Abstract
Rat parathyroid hormone (PTH) 1-34 (4 μg/kg/day) was applied for 2.5 months to 9 month-old rats immediately after ovariectomy or orchidectomy or to 15 month-old rats with low bone mass which had been castrated 6 months before in order to know the effects on serum biochemistry parameters, lumbar and femoral bone mineral density, histology, cancellous and cortical bone histomorphometry, mineralisation content profile in cortical bone by backscattered-electron microscopy, and femoral torsion biomechanical testing. In ovariectomised rats, preventive PTH treatment avoided cancellous bone loss in tibial metaphysis and partially in lumbar vertebra, while in cortical bone, PTH increased endosteal resorption and periosteal formation. In intervention study, PTH did not restore cancellous bone but a strong endosteal and periosteal new bone formation was detected. In orchidectomised rats, PTH, in preventive study, avoided cancellous bone loss in metaphysis and lumbar vertebra, and a mild new bone formation in cortical bone was found. In intervention study, PTH maintained baseline cancellous bone mass, but in cortical bone a strong endosteal and periosteal new bone formation was detected. The PTH-induced new bone formation was confirmed by histology and by mineral content profiles. After castration, biomechanical properties were affected in females but not in male rats and PTH reverted this effect.
Introduction
The fall in oestrogen levels after menopause leads to a decrease in bone mass as well as an increase in fracture risk. Likewise, androgen withdrawal also leads to a decrease in bone mass [Citation1,Citation2]. The analysis of results after clinical use of several kinds of osteoporosis-treatment drugs showed that a greater increase in bone mass was not always related to a decrease in fracture risk [Citation3].
Recently, the definition of osteoporosis was reviewed and changed, introducing bone quality concept and bone mass concept together [Citation4]. It is currently accepted that increase in bone fragility, i.e., fracture risk, is closely associated with bone quality [Citation5]. Bone quality includes several parameters as bone turnover, bone mass, size and geometry, bone microarchitecture, mineralisation and organic matrix composition as well as microfracture reparation capacity [Citation6]. Given the critical role of these factors for bone deterioration, it is essential to understand how they are influenced by therapeutic agents.
Drugs used for prevention and treatment of both post-menopausal and androgen-withdrawal osteoporosis can be classified in three main groups: resorption inhibitors like calcitonin [Citation7]; raloxifene [Citation8] and biphosphonates [Citation9–11], bone formation stimulators like parathyroid hormone [Citation12]; and those supposed to produce both effects simultaneously, like strontium ranelate [Citation13]. All these treatment drugs produce significant decreases in fracture risk. However, more studies addressing the effects of these therapies on bone quality are required. A recent work by Kleerekoper [Citation14] reviewed the currently-known aspects related to how osteoporosis therapies impact parameters of bone strength and quality.
Parathyroid hormone (PTH) is an anabolic agent when it is administered intermittently for osteoporosis [Citation15]. PTH exerts its actions directly on osteoblasts implementing its activity on bone mineralising surfaces [Citation16,Citation17]. The 34-amino-acid fragment PTH (1-34) has shown to increase bone mass in humans as well as reducing the risk of fracture and is now available for treatment of patients with established osteoporosis and high risk of fracture [Citation18]. However, it is not fully known how this treatment affects some parameters involved in bone quality.
The aims of this work were: (1) To study comparatively the effects produced by oestrogen or androgen depletion in some bone quality parameters such as bone mass measured by double energy X-ray densitometry (DEXA); bone microarchitecture quantified by histomorphomety; bone mineral distribution by backscattering electron microscopy and biomechanical properties of bone through torsion tests. Bone remodelling variations due to oestrogen/androgen lack were also analysed by biochemical markers of bone resorption and formation given that bone turnover is directly involved in bone tissue mineralisation and in microfracture repair [Citation19,Citation20]; (2) To study the effects of intermittent (1-34) rat PTH-treatment on these same parameters both in female and in male castrated rats at preventive and treatment level. This study has been performed using widely validated experimental models, viz., ovariectomised and orchidectomised rats [Citation21,Citation22].
Methods
Animals
Ninety-two female (F) and 102 male (M) Wistar rats, 9 months old, weighing 354 ± 66 g and 523 ± 74 g (mean ± SD), respectively, at the start of the study, were used. At this age, rats were sexually mature and fully grown animals were then ovariectomised, orchidectomised or sham-operated (SHAM) under Ketamine (40 mg/kg, Ketolar, Bayer) and Xilacine (8 mg/kg, Rompún, Parke-Davis, Pfizer), and distributed into two studies. The animals were kept under constant living conditions (22°C, 12 h per day light–dark cycles), and food (standard Laboratory chow) and water were available ad libitum. Two studies were performed: prevention of ovariectomy- or orchidectomy-induced bone loss (study A), and treatment of ovariectomy- or orchidectomy-induced osteopenia (study B).
Study A (prevention): Thirty female and 40 male rats were randomised in the following groups: SHAM groups (SHAMF n = 10 and SHAMM n = 15) treated with vehicle for 2.5 months; castrated groups (OVX n = 10 and ORX n = 12) treated also with vehicle for 2.5 months; and castrated groups treated with PTH for 2.5 months (OVX + PTH n = 10 and ORX + PTH n = 13). Treatment in this study was started immediately after surgery. Rats were killed at the end of this treatment ().
Figure 1. Design of the experimental study. The effects of rat-PTH treatment, at daily doses of 4 μg/kg, were studied in female and male rats under two different conditions. In prevention experiment (study A) recently ovariectomised or orchidectomised rats were treated with PTH for 2.5months (groups OVX/ORX + PTH). These rats were sacrificed at 11 months old with their paired SHAMF/SHAMM and OVX/ORX groups. In intervention experiment (study B) rats that were ovariectomised or orchidectomised 6 months before, and were treated with PTH for 2.5 months (groups OVX2/ORX 2 + PTH). In study B, groups of 15-month-old rats SHAMF1/SHAMM 1 and OVX1/ORX1 were sacrificed as baseline groups for the final 18.5-month-old rat groups SHAMF2/SHAMM2 and OVX2/ORX2.
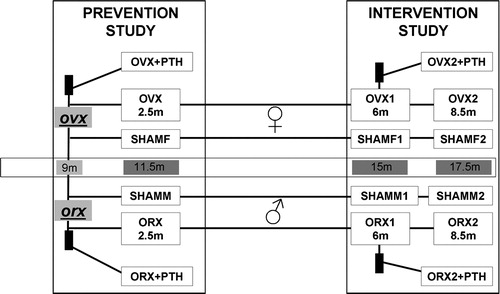
Study B (intervention): Sixty-two female and 62 male rats were randomised in the following groups: SHAM groups killed 6 months after simulated surgery (SHAMF1 n = 12 and SHAMM1 n = 11); castrated groups killed 6 months after ovariectomy or orchidectomy (OVX1 n = 12 and ORX1 n = 11); SHAