Abstract
Introduction. The effect of aging on plasma-protein, lipid and DNA oxidation is well documented. However, none of the studies specify the effect of gender. The purpose of this study is to clarify the ambiguity raised in preliminary reports as to gender dependency of oxidative damage in plasma.
Methods. In the current study, we investigated the relation between 8-hydroxy-2′-deoxyguanosine levels (8-OHdG), which is a measure of DNA oxidation and protein oxidation parameters such as protein carbonyl (PCO), total thiol (T-SH), and advanced oxidation protein products (AOPP). Our study also covered other oxidative stress parameters, such as lipid hydroperoxides (LHP), malondialdehyde (MDA), erythrocyte glutathione (GSH), superoxide dismutase (Cu-Zn SOD) and the catalase (CAT) activity in plasma of the male and female aged rats.
Results. 8-OHdG and MDA levels in male rats were significantly higher than those in the female group (p < 0.01 for both parameters). T-SH levels were found to be higher in female rats than in the male (p < 0.05). Plasma Cu-Zn SOD activities of male rats were significantly higher compared with those of the female rats (p < 0.05). On the other hand, PCO, AOPP, LHP, GSH levels, and CAT activity were not found to be different between genders.
Conclusions. We suggest that increased T-SH levels found in female rats may point to an adaptive reaction to oxidative damage, reflecting 8-OHdG and MDA overproduction. We are of the conviction that the increased 8-OHdG and MDA that we have determined in aged male rats may be a risk factor in the extent of oxidation in plasma.
Introduction
For the past several decades, the world has experienced falling fertility and increasing longevity, two demographic indicators that reveal the world is filled with an aging population. On the other hand, the molecular basis of the difference in life expectancy between males and females is still unknown. The gender-related homeostatic and redox-dependent molecular mechanisms responsible for such difference are still obscure and controversial.
Free radicals; reactive oxygen species (ROS) and reactive nitrogen species (RNS) are highly reactive molecules or molecular fragments containing one or more unpaired electrons in atomic or molecular orbitals. ROS and RNS can be harmful as they can damage cellular lipids, sugars, proteins and nucleic acids, thus inhibiting the latter's normal function [Citation1]. This oxidative damage can compromise cell viability or induce cellular responses leading to cell death by necrosis or apoptosis [Citation2]. The damage promoted by ROS and RNS is termed oxidative stress and nitrosative stress, respectively. Efficient regulation of ROS/RNS production and neutralization is essential for avoiding their detrimental effects, and different molecular mechanisms co-operate to preserve this equilibrium termed ‘redox balance’ or ‘redox homeostasis’. It has been proposed that 0.2–2% of the total oxygen consumption is converted into free radicals in mitochondria. During energy transduction, a small number of electrons ‘leak’ out from oxygen prematurely, thereby forming ROS, which is mainly the oxygen free radical superoxide (O2·−) [Citation3].
Aging is the progressive deterioration in various physiological functions including vascular metabolic process [Citation4,Citation5]. ROS and RNS are known to contribute to altered redox-homeostasis of macromolecules in endothelial cells and consequent vascular senescence [Citation4]. On the other hand, multiple extracellular and intracellular redox-dependent pathways are known to contribute individually and synergistically to the progressive decline of tissue and organ functions. Important oxidative alterations in the structure and function of endothelial cells accompany advancing age [Citation5]. These age-associated alterations in the endothelial cells of the arterial wall are favorable to the initiation or progression of superimposed vascular diseases. Although all these changes in vascular structure and function were previously thought to be part of chronological aging, this concept needs to be revisited in view of novel emerging data. These vascular changes are accelerated in the presence of elevated levels of oxidized proteins [Citation6,Citation7], lipids [Citation6–8] and DNA [Citation9,Citation10] in aging plasma. The gender-dependent factors responsible for such redox-dependent variations of the level of oxidized macromolecules are largely unknown and controversial. The ambiguity arose in preliminary reports as to gender-dependent variations of the levels of main oxidative-stress parameters in plasma of aged subjects. However, the multiple relationships among gender and all these oxidized macromolecules in this regard could not be meaningfully correlated in previous studies [Citation4,Citation5,Citation9]. We hope the present study will provide an insight that would clarify the complex issue. In the current study, we investigated the relation between 8-hydroxy-2′-deoxyguanosine levels (8-OHdG), which is a measure of DNA oxidation and protein oxidation parameters such as protein carbonyl (PCO), total thiol (T-SH), and advanced oxidation protein products (AOPP). Our study also covered other oxidative stress parameters, such as lipid hydroperoxides (LHP), malondialdehyde (MDA), erythrocyte glutathione (GSH), Cu-Zn superoxide dismutase (Cu-Zn SOD) and the catalase (CAT) in plasma of the male and female aged rats.
