Abstract
Bovine liver catalase was covalently immobilized onto amino acid-modified chitosan beads. The beads were characterized with SEM, FTIR, TGA and the effects of immobilization on optimum pH and temperature, thermostability, reusability were evaluated. Immobilized catalase showed the maximal enzyme activity at pH 7.0 at 30°C. The kinetic parameters, Km and Vmax, for immobilized catalase on alanine–chitosan beads and lysine–chitosan beads were estimated to be 25.67 mM, 27 mM and 201.39 μmol H2O2/min, 197.50 μmol H2O2/min, respectively. The activity of the immobilized catalase on Ala–CB and Lys–CB retained 40% of its high initial activity after 100 times of reuse.
Introduction
Catalases (H2O2:H2O2 oxidoreductase; EC 1.11.1.6) are abundant enzymes in nature that decompose hydrogen peroxide to water and molecular oxygen. Immobilized catalase can find applications in the food industry in the removal of excess H2O2 after cold pasteurization of milk and in combination with a variety of oxidase including glucose oxidase in the production of gluconic acid, removal of oxygen and/or glucose, and in the treatment of wastewater containing H2O2 (Fiedurek Citation2001, Costa et al. Citation2001, Silgia et al. Citation2002, Lida et al. Citation2000, Kubal and Souza Citation2004). With immobilized enzymes, improved stability, reusability, continuous operation, possibility of better control of reactions, high purity and product yields and hence, more favorable economic factors can be expected (Kubal and Souza Citation2004, Rasera et al. Citation2009).
Catalase has been immobilized on numerous carrier materials such as controlled pore glass, alginate bead, kappa-carragenan gel, cellulose membrane, collagen membrane, alumina, dextran, calcium hydroxyapatite, silica gel polyacrylamide gel and chitosan (Urban et al. Citation1991, Gorenek et al. Citation2004, Campanella et al. Citation1998, Selli et al. Citation1993, Vrbova et al. Citation1993, Costa et al. Citation2001, Marshall and Rabinowitz Citation1976, Kondo et al. Citation1993, Barroug et al. Citation1998, Barroug et al. Jıang and Zhang Citation1993, Çetinus and Öztop Citation2003).
Many studies have already shown chitosan to be a good support for catalase immobilization (Çetinus and Öztop Citation2003, Ran et al. Citation2010, Liu et al. Citation2010, Çetinus and Öztop Citation2000). Chitosan is a heteropolysaccharide obtained from the alkaline deacetylation of chitin, which is the most abundant polysaccharide after cellulose on earth. Chitosan is biocompatible, biodegradable, hydrophilic, non-toxic and anti-bacterial, therefore, it is widely used in water treatment, agriculture, fabric and textiles, cosmetics, nutritional enhancement and food processing (Subramanian et al. Citation2006, Pan et al. Citation2009). However, the reactivity and processability of natural chitosan are restricted: its reactive primary amino groups are directly bonded with pyranoid rings, which would cause large steric hindrance; the protonation of these amino groups leads to total or partial dissolution of chitosan in acidic mediums. In recent decades, physical or chemical modification of chitosan has been frequently conducted to broaden its applications. The physical modification could increase the adsorption properties of chitosan; the chemical modification, on the other hand, can not only increase its adsorption properties and prevent its dissolution in strong acids, but also improve the mechanical strength and superficial area (Yi et al. Citation2009, Kiyohara et al. Citation1996, Zeng and Ruckesteine Citation1996).
This paper aims to prepare a chitosan anion resin modified by glutaraldehyde and amino acids (lysine and alanine) (Yi et al. Citation2009) (). Catalase, which is frequently used in the food industry was chosen as a model enzyme for the immobilization experiment, and the kinetic parameters, operational, thermal and storage stability of the immobilized catalase in batch systems were investigated.
Figure 1. Schematic illustration for preparation of amino acid-modified chitosan beads and immobilization of enzymes (Yi et al. Citation2009).
