Abstract
In this study; glucose oxidase (GOD) and MnO2 were immobilized in alginate gel beads using the entrapment method and effects of oxygen vectors on immobilized GOD activity were investigated. The specific activity of free enzyme was 1.3 units/mg protein whereas that of immobilized enzyme was 8.4 × 10−1 units/mg protein. The Km values for free and immobilized enzyme were found to be 2.7 mM, 2.9 mM; Vmax values 3.64 × 10−1 μmol/min.mL, 2.61 × 10−1 μmol/min.mL, respectively. When 4% (v/v) silicone oil or soybean oil was used, the activity of immobilized GOD increased 1.7 times and 1.3 times, respectively, compared to media without these oils.
Introduction
Gluconic acid (GA) and its salts are important compounds that are used in food, pharmaceutical, textile, detergent, leather, photographic and other biological industries (Ramachandran et al. Citation2006). GA can be produced by the partial oxidation of glucose through chemical catalysis, microbial conversion and enzymatic catalysis. Microbial conversion is one of the dominant routes for GA production at present (Ramachandran et al. Citation2006, Anastassiadis et al. Citation2003, Tomotani and Vitolo Citation2005, Jianguo and Zhanfeng Citation2007, Anastassiadis and Reehm Citation2006). However, using immobilized GOD instead of microbial conversion, the overall bioconversion process could be simplified by eliminating the disadvantages of microbial conversion such as cell separation, formation of by-products and the use of toxic organic solvents. In the last decade an increasing interest on enzymatic oxidation using GOD has been observed (Libertino et al. Citation2008, Holland et al. Citation2011, Arimatsu et al. Citation2004, Tavcer et al. Citation2012).
Glucose oxidase (EC 1.1.3.4) catalyzes the oxidation of D-glucose into D-glucono-1,5-lactone which is spontaneously hydrolyzed to gluconic acid and hydrogen peroxide. The deactivation of GOD was due to the attack of hydrogen peroxide produced during the oxidation of glucose. Since fine manganese-IV-oxide (MnO2) particles catalytically decompose hydrogen peroxide to water and oxygen, these particles were immobilized together with glucose oxidase in calcium alginate gel beads (Arimatsu et al. Citation2004).
Since oxygen solubility in water is very low (approximately 8–10 mg/L at 25–30°C and 1 atm pressure), the oxygen transfer rates could be enhanced by using oxygen-vectors such as fluorocarbons, phthalates, hemoglobin, soybean oil and silicone oil (Leung et al. Citation1997, Rols and Goma, Citation1991). Since the gluconic acid formation is an oxidative process, its overall productivity strongly depends on the dissolved oxygen concentration in the reactor. It is necessary to increase oxygen level in the reaction system to prevent oxygen limitation (Jianguo and Zhanfeng Citation2007). Although oxygen solubility is important, no research has been conducted using oxygen vectors during enzymatic reaction. Our main objective in this study was to investigate the enhancement of immobilized GOD enzyme activity by using silicone and soybean oil.
Materials and methods
Reagents
ß-D glucose and silicone oil were purchased from Fluka. Calcium chloride and acetic acid were purchased from Merck. MnO2, low viscosity sodium salt of alginic acid, glutaraldehyde and Bradford kid for protein determination were obtained from Sigma. Soybean oil was bought from commercial market. GOD was isolated from Alphamalt Gloxy 14080 (Mühlenchemie, Germany). Alphamalt Gloxy 14080 is inexpensive and used in baking industry. It consists of enzyme and processed wheat flour as carrier. This commercial preparation was stored in a cool and dry place and used without any activity loss. The water used in analyses is double-distilled.
Enzyme isolation
2.5 g of Alphamalt Gloxy was dissolved in 12.5 mL of water. The solution was filtered (Whatman paper -41). The filtrates were combined and made up to 25 mL with water in volumetric flask. The prepared solution was used as GOD enzyme solution.