It is well known that elderly people in both sexes who have generally suffered from various aging-related disorders or may be using some medication which interfere with the accuracy of the result compel us to prefer to apply the same procedures in aged rats. Our aim was to bring out the difference between broad set of oxidative stress parameters of male and female rats of the same chronological age in order to understand the gender-dependent homeostatic mechanisms enabling females to live longer than males.
Methods
Experimental animals and procedures
Aged Wistar rats (24 months) supplied by the Centre for Experimental and Applied Medical Research, Istanbul University, Istanbul, Turkey were used. Rats were divided into two groups as male (n = 9) and female (n = 9).
These animals were housed in conventional wire-mesh cages, four rats per cage, in a room with the temperature regulated at 21 ± 1°C, humidity 45–50%, and light/dark cycles (12 h). All animals were given ad libitum access to standard food and tap water by drinking bottle throughout the course of the experiment. The experimental protocol was approved by the University of Istanbul, Animal Research Committee in accordance with the NIH Guide for the Care and Use of Laboratory Animals.
Sample collection
All blood samples were taken in the morning. Male and female aged rats were fasted and blood was obtained from the heart aorta under deep anaesthesia with sodium pentothal (Abbott, Campoverde di Aprilia, Italy, 100 mg/kg). Samples were drawn in the fasting state and processed within 1 h of collection. Blood samples were collected in tubes containing lithium heparin, ethylenediaminetetraacetic acid (EDTA) or no additive depending on the analysis. For the oxidative stress parameters, plasma samples containing lithium heparin and EDTA were stored at −70°C until analysis. All other parameters such as erythrocyte GSH and total protein levels were determined in a same-day analysis.
Analytical methods
Apparatus
All the samples were centrifuged at +4°C (Jouan G 412). Plasma PCO, T-SH, AOPP, LHP, MDA and erythrocyte GSH levels were measured by a UV-visible spectrophotometer (Heraeus 400, Kendro Laboratory Product, Osterode, Germany). Plasma 8-OHdG measurements were performed by an ELISA system (Organon Teknika, Boseind, the Netherlands).
Chemicals
All kinds of chemical reagents were analytical grade. Deionized water was used in entire analytical procedures. The reagents were stored at +4°C and equilibrated at room temperature for 0.5 h before use.
Assay of plasma 8-hydroxy-2-deoxyguanosine levels
8-OHdG levels of the plasma samples were determined using an enzyme-linked immunosorbent assay detection kit (OXIS, Bioxytech, EIA, USA). The coefficients of intra- and interassay variations for 8-OHdG assay were 6.8% (n = 10) and 7.1% (n = 10), respectively.
Assay of plasma protein carbonyl levels
PCO groups were measured spectrophotometrically by using the method of Reznick and Packer [Citation11]. PCO groups react with 2,4-dinitrophenylhydrazine (DNPH) to generate chromophoric dinitrophenylhydrazones. DNPH was dissolved in HCl, and after the DNPH reaction, proteins were precipitated with an equal volume of 20% (w/v) trichloroacetic acid and washed three times with 4 mL of an ethanol/ethyl acetate mixture (1:1). Washings were achieved by mechanical disruption of pellets in the washing solution using a small spatula, and re-pelleting by centrifugation at 6000 g for 5 min. Finally, the protein precipitates were dissolved in 6 M-guanidine-HCl solution and the absorbances were measured at 360 nm using the molar extinction coefficient of DNPH, ε = 2.2 × 104 M−1 × cm−1. Protein contents were determined on the HCl blank pellets spectrophotometrically using a Folin kit (Sigma Diagnostics, St.Louis, MO). The coefficients of intra- and inter-assay variations for carbonyl assay were 5.2% (n = 12) and 9.3% (n = 10), respectively.