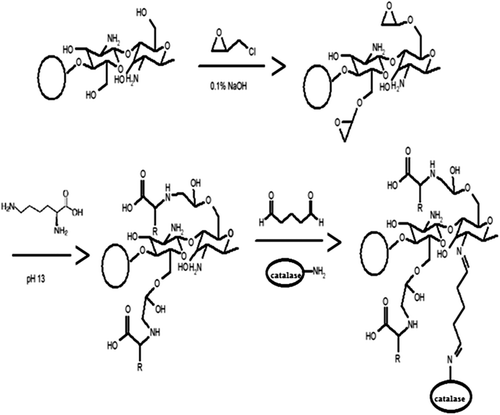
Materials and methods
Materials
Chitosan, catalase (hydrogen peroxide oxidoreductase; EC. 1.11.1.6, bovine liver), glutaraldehyde, sodium triphosphate pentabasic (TPP), alanine and lysine were obtained from Sigma Chemical Co. (St. Louis, MO, USA). Hydrogen peroxide and all other chemicals were obtained from Merck AG (Darmstadt, Germany).
Preparation of chitosan beads
To prepare the chitosan beads, 1 g chitosan flake was dissolved in acetic acid (2%, 100 mL), and then agitated by magnetic string at room temperature. After 10 min, the viscous solution was placed in an ultrasonic bath to remove air bubbles. The chitosan solution was dropped through a syringe into cold TPP solution (pH 8.2) and was stirred for 4 hour. Then, the beads were washed with deionized water until they became neutral and were stored in deionized water for use afterwards.
Modification of chitosan beads with amino acids
A schematic illustration for the modification of chitosan beads with amino acids is shown in (Yi et al. Citation2009). Kiyohara et al. reported a method for easy coupling of amino acids on a glycidyl methacrylate polymer using an epoxide ring opening reaction in aqueous solution (Yi et al. Citation2009). In order to introduce the epoxide ring onto the chitosan beads, they were first treated with excess epichlorohydrin. Zeng et al. reported that epichlorohydrin had greater reactivity toward hydroxyl groups over amine groups when reacted with chitosan under mild basic conditions (Kiyohara et al. Citation1996). Thus, the amine groups on the chitosan beads could be saved for the immobilization of catalase.
Chitosan beads (0.1 g) were treated with excess epichlorohydrin (40 mM) in 25 mL of 0.1 M aqueous NaOH for 2 hour at 50°C (Zeng and Ruckesteine Citation1996). The beads were then washed with distilled water until neutral. The epichlorohydrin activated chitosan beads (0.1 g) were then treated with alanine and lysine (40 mM, 25 mL) for 24 hour at 50°C in aqueous NaOH (pH 13) (Kiyohara et al. Citation1996). The beads were then washed with distilled water until neutral. The washed wet beads were used in catalase immobilization.
Immobilization of catalase on amino acid-modified chitosan beads
Amino acid modified chitosan beads (0.1 g) were treated with 100 mL of 2% glutaraldehyde in phosphate buffer (50 mM, pH 7.0) for 2 hour at 30°C, and washed with distilled water. Then, the activated beads were incubated with 4 mL of catalase (0.1 mg) solution in phosphate buffer (50 mM, pH 7.0) for 4 hour at 4°C (Yi et al. Citation2007). Finally, the immobilized catalase on chitosan beads were filtered and washed with distilled water. The amount of immobilized protein was determined according to Bradford's method. Bovine serum albumin was used as the standard (Bradford Citation1976).
Activity assays of catalase
The activity of catalase was determined spectrophotometrically at 240 nm by measurement of decrease in the absorbance of hydrogen peroxide owing to its decomposition by the enzyme. Catalase immobilized chitosan beads (25 mg) were mixed with 3 mL of hydrogen peroxide solution (10 mM). After 3 min, the reaction was stopped by removal of the beads from reaction mixture. The absorbance of the reaction mixture was measured and activity of immobilized catalase was calculated. One unit of activity is defined as the decomposition of 1 μmol hydrogen peroxide per min at 25°C and pH 7.0. Activity of free catalase was given as U and immobilized catalase activities were expressed as U/g carrier.