Enzyme immobilization
The enzyme was immobilized in calcium alginate beads. 0.75 g of sodium alginate was dissolved in 25 mL of enzyme solution and 0.5 g of MnO2 was added into this solution. This mixture was dropped into 100 mL of 1M CaCl2 solution by using a pipette (Blandino et al. Citation2001). Once the Na-Alginate/MnO2/enzyme solution had been dropped into CaCl2 solution, a capsular membrane formed instantaneously around each droplet due to the cross-linking of the interfacial alginate molecules by calcium cations. In this way, spherical, black gel beads were formed at 25°C. The solution was kept in refrigerator at 4°C for an hour. After this gelation time, the gel beads were filtered using a Buchner funnel. The beads were washed three times with water, and immediately transferred into 2.5% v/v glutaraldehyde solution and incubated for 5 minutes. The glutaraldehyde solution was used to avoid enzyme leakage. Finally, the gel beads were washed with water to remove excess glutaraldehyde. 15.2 g of Ca-alginate beads were obtained at the end of the immobilization procedure and the median diameter of gel beads was determined as 3.71 ± 0.05 mm. The Ca-alginate gel beads were stored in 50 mM Ca-acetate buffer (pH 5.5) at 4°C.
All filtrates and washing solutions were used for the determination of protein concentration.
Determination of protein concentration
The protein concentration of filtrates and washing solutions after immobilization was determined according to the Bradford method (Bradford Citation1976) using bovine serum albumin (BSA) as protein standard. The percentage of total immobilized enzyme was calculated as follows:
Loading efficiency (%) = [(CiVi − CfVf)/CiVi] × 100 (1)
Where Ci is the initial protein concentration, Vi the initial volume of enzyme solution, Cf the protein concentration in the total filtrate, and Vf the total volume of filtrate.
Determination of enzyme activity
4 mL of free and 3 g of immobilized enzyme were added into 50 mL of 30 mM glucose solution (in Ca- acetate buffer at pH 5.5). The experiments were carried out in a constant temperature shaker at 200 rpm and the airflow rate 4 vvm. At different time intervals, a sample was taken from the reaction medium. Trichloroacetic acid (15% w/v) was added to stop the reaction (Blandino et al, Citation2001). The sample solutions were centrifuged at 5000 rpm for 5 minutes and filtered through 0.40 μM microfiltration membrane. Free and immobilized enzyme activities (initial reaction rates) were determined by measuring the decreasing glucose concentration with a HPLC analysis. Glucose concentration was measured using HPLC (Waters) equipped with a sugarpak column and a refractive index detector. Flow rate and temperature were 0.5 mL per minute, 90°C, respectively. 50 mg/L Ca-EDTA was used as mobile solution. Standard glucose solutions were used for plotting a calibration curve.
Determination of kinetic parameters
The kinetic constants for free and immobilized glucose oxidase were determined by measuring the enzymatic reaction rates at different substrate concentrations ranging from 1.0 to 50 mM at the optimum reaction conditions. Michaelis constants (Km and Vmax) were calculated from the slope and intercept of the straight lines, respectively.
Results and discussion
Activities of free and immobilized enzyme
The specific activity is expressed in units/mg protein. Specific activity values found for free and immobilized GOD were shown in . The loading efficiency (percent of total enzyme immobilized), calculated according to Eq (1) was 88.7%.
Table I. Enzyme activity values of the free and immobilized GOD.
Effect of pH on free and immobilized enzyme activities
Optimum pH for free and immobilized GOD was measured through the activity of enzymes in buffers of different pH values between 2.2 and 9.9. The buffers were: pH 2.2–3.6 (50 mM, gly/HCl); pH 4.4–5.0 (50 mM, calcium acetate/acetic acid); pH 7.2–9.0 (50 mM, tris/HCl). The effect of pH on the free and immobilized GOD activities was given in . The optimum pH was 5.5 for both free and immobilized enzymes. Between pH 2.2 and 9.0, immobilized GOD was more stable than free enzyme. For instance, the free GOD showed 30% of its initial activity, while the immobilized enzyme maintained 51% of its initial activity at pH 9.0.