Assay of plasma total thiol levels
Plasma T-SH concentration was determined by using 5,5′-dithio-bis(2-nitrobenzoic acid) (DTNB) as described by Hu [Citation12]. The coefficients of intra- and inter-assay variations were 1.8% (n = 10) and 4.6% (n = 9), respectively.
Assay of advanced protein oxidation products
Spectrophotometric determination of plasma AOPP levels was performed by modification of Witko–Sarsat's method [Citation13]. Samples were prepared in the following way: 200 μL of plasma was diluted 1:5 in Phosphate-buffered saline (PBS), 10 μL of 1.16 M potassium iodide was then added to each tube, 2 min later followed by 20 μL acetic acid. The absorbance of the reaction mixture was immediately read at 340 nm against a blank containing 2000 μL of PBS, 100 μL of KI, and 200 μL of acetic acid. The coefficients of intra- and inter-assay variations were 1.6% (n = 10) and 2.4% (n = 10), respectively. The chloramine-T absorbance at 340 nm being linear within the range of 0–100 μmol/L, AOPP concentrations were expressed as micromoles per liter of chloramine-T equivalents.
Assay of plasma lipid hydroperoxide levels
Plasma LHP levels were determined spectrophotometrically according to the method of ferrous oxidation with xylenol orange version 2 (FOX2) [Citation14]. Lipid hydroperoxides oxidize ferrous to ferric ions selectively in dilute acid and the resultant ferric ions can be determined by using ferric-sensitive dyes as an indirect measure of hydroperoxide concentration. Xylenol orange binds ferric ions with high selectivity to produce a coloured (blue-purple) complex with an extinction coefficient of 1.5 × 104 M−1 × cm−1. Ninety microliter aliquots of plasma were transferred into microcentrifuge reaction vials. Triphenylphosphine (TPP) (10 mM in methanol; 10 μL) was added to vials to remove hydroperoxides. Methanol alone (10 μL) was added to the remaining vials. The LHP content in the plasma samples is determined as a function of the absorbance difference of samples with and without elimination of LHPs by TPP. The samples were vortexed and subsequently incubated at room temperature for 30 min. FOX2 reagent (900 μL) was added and the samples were vortexed. After incubation at room temperature for 30 min, the samples were centrifuged at 15,000g at 20°C for 10 min, the supernatant was carefully decanted into a cuvette, and absorbance was read at 560 nm. The coefficients of intra- and inter-assay variations were 2.4% (n = 12), and 2.3% (n = 11), respectively.
Determination of thiobarbituric acid reactive substances
The rate of lipid peroxidation was determined by the procedure of Beuge and Aust [Citation15]. One of the major secondary products of lipid peroxidation is malondialdehyde (MDA). MDA along with other by-products reacts with thiobarbituric acid (TBA) to generate a colored product which absorbs at 535 nm representing the color produced by all the thiobarbituric acid reactive substances (TBARS). The coefficients of intra- and inter-assay variations for MDA assay were 3.5% (n = 10) and 5.4% (n = 10), respectively.
Determination of reduced glutathione levels
Whole blood reduced glutathione (GSH) levels were measured by a commercially available GSH assay kit (Cayman Chemical, Ann Arbor, MI). GSH assay kit utilizes enzymatic recycling method using glutathione reductase for the quantification of GSH. The thiol group of GSH reacts with DTNB (5-5′-dithiobis-2-nitrobenzoic acid, Ellman's reagent) and produces a yellow colored 5-thio-nitrobenzoic acid (TNB). The mixed disulfide, GSTNB that is concomitantly produced, is reduced by glutathione reductase to recycle the GSH and produce more TNB. The rate of TNB production is directly proportional to the concentration of GSH in the deproteinized sample. Measurement of the absorbance of TNB at 414 nm provides an accurate estimation of GSH in the sample.