The effect of substrate concentration on the activity was tested by using increasing concentrations of H2O2, Vmax and Km values of immobilized and free catalase were determined.
Effect of pH and temperature of free and immobilized catalase
pH stabilities of free and immobilized catalase were examined by measuring the activity of enzyme after the enzyme had been incubated in the solutions for 60 min at pH 4.0–9.0. For determination of thermal stability, either free or immobilized catalase was incubated in phosphate buffer (50 mM, pH 7.0) for 60 min at 20–60°C. Activity of samples were performed under optimum conditions.
Storage stabilities of free and immobilized catalase
Storage stability of the free and immobilized catalase was investigated by measuring their activities after being stored at 4°C and 25°C for 70-day periods and the remaining activity measurement was performed once a week.
Operational stability of the immobilized catalase
In order to show the operational stability of the immobilized catalase, activity was examined under batch operation mode at 25°C at intervals of 3 min. After each activity measurement, the immobilized catalase was separated from the reaction mixture and washed three times with phosphate buffer (50 mM, pH 7.0) and then a fresh reaction medium was introduced onto the immobilized catalase. By this way, the next activity measurement was carried out.
Characterization of chitosan and modified chitosan beads
Fourier transform infrared (FTIR) analysis was accomplished on a Perkin Elmer spectrum BX scanning from 4000 to 400 cm‐1 at room temperature. Chitosan, chitosan– glutaraldehyde, chitosan–alanine–glutaraldehyde, chitosan–lysine–glutaraldehyde were mixed with KBr and pressed to plates for measurements.
The weight loss temperatures of the chitosan and modified chitosan beads were determined with a Mettler Toledo TGA analyzer (TGA/SDTA851e).
The surface morphology of the beads was examined using scanning electron microscopy (SEM) (XL30-SFEG, FEI/Philips).
Results and discussion
Characterization of enzyme immobilization matrix
The surface morphologies of chitosan beads, lysine modified chitosan beads, alanine modified chitosan beads and immobilized catalase on chitosan and amino acid modified chitosan beads were exemplified by the electron micrographs in . As the surface morphologies of amino acid modified chitosan beads and catalase immobilized chitosan and amino acid modified chitosan beads (Figures 2B–2F) were compared with that of original chitosan (), it is clearly seen that the surface morphologies of chitosan were affected from modification, activation and immobilization procedures.
Figure 2. Scanning electron micrographs of the surfaces of (A) chitosan bead, (B) lysine-modified chitosan bead, (C) alanine-modified chitosan bead, (D) catalase-immobilized chitosan bead, (E) catalase-immobilized Lys–CB, (F) catalase-immobilized Ala–CB.
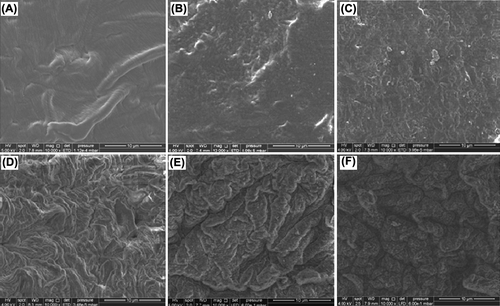
The FTIR spectra corresponding to chitosan– glutaraldehyde (CGA), chitosan–alanine–glutaraldehyde (Ala–CGA) and chitosan–lysine–glutaraldehyde (Lys–CGA) are shown in . As seen in FTIR of CGA, the peak around 3400 cm‐1 observed to the –OH group. The C–H stretching vibration of the polymer backbone is manifested through strong peaks at 2925 and 2855 cm‐1. The stretching vibrations of C–O are found at 1084 cm‐1. The characteristic adsorption bands appeared at 1589 cm‐1 which can be assigned to N–H bending vibration, and peaks appearing at 1399 cm‐1 to –C–O stretching of the primary alcoholic group in chitosan. For cross-linked chitosan beads, the peak at 1656 cm‐1 can be observed in FTIR of CGA, Ala-CA and Lys-CGA, which corresponds to stretching vibrations of the C = N bond. This peak indicated the formation of Schiff's base as a result of the reaction between the carbonyl group of glutaraldehyde and the amine group of chitosan chains.