Effect of temperature on the free and immobilized enzyme activities
The temperature stability was determined measuring the activity values at constant pH (5.5) and varying temperatures. The effect of temperature on the free and immobilized GOD activities was shown in . The optimum temperature value for both free and immobilized GOD was 50°C. In comparison with the free enzyme, immobilized GOD showed more stability against temperature changes.
Kinetic parameters of the free and immobilized enzymes
Enzymatic kinetic parameters were investigated by changing the substrate concentration (1, 3, 5, 8, 10, 20, 30, 40 and 50 mM) at optimum pH and temperature. The effect of substrate concentration on the initial velocity of glucose oxidation for free and immobilized GOD was given in . The reaction rate increased linearly glucose concentration before reaching the plateau values and substrate inhibition occurred at higher than 40 mM. This result is consistent with the results of literature (Blandino et al. Citation2001, Rodriguez-Nogales Citation2004).
Figure 3. Effect of substrate concentration on the initial velocity of glucose oxidation. Immobilized enzyme
free enzyme.
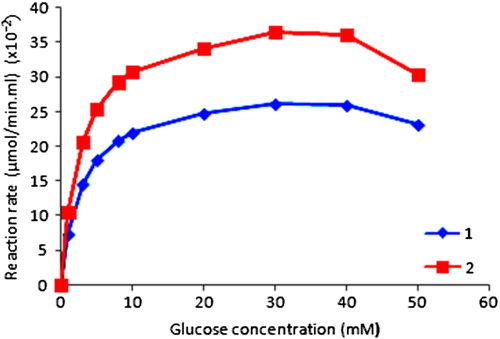
The Lineweaver-Burk plot for the free and immobilized GOD was given in . Km and Vmax values for free and immobilized GOD were shown in . This higher Km value can be attributed to the diffusional resistance of the carrier against substrate and/or product and less porous structure of the gel beads. The increase in the Km values may be also attributed to conformational changes of GOD caused by the immobilization.
Table II. Kinetic parameters of the free and immobilized GOD.
Effects of silicone oil and soybean oil on the free and immobilized enzyme activities
Oxygen vectors increase the oxygen solubility in an aqueous medium. Silicone oil and soybean oil were used as oxygen vector in this study. The effect of silicone oil on GOD activity was examined using the media containing 0, 2, 4, 6 and 8% (v/v) silicone oil, respectively. The GOD activity was maximum level at 4% silicone oil concentration. When the volumetric percentage of silicone oil was higher than 4%, there was no significant increase in the activity (). The GOD activity was increased approximately 1.7 times with respect to the media without silicone oil.
The effect of soybean oil on GOD activity was examined using the media containing 0, 2, 4, 8 and 10% (v/v) soybean oil, respectively. The activity increased approximately 1.3 times 4% soybean oil in the medium. Using soybean oil concentrations higher than 4% (v/v) resulted in significant decreases in GOD activity (). This decline may be attributed to increase in viscosity of the medium as it can cause resistance to oxygen transfer (Rols and Goma, Citation1991). It may be also attributed to substrate and reaction products diffusing in and out of the gel beads, as they were covered with soybean oil. The effect of diffusion limitation causes a more drastic decrease of enzyme activity at higher soybean oil concentrations.
Reuse number and storage stability of immobilized enzyme
The enzyme activity at optimum conditions was measured for two weeks. The activity of immobilized enzyme after repeated use was given in . The immobilized enzyme showed an excellent consistent performance up to 5 reuses and 58% of its original activity up to 7 reuses. Since no protein is found in washing and buffer solutions, the decreasing trend of enzyme activity after 7 reuses can be attributed to denaturation caused by repeated use at 50°C. At higher temperatures destabilize the enzyme and lead to thermal inactivation of GOD.