Determination of plasma Cu-Zn SOD activity
Determination of Cu-Zn SOD (EC 1.15.1.1) activity was performed in plasma specimens based on the method developed by Sun et al. [Citation16]. Xanthine and xanthine oxidase were used to generate O2·− and nitroblue tetrazolium (NBT), which reacts with O2·− to form a nitroblue dye used as a detector. SOD inhibits the formation of the nitroblue dye, and the Cu-Zn SOD activity was measured as a percent inhibition compared with a calibration curve with purified SOD. The amount of superoxide dismutase that inhibits the rate of the NBT dye formation by 50% is defined as 1U.
Determination of plasma catalase activity
The activity of catalase (EC 1.11.1.6) was determined by the method of Yasmineh et al., by measuring the rate of decomposition of hydrogen peroxide [Citation17]. One catalase unit is the amount of catalase decomposing 1.0 μmol of hydrogen peroxide per minute at pH 7.0 at 25°C.
Statistical analysis
Computer program In-Stat was used for data analysis. Descriptive statistics were given as mean ± standard error of the mean (SEM). All data were not normally distributed. All parameters of the aged rats were compared using the Mann–Whitney U-test. When not specified, p < 0.05 was considered significant.
Results
In the observations of our experiments conducted during 2007–2009 within the animal husbandry conditions specified by the Istanbul University, Centre for Experimental and Applied Medical Research, the median life-span of the male Wistar rats were found to be 25 months and their maximum life-span 28 months; on the other hand, the median life-span of the female Wistar rats turned out to be 27 months and their maximum life-span 29 months. The life-span of the aged rats was observed within the same group of rats.
Plasma protein oxidation parameters in the study groups are given in . Plasma PCO and AOPP levels of male rats were not significantly higher compared with those of the female rats (p > 0.05, respectively). There was a trend toward higher PCO levels in female rats compared to male rats, but this did not reach statistical significance (). T-SH levels of the plasma were significantly lower in the aged male rats compared with those of the female rats (p < 0.05). Concentrations of the lipid peroxidation markers in the plasma of male and female rats are given in . MDA levels were found to be significantly higher in male rats when compared to those in female rats. On the other hand, plasma LHP levels were not found to be different between genders. Although there was a trend toward higher erythrocyte GSH levels in female rats compared to those in male rats, GSH levels were not found to be significantly higher in female rats when compared with those in male rats. Plasma Cu-Zn SOD activity of male rats was significantly higher compared with those of the female rats (p < 0.05). CAT activities were not found to be significantly different between genders (). shows the effect of gender on plasma oxidative DNA damage of aged rats wherein there was a significant increase in 8-OHdG (p < 0.01) in aged male rats.
Figure 1. Concentrations of the protein oxidation markers in the plasma of male (n = 9) and female (n = 9) aged rats. Results are expressed as mean ± S.E.M. Data are statistically different between genders. (*) p < 0.05, PCO: protein carbonyl; AOPP: advanced oxidation protein products; T-SH: total thiol; pr: protein.
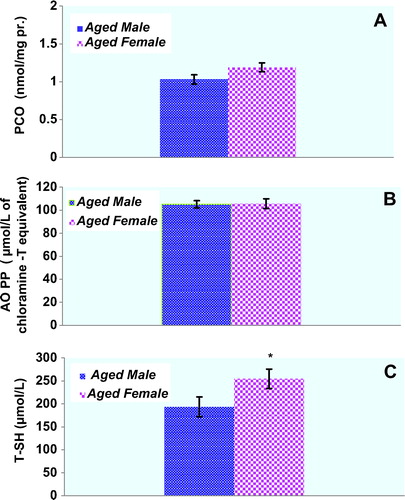
Figure 2. Concentrations of the lipid peroxidation markers in the plasma of male (n = 9) and female (n = 9) aged rats. Results are expressed as mean ± SEM. Data are statistically different between genders. (**) p < 0.01. MDA: malondialdehyde; LHP: lipid hydroperoxides; pr: protein.