The thermal stability of the chitosan beads and alanine-modified chitosan beads (Ala–CB) has been examined using thermogravimetric analysis and results are shown in . All thermal decomposition profiles exhibit two main stages, with one starting at around 100°C and another at 250°C. The maximum mass losses observed around 210.35 and 288.48°C are for chitosan and alanine–chitosan, respectively. Amino acid modification of chitosan beads turns the chitosan more resistant to degradation when compared to the chitosan.
Enzyme immobilization
Catalase immobilized on chitosan and modified chitosan beads with different amounts of glutaraldehyde (1–5%) and different cross-linking durations (60–300 min) during immobilization process. 120 min was considered as a sufficient coupling time when compared with the other tested coupling times (60, 180, 240, 300 min). Both the immobilized enzymes on Ala–CB and Lys–CB reach a maximum at 2% glutaraldehyde concentration. The loss of the activity of the immobilized catalase on Ala–CB is smaller than that of Lys–CB at high glutaraldehyde concentration. Therefore, the glutaraldehyde concentration and cross-linking time are selected to be 2% and 120 min, respectively.
Kinetic parameters, optimum temperature and optimum pH
Kinetic constants that might be expected to be changed on immobilization were determined for immobilized catalase preparations and results are given in . Km value (33.76 mM) for catalase immobilized on chitosan beads was higher than that of the free catalase (14.28 mM). Km for catalase immobilized on Ala–CB (25.67 mM) and Lys–CB (27.0 mM) were also higher than Km for free catalase (14.28 mM). As shown from , the Vmax of immobilized catalase on chitosan(141.9 μmol H2O2/min), Ala–CB (201.39 μmol H2O2/min) and Lys–CB (197.50 μmol H2O2/min) were smaller than that of free catalase (3076.92 μmol H2O2/min). There is a significant difference between Vmax of free catalase and Vmax of immobilized catalases. Obtained Km and Vmax for catalase chitosan and amino acid-modified chitosan beads suggest that there were diffusional constrains in the former case while enzyme has undergone a conformational change during immobilization onto chitosan beads (Dwevedi and Kayastha Citation2009, Seong et al. Citation1981). Çetinus and Öztop (Citation2003) immobilized bovine liver catalase on glutaraldehyde-pretreated chitosan films. They found Km = 25.16 mM, Vmax = 24042 μmol H2O2/mg protein min for free catalase, Km = 27.67 mM, Vmax = 1022 μmol H2O2/mg protein min for immobilized catalase on chitosan. Chatterjee et al. (Citation1990) immobilized goat liver catalase on or in various carriers. They reported Km values of free, alumina-, gelatine-, polyacrylamide- and egg shell-immobilized catalases as 110, 200, 140, 200 and 67 mM, respectively.
Table I. Kinetic parameters of free and immobilized catalases.
The effect of temperature on the activity of the free and immobilized catalase on chitosan beads was investigated in the temperature range of 5–60°C at pH 7.0. It was found that free catalase and immobilized catalase on chitosan beads showed the highest relative activity at 35°C. However, the immobilized catalase on Ala–CB and Lys–CB exhibited highest relative activities between 30 and 40°C. Amino acid modified chitosan beads were more stable at higher temperatures than free and immobilized catalase on chitosan beads. Çetinus and Öztop (Citation2003) reported that immobilization of catalase onto the chitosan film did not change the optimum temperature. Arıca et al. (Citation1997) reported a slight increase (5°C) in the optimum temperature of the immobilized catalase preparations. Jıang and Zhang (Citation1993) reported that immobilization of catalase by gel entrapment does not greatly change the temperature activity profile and they reported more pronounced temperature dependence for catalase entrapped in polyacrylamide gel.
