substrates with varying Km values (Kumar and Goswami Citation2008). We tested various substrate namely, methanol, butan-1-ol, n-heptanol, R-(2)-octanol, 2- dodecanol, and 1-undecanol for the catalytic activity of the Enzs-GV. Spectrophotometric scans have shown the production of ABTS.·, thus imply the activity realized with all the substrates used for the assay. However, exceptionally high activity of Enzs-GV was detected when n-heptanol (curve C in ) was used as substrate. Very low but significant activity of the Enzs- GV was detected for small chain (A, B) and long chain alcohols (D,E,F) as shown in the exploded spectra in the inset of the . The activities of Enzs-GVs obtained for the rest of the alcohol substrates were less by atleast 14-fold, than the ones obtained for n-heptanol. The low activity of AOx from A. terreus for the short chain alcohol substrates is known (Kumar and Goswami Citation2006); however, the reason for low activity of Enzs-GV for other long chain alcohols namely, R-(2)-octanol, 2-dodecanol, and 1-undecanol is not known.
Conclusion
The GV as an encapsulating matrix for the massive multimeric AOx protein has been demonstrated. Enhanced stability of the AOx upon its encapsulation in the GVs was also demonstrated. Further, the constructed GVs were used as a container for AOx-HRP-coupled reaction to probe the presence of alcohol in the samples using optical detection method. The findings have opened up a new avenue to explore GVs as a micro-dimensional container for high molecular weight enzyme and coupled enzymes for stability and biocatalytic reactions.
Acknowledgements
DBT, India provided JRF to V. Barua. The help rendered by Seraj Ahmad, Mitun Chakraborty, Soma Sekhar Reddy, Ankana Kakoti for purification of AOx is acknowledged.
Declaration of interest
The authors report no conflicts of interest. The authors alone are responsible for the content and writing of the paper.
References
- Chang TMS. 2007. Artificial cells: biotechnology, nanomedicine, regenerative medicine, blood substitutes, bioencapsulation, cell/stem cell therapy. In: Lim SC, Ed. Regenerative medicine, artificial cells and nanomedicine, vol. 1. Hackensack, N.J.: World Scientific Publishers, pp. 299–332.
- Frimer AA. 1985. The Semipermeability of biological membranes: an undergraduate laboratory experiment. J Chem Educ. 62:89–90.
- Hwang SY, Kim HK, Choo J, Seong GH, Hien TB, Lee EK. 2012. Effects of operating parameters on the efficiency of liposomal encapsulation of enzymes. Colloids Surf B Biointerfaces. 94:296–303.
- Johannes C, Majcherczyk A. 2000. Natural mediators in the oxidation of polycyclic aromatic hydrocarbons by laccase mediator systems. Appl Environ Microbiol. 66:524–528.
- Kemp GD, Dickinson FM, Ratledge C. 1988. Inducible long chain alcohol oxidase from alkane-grown Candida tropicalis. Appl Microbiol Biotechnol. 29:370–374.
- Kumar AK, Goswami P. 2006. Functional characterization of alcohol oxidases from Aspergillus terreus MTCC 6324. Appl Microbiol Biotechnol. 72:906–911.
- Kumar AK, Goswami P. 2008. Purification and properties of a novel broad substrate specific alcohol oxidase from Aspergillus terreus MTCC 6234. BBA Protein Proteome. 1784:1552–1559.
- Moscho A, Orwar O, Chiu DT, Modi BP, Zare RN. 1996. Rapid preparation of giant unilamellar vesicles. Proc Natl Acad Sci USA. 93: 11443–11447.
- Nasseau M, Boublik Y, Meier W, Winterhalter M, Fournier D. 2001. Substrate-permeable encapsulation of enzymes maintains effective activity, stabilizes against denaturation, and protects against proteolytic degradation. Biotech Bioeng. 75:615–618.
- Ozimek P, Veenhuis M, van der Klei IJ. 2005. Alcohol oxidase: a complex peroxisomal, oligomeric flavoprotein. FEMS Yeast Res. 5:975–983.
- Solís-Oba M, Ugalde-Saldívar VM, González I, Viniegra-González GJ. 2005. An electrochemical–spectrophotometrical study of the oxidized forms of the mediator 2,20-azino-bis-(3-ethylbenzothiazoline-6-sulfonic acid) produced by immobilized laccase. J Electroanal Chem. 579:59–66.
- Stano P, Carrara P, Kuruma Y, Pereira De Souza T, Luisi PL. 2011. Compartmentalized reactions as a case of soft-matter biotechnology: synthesis of proteins and nucleic acids inside lipid vesicles. J Mat Chem. 21:18887–18902.
- van Dijk R, Lahtchev KL, Kram AM, van der Klei IJ, Veenhuis M. 2002. Isolation of mutants defective in the assembly of octameric alcohol oxidase of Hansenula polymorpha. FEMS Yeast Res. 1:257–263.
- Walde P, Ichikawa S, Yoshimoto M. 2009. The fabrication and applications of enzyme-containing vesicles. In: Ariga K, Nalwa HS, Eds. Bottom-Up Nanofabrication, vol. 2. Los Angeles: American Scientific Publishers, pp. 199–221.
- Walde P, Ichikawa S. 2001. Enzymes inside lipid vesicles: preparation, reactivity and applications. Biomol Eng. 18:143–177.