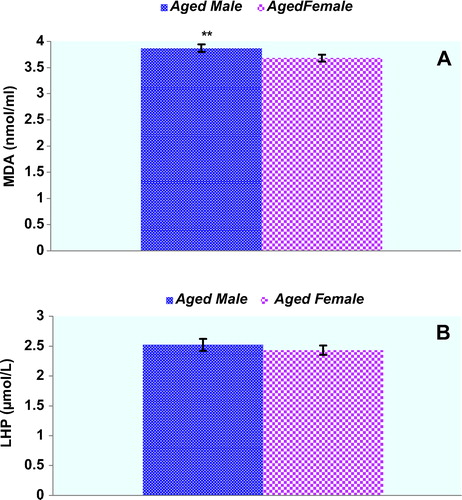
Figure 3. Erythrocyte GSH levels and plasma antioxidant enzyme activities in the study groups. Results are expressed as mean ± SEM. Data are statistically different between genders. (*) p < 0.05, GSH: glutathione, Cu-Zn SOD: superoxide dismutase, CAT: catalase.
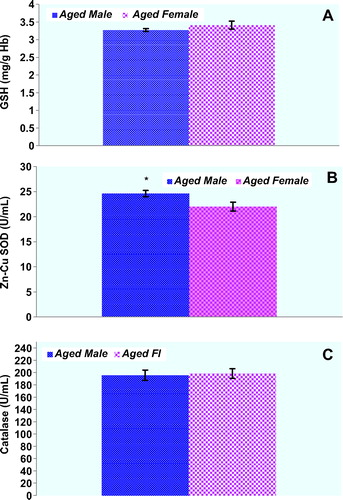
Figure 4. Plasma 8-OHdG levels in the study groups. Results are expressed as mean ± SEM. Data are statistically different between genders. (**) p < 0.01, 8-OHdG: 8-hydroxy-2′-deoxyguanosine.
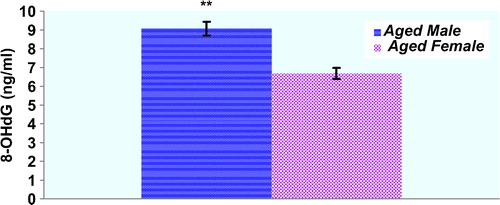
Spearman's rank correlation coefficients among various oxidative stress markers in the whole series of male and female rats are given in , respectively. In plasma of male rats, 8-OHdG levels were significantly correlated with MDA and T-SH levels. On the other hand, plasma MDA levels were significantly correlated with T-SH levels in male rats (). Spearman's rank correlation coefficients among the oxidative stress parameters except the MDA/TSH couple were not significantly correlated in female rats (MDA/8-OHdG, T-SH/8-OHdG, for both couple p > 0.05), (). Cu-Zn SOD activity of the both group of rats were not correlated with other oxidative stress parameters.
Discussion
It is well known that gender is the main risk factor in the involvement of vascular disease in aging. Aging gives rise to the oxidative and nitrosative redox imbalance in plasma, and the reactive products of oxidative and nitrosative damage tend to accumulate during the aging process [Citation6–9]. Because intervention in pre-clinical conditions would have the greatest public health impact, there is a need to pay due attention to dysregulation of the redox balance of the aging plasma. Plasma redox imbalance could be related to an increased risk for endothelial damage. In order to help identify diagnostic biomarkers that can predict the gender-dependent risk of conversion to coronary artery disease, there is need to develop new biomarkers for early diagnosis of redox imbalance in plasma.
All amino acids can be attacked by ROS and RNS, but sulphur-containing and aromatic amino acids are the most susceptible to protein oxidation in aging cell. PCO groups may be formed by peptide backbone fragmentation (I); extraction of hydrogen from alpha carbon atom (II); or an oxidative attack on several amino acid side-chains (III), the formation of adducts between some amino acid residues and the products of lipid peroxidation such as MDA (IV). On the other hand, PCO groups are also generated by glycation/glyoxidation of lysine amino groups [Citation18]. Disruption of redox regulation is likely to contribute to the exponential age-related rise in the level of oxidized protein and occurrence of disease [Citation19]. Several recent studies point out the role of protein oxidation in acceleration of atherosclerosis [Citation20–22].