The effect of pH on the activity of free and immobilized catalases was studied in the pH range of 4.0–9.0. Free catalase and immobilized catalases showed their maximum activity at pH 7.0. Relative activities of immobilized catalases on Ala–CB and Lys–CB were 83 and 81% at pH 6.0, respectively. At pH 8.0, relative activity of Ala–CB was 73% whereas the relative activity of Lys–CB was 78%. The loss of activity of Ala–CB was higher than Lys–CB at alkaline pHs. Immobilized catalase was less sensitive to pH changes at alkaline pHs than acidic pHs. Costa et al. (Citation2001) reported that treatment of the immobilized preparations with glutaraldehyde further increased their ability to retain enzymatic activity at alkaline pHs.
pH, thermal and storage stabilities
pH stability studies were accomplished for free and immobilized catalases, which were incubated at various pH values for 60 min. As seen in , the immobilized enzymes on Ala–CB and Lys–CB showed more pH stability than free enzyme and unmodified chitosan beads. Catalase immobilized on modified chitosan beads was more stable in the pH range from 4.0 to 9.0. Generally the profiles of the immobilized and free catalase are similar, but the immobilized catalase is generally more stable at higher values of pH (Costa et al. Citation2001, Acosta et al. Citation2001).
Figure 4. pH (A) and temperature (B) stability of free and immobilized catalase at various pH and temperature after 60 min (



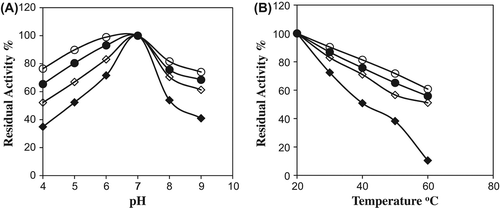
The thermal stabilities of free and immobilized enzymes were studied at different temperatures at 20–60°C (). Residual activities for the free and immobilized catalases on Ala–CB, Lys–CB were found to be 38% and 81%, 65% of their original activities, respectively, at 50°C for a 60-min incubation period. Free catalase retained about 10% of its original activity at 60°C for 60-min incubation period. Immobilized catalase on Ala–CB and Lys–CB protected about 60 and 55% of its original activity for the same period at 60°C, respectively. The results showed that the thermal stability of the immobilized catalase on Ala– CB and Lys–CB was greater than that of the free catalase or unmodified chitosan beads. Horst et al. (Citation2006) immobilized catalase on glutaraldehyde-treated magnetite (Fe3O4). They reported that the thermal stability of immobilized catalase was lower than the free catalase.
The storage stability of immobilized catalase was greater than that of free catalase both at room temperature and at 4°C. Free catalase completely lost its initial activity at the end of 21 days at room temperature and 42 days at 4°C. However, Ala–CB and Lys–CB lost their initial activities at the end of 49 days at room temperature and at 4°C at the end of 70 days. It is obvious that there is a remarkable difference in the activity retentions with storage time. In other words, catalase immobilized onto amino acid- modified chitosan beads showed high stability, whereas free catalase lost most of its initial activity very soon. Çetinus and Öztop (Citation2003) immobilized catalase on glutaraldehyde-pretreated chitosan films. They reported that the free enzyme retained about 50% of its activity for 18 days, immobilized catalase stored wet retained about 50% of its activity for 25 days and when stored dry, retained about 50% of its activity for 5 days at 5°C. Silva et al. (Citation1991) reported that the covalent immobilization of catalase on the Teflon membrane showed an excellent lifetime of more than 4 months, the membrane being stored at room temperature.
Operational stability
The stability of immobilized enzyme systems is very important for various biotechnological applications; an increased stability could make the immobilized enzyme more advantageous than its free counterparts. The main advantage of the immobilized enzyme is its reusability. Operational stabilities of immobilized catalase preparations were determined and results presented in . The activity decays exponentially with time in both preparations. The remaining activity of the catalase immobilized on Ala–CB and Lys–CB was about 40% after 100 cycles of batch operation.