Immobilized GOD activity in 50 mM calcium acetate buffer solution at pH 5.5 was monitored during storage at 4°C for 30 days. As seen in the plot (), immobilized GOD enzyme retained 88% of its original activity after 30 days.
Conclusions
In this study; glucose oxidase (GOD) and MnO2 were successfully immobilized in alginate gel beads using the entrapment method. Immobilized GOD enzyme retained 88% of its original activity after 30 days. Since the gluconic acid formation is an oxidative process, its overall productivity strongly depends on the dissolved oxygen concentration in the reactor. When silicone oil and soybean oil were used as oxygen vector, glucose oxidase activity increased, compared to media without these oils. The GOD activity was increased approximately 1.7 times with respect to the media without silicone oil. From the achieved results, it can be concluded that immobilized alginate gels contain glucose oxidase-MnO2 and these oils could be used together for increasing gluconic acid production.
Declaration of interest The authors report no conflicts of interest. The authors alone are responsible for the content and writing of the paper.
References
- Anastassiadis S, Aivasidis A, Wandrey C. 2003. Continuous gluconic acid production by isolated yeast-like mould strains of Aureobasidium Pullulans. Appl Microbiol Biot. 61:110–117.
- Anastassiadis S, Reehm HJ. 2006. Continuous gluconic acid production by Aureobasidium Pullulans with and without biomass retention. Journal Biotecnol. 9:494–504.
- Arimatsu Y, Bao J, Furumoto K, Yoshimoto M, Fukunaga K, Nakao K. 2004. Continuous production of calcium gluconate crystals in an integrated bioreaction-crystallization process using external loop airlift bubble columns with immobilized glucose oxidase gel beads. J Chem Eng Jpn. 37:1035–1040.
- Blandino A, Macias M, Cantero D. 2001. Immobilization of glucose oxidase within calcium alginate gel capsules. Process Biochem. 36: 601–606.
- Bradford MM. 1976. A rapid and sensitive method for the quantitation of microgram quantities of protein utilizing the principle of protein-dye binding. Anal Biochem. 72:248–254.
- Holland JT, Lau C, Brozik S, Atanassov P, Banta S. 2011. Engineering of glucose oxidase for direct electron transfer via site-spesific gold nanoparticle conjugation. J Am Chem Soc. 133:19262–19265.
- Jianguo L, Zhanfeng C. 2007. Optimization of operating conditions for glucose oxidation in an enzymatic membrane bioreactor. J Membrane Sci. 302:180–187.
- Leung R, Poncelet D, Neufeld RJ. 1997. Enhancement of oxygen transfer rate using microencapsulated silicone oils as oxygen carriers. J Chem Technol Biot. 68:37–46.
- Libertino S, Aiello V, Scandurra A, Renis M, Sinetra F. 2008. Immobilization of the enzyme glucose oxidase on both bulk and porous SiO2 surfaces. Sensors. 8:5637–5648.
- Ramachandran S, Fontanille P, Pandey A, Larroche C. 2006. Gluconic acid: Properties, applications and microbial production. Food Technol Biotechnol. 44:185–195.
- Rodriguez-Nogales JM. 2004. Kinetic behaviour and stability of glucose oxidase entrapped in liposomes. J Chem Technol Biot. 79: 72–78.
- Rols JL, Goma G. 1991. Enhanced oxygen transfer rates in fermentation using soybean oil-in-water dispersions. Biotechnol Lett. 13:7–12.
- Tavcer PF. 2012. Low-temperature bleaching of cotton induced by glucose oxidase enzymes and hydrogen peroxide activators. Biocatal Biotransfor. 300:20–26.
- Tomotani EJ, Vitolo M. 2005. Immobilized glucose oxidase as a catalyst to the conversion of glucose into gluconic acid using a membrane reactor. Appl Biochem Biotech. 121:149–162.