The presence of reactive thiol (—SH) group in proteins and amino thiols such as GSH not only predisposes these molecules to oxidative modification but also confers potent antioxidant properties. The assessment of T-SH groups could both provide an estimate of the antioxidant potential of the plasma and could also provide insight into the redox-status. We propose that decreased T-SH levels which we have observed in our male rats may be an inefficient homeostatic mechanism trying to challenge increased oxidative stress due to increased plasma MDA and 8-OHdG levels. A clear positive correlation was observed between the MDA and 8-OHdG concentrations in male rats. The positive correlation between plasma MDA and 8-OHdG levels is the indicator of a close relation between lipid peroxidation and DNA oxidation in male rats. There is increasing evidence that human atherosclerosis is associated with damage to the DNA of both circulating cells and the cells of the vessel wall [Citation23]. Indeed, various animal models of atherosclerosis support the evidence that damaged somatic DNA plays a key role in both the formation and the complications of atherosclerosis [Citation24,Citation25]. We found a strong negative correlation between oxidative DNA damage and T-SH levels in plasma of male rats. These gender-dependent differences will, we hope, lead to a new insight into the pathogenesis of atherosclerosis in aging males.
We are of the conviction that the increased T-SH groups that we have determined in aged female rats may be a limiting factor in propagation of protein oxidation, as the PCO and AOPP levels in their plasma were unchanged. There are no established data or explanation in the current literature why the plasma T-SH levels cannot be equivalently balanced in both genders. Spearman's rank correlation coefficients among the various oxidative stress parameters except the MDA/T-SH couple were not significantly correlated in female rats. At the same time, high level of negative MDA–T-SH correlation (r = −0.82) demonstrates the activation of the antioxidant potential of the plasma T-SH in females. In the current study, it is important to note that the gender-dependent changes in correlation of the oxidative stress parameters indicate that different regulatory mechanisms might play an important role in gender dependency.
GSH inhibits free radical mediated injury by eliminating ROS and protects T-SH groups from oxidation by serving as a biological redox agent. Erythrocytes play an important role in the delivery GSH to tissues with high rate of GSH utilization, including lung, heart, gut, and brain [Citation26]. In this study, erythrocyte GSH levels were not found to be different between genders. We suggest that unchanged GSH levels found in our study may point to the important strict maintenance of the erythrocyte GSH levels for the redox homeostasis T-SH levels in aforementioned tissues. It has been previously shown that Cu-Zn SOD and CAT activities in plasma of human subjects are regulated by aging process [Citation27]. Increased activities of Cu-Zn SOD would be expected to lessen susceptibility of aging plasma to oxidative injury in male rats. On the other hand, there are no established data or explanations in the current literature why the plasma CAT activities of both group of aged rats did not differ.
In conclusion, our data analysis above seems to suggest strongly that the major part of the plasma oxidative stress parameters was significantly different between male and female rats. The observed differences could be attributed to the aging processes, gender-related hormonal status, and altered homeostatic mechanisms. The wide variations found between values of aged male and female rats should be mostly referred to the role that gender-related factors play in protecting aged rats against the atherosclerosis. Our findings support the conviction that plasma redox status of aged rats has a controlling role in differing regulatory mechanisms through gender differences. The gender-related data on these oxidative stress parameters in aged rats will be useful in studies of aging-related disorders and therapeutic interventions using this model.
Acknowledgements
This work was supported in part by funding from a grant from the Research Fund of The University of Istanbul (UDP-4041/2009). The corresponding author is grateful to linguistic expert Mr. Erhan Tecimen for reading the manuscript and for improvements in the linguistic style.
References
- Halliwell B, Gutteridge JMC. Free radicals in biology and medicine, 4th ed. Oxford, UK: Clarendon Press; 2007.
- Valko M, Leibfritz D, Moncol J, Cronin MT, MazurM, Telser J. Free radicals and antioxidants in normal physiological functions and human disease. Int J Biochem Cell Biol 2007;1:44–84.
- Balaban RS, Nemoto S, Finkel T. Mitochondria, oxidants, and aging. Cell 2005;4:483–495.
- Malorni W, Straface E, Matarrese P, Ascione B, Coinu R, Canu S, Galluzzo P, Marino M, Franconi F. Redox state and gender differences in vascular smooth muscle cells. FEBS Lett 2008;582:635–642.
- Walsh T, Donnelly T, Lyons D. Impaired endothelial nitric oxide bioavailability: a common link between aging, hypertension, atherogenesis? J Am Geriatr Soc 2009;57:140–145.
- Kayali R, Çakatay U, Tekeli F. Male rats exhibit higher oxidative protein damage than females of the same chronological age. Mech Ageing Dev 2007;128:365–369.