Conclusions
Modification of chitosan was successfully carried out with alanine, lysine and glutaraldehyde for covalent immobilization of catalase. Amino acid-modified chitosan beads were developed in order to improve the activity of immobilized catalase on chitosan beads. The amino acids were introduced onto the chitosan beads after activation with epichlorohydrin. The immobilized catalase on amino acid-modified chitosan beads showed higher activities than that of unmodified chitosan beads. The pH value for optimum activity of catalase was not affected by the immobilization. The immobilized catalase on Ala–CB and Lys–CB showed optimal conditions at 35°C and pH 7.0, and the property of improved stability in a broader temperature and pH range was very useful for its application in the food industry. The reusability of the immobilized catalase was tested and it was confirmed that the washing procedure was important. The reusability of modified chitosan beads may provide economic advantages for large-scale biotechnological applications. We also confirmed that the amino acid-modified chitosan beads were a good support for the immobilization of catalase and that they could be applied for immobilization of other useful enzymes.
Declaration of interest The authors report no conflicts of interest. The authors alone are responsible for the content and writing of the paper.
This work was supported by Research Grants BAP 2010-106 from Celal Bayar University.
References
- Acosta M, Garc´ıa C´anovas F, Hiner AN, Arnao MB, Hern´andez Ruiz J. 2001. Catalase-like activity of horseradish peroxidase, relationship to enzyme inactivation by H2O2. Biochem J. 354:107–114.
- Arıca MY, Denizli A, Salih B, Piskin E, Hasirci V. 1997. Catalase adsorption onto Cibacron Blue F3GA and Fe(III)-derivatized poly(hydroxyethyl metacrylate) membranes and application to a continuous system. J Membr Sci. 129:65–76.
- Barroug A, Lernoux E, Lemaitre S, Rouxhet PG. 1998. Adsorption of catalase on hydroxyapatite. J Colloid Interface Sci. 208:147–152.
- Bradford M. 1976. A rapid and sensitive method for the quantitation of microgram quantities of protein utilizing the principle of protein-dye binding. Anal Biochem. 72:248–254.
- Campanella L, Roversi R, Sammartino MP, Tomassetti M. 1998. Hydrogen peroxide determination in pharmaceutical formulations and cosmetics using a new catalase biosensor. J Pharm Biomed Anal. 18:105–116.
- Çetinus Ş, Öztop H. 2000. Immobilization of catalase on chitosan film. Enzyme Microb Technol. 26:497–501.
- Çetinus Ş, Öztop H. 2003. Immobilization of catalase into chemically crosslinked chitosan beads. Enzyme Microb Technol. 32:889–894.
- Chatterjee U, Kumar A, Sanwal GG. 1990. Goat liver catalase immobilization on various solid supports. J Ferment Bioeng. 70: 429–430.
- Costa SA, Tzanov T, Paar A, Gudelj M, Gubitz GM, Cavaco PA. 2001. Immobilization of catalase from Bacillus SF on alumina for the treatment of textile bleaching effluents. Enzyme Microb Technol. 28:815–819.
- Dwevedi A, Kayastha A. 2009. Optimal immobilization of b-galactosidase from Pea (PsBGAL) onto Sephadex and chitosan beads using response surface methodology and its applications. Bioresour Technol. 100:2667–2675.
- Fiedurek J. 2001. Production of gluconic acid by immobilized mycelium of Aspergillus niger in pumice Stones using unconventional oxygenation of culture. Biotechnol Lett. 23:1789–1792.
- Gorenek G, Akyilmaz E, Dinckaya E. 2004. Immobilization of catalase by entrapping in alginate beads and catalase biosensor preparation for the determination of hydrogen peroxide decomposition. Artif Cells Blood Substit Biotechnol. 32:453–461.
- Horst F, Rueda EH, Ferreira ML. 2006. Activity of magnetite- immobilized catalase in hydrogen peroxide decomposition. Enzym Microbi Technol. 38:1005–1012.
- Jıang B, Zhang Y. 1993. Immobilization of catalase on crosslinked polymeric hydrogels-effect of anion on the activity of immobilized enzyme. Eur Polym J. 29:1251–1254.
- Kiyohara S, Sasaki M, Saito K, Sugita K, Sugo T. 1996. Amino acid addition to epoxy-group-containing polymer chain grafted onto a porous membrane. J Membr Sci. 109:87–92.
- Kondo A, Murakami F, Kawagoe M, Higashitani K. 1993. Kinetic and circular dischroism studies of enzymes adsorbed on ultrafine silica particles. Appl Microbiol Biotechnol. 39:726–731.
- Kubal B, Souza S. 2004. Immobilization of catalase by entrapment of permeabilized yeast cells in hen egg white using glutaraldehyde. J Biochem Biophys Meth. 59:61–64.
- Lida T, Maruyama D, Fukunaga K. 2000. Stabilization of entrapped catalase using photo cross-linked resin gel for use in wastewater containing H2O2. J Chem Technol Biotechnol. 75:1026–1030.
- Liu Y, Jia S, Ran J, Wu S. 2010. Effects of static magnetic field on activity and stability of immobilized a-amylase in chitosan bead. Catal Commun. 11:364–367.
- Marshall JJ, Rabinowitz ML. 1976. Stabilization of catalase by covalent attachment to dextran. Biotechnol Bioeng. 18:1325–1329.
- Pan C, Hu B, Li W, Sun Y, Ye H, Zeng X. 2009. Novel and efficient method for immobilization and stabilization of β-D-galactosidase by covalent attachment onto magnetic Fe3O4-chitosan nanoparticles. J Mol Catal B Enzym. 61:208–215.
- Ran J, Jia S, Liu Y, Zhang W, Wua S, Pan X. 2010. A facile method for improving the covalent crosslinking adsorption process of catalase immobilization. Bioresour Technol. 101:6285–6290.
- Rasera K, Ferla J, Dillon A, Riveiros R, Zeni M. 2009. Immobilization of laccase from Pleurotus sajor-caju in polyamide membranes. Desalination. 245:657–661.
- Selli E, D’Ambrosio A, Bellobono IR. 1993. Enzymatic activity under tangential flow conditions of photochemically grafted membranes containing immobilized catalase. Biotechnol Bioeng. 41:474–478.
- Seong BL, Han MH, Park JM, Choi CY. 1981. A graphical method of determining the Michaelis–Menten constant free of external mass transfer resistance for immobilized enzymes in a packed bed reactor. Biotechnol Lett. 3:607–612.
- Silgia AC, Tzanko T, Filipa C, George MG, Arthur CP. 2002. Recycling of textile bleaching effluents for dying using immobilized catalase. Biotechnol Lett. 24:173–176.
- Silva MAD, Gil MH, Piedade AP, Redinha JS, Brett AMO, Costa JMC. 1991. Immobilization of catalase on membranes of poly(ethylene)-g-co-acrylic acid and their application in hydrogen peroxide electrochemical sensors. J Polym Sci Part A Polym Chem. 29:269–274.
- Subramanian A, Rau A, Kaligotla H. 2006. Surface modification of chitosan for selective surface-protein interaction. Carbohydr Polym. 66:321–332.
- Urban G, Kamper H, Jachimowicz A, Kohl F, Kuttner H, Olcaytug F, et al. 1991. The construction of microcalorimetric biosensors by use of high resolution thin-film thermistors. Biosens Bioelectron. 6:275–280.
- Vrbova E, Peckova J, Marek M. 1993. Biosensor for determination of starch. Starch. 45:341–344.
- Yi SS, Lee C, Kim J, Kyung D, Kim BG, Lee YS. 2007. Covalent immobilization of w-transaminase from vibro fluvialis JS17 on chitosan beads. Proc Biochem. 42:895–898.
- Yi S, Noh J, Lee Y. 2009. Amino acid modified chitosan beads: improved polymer supports for immobilization of lipase from Candida rugosa. J Mol Catal B Enzym. 57:123–129.
- Zeng X, Ruckesteine E. 1996. Control of pores in macroporous chitosan and chitin membranes. Ind Eng Chem Res. 35:4169–4175.