- Çakatay U, Kayali R, Uzun H. Relation of plasma protein oxidation parameters and paraoxonase activity in the ageing population. Clin Exp Med 2008;8:51–57.
- Topçuoglu A, Uzun H, Balci H, Karakus M, Coban I, Altug T, Aydin S, Topçuoglu D, Çakatay U. Effects of estrogens on oxidative protein damage in plasma and tissues in ovariectomised rats. Clin Invest Med 2009;32:E133–E143.
- Aydin S, Uzun H, Sozer V, Altug T. Effects of atorvastatin therapy on protein oxidation and oxidative DNA damage in hypercholesterolemic rabbits. Pharmacol Res 2009;59:242–247.
- Humphreys V, Martin RM, Ratcliffe B, Duthie S, Wood S, Gunnell D, Collins AR. Age-related increases in DNA repair and antioxidant protection: a comparison of the Boyd Orr cohort of elderly subjects with a younger population sample. Age Ageing 2007;36:521–526.
- Reznick AZ, Packer L. Oxidative damage to proteins: spectrophotometric method for carbonyl assay. Methods Enzymol 1994;233:357–363.
- Hu ML. Measurement of protein thiol groups and glutathione in plasma. Methods Enzymol 1994;233:381–385.
- Witko V, Nguyen AT, Descamps-Latscha B. Microtiter plate assay for phagocyte derived taurine-chloramines. J Clin Lab Anal 1992;6:47–53.
- Nourooz-Zadeh J. Ferrous ion oxidation in presence xylenol orange for detection of lipid hydroperoxides in plasma. Methods Enzymol 1999;300:58–62.
- Buege JA, Aust SD. Microsomal lipid peroxidation. Methods Enzymol 1978;52:302–310.
- Sun Y, Oberly LW, Ying LA. Simple method for clinical assay of superoxide dismutase. Clin Chem 1988;34:497–500.
- Yasmineh WG, Kaur TP, Blazar BR, Theologides A. Serum catalase as marker of graft-versus-host disease in allogenic bone marrow transplant recipients. Clin Chem 1995;41:1574–1580.
- Stadtman ER, Levine RL. Chemical modification of proteins by reactive species. In: Dalle-Donne I, Scaloni A, Butterfield DA, editors. Redox proteomics. New Jersey: Wiley-Interscience; 2006; Chapter 1: 3–23.
- Humphries KM, Szweda PA, Szweda LI. Aging: a shift from redox regulation to oxidative damage. Free Radic Res 2006;40:1239–1243.
- Kaneda H, Taguchi J, Ogasawara K, Aizawa T, Ohno M. Increased level of advanced oxidation protein products in patients with coronary artery disease. Atherosclerosis 2002;162:221–225.
- Woods AA, Linton SM, Davies MJ. Detection of HOCl-mediated protein oxidation products in the extracellular matrix of human atherosclerotic plaques. Biochem J 2003;370:729–735.
- Lugman S, Rizvi SI. Protection of lipid peroxidation and carbonyl formation in proteins by capsaicin in human erythrocytes subjected to oxidative stress. Phytother Res 2006;20:303–306.
- Andreassi MG. Oxidative stress, DNA damage and vascular endothelial function. Biomed Pharmacother 2006;60:480–487.
- Kim KS, Kang KW, Seu YB, Baek SH, Kim JR. Interferon-γ induces cellular senescence through p53-dependent DNA damage signaling in human endothelial cells. Mech Ageing Dev 2009;130:179–188.
- Oesuburg H, Iusuf D, van der Harst P, van Gilst WH, Henning RH, Roks AJ. Bradykinin protects against oxidative stress-induced endothelial cell senescence. Hypertension 2009;53:417–422.
- Niwa T. Proteome analysis of oxidative stress: Glutathionyl hemoglobin in diabetic and uremic patients. In: Dalle-Donne I, Scaloni A, Butterfield DA, editors. Redox proteomics. New Jersey: Wiley-Interscience; 2006. Chapter 1: 651–667.
- Rizvi SI, Maurya PK. Alterations in antioxidant enzymes during aging in humans. Mol Biotechnol 2007;37:58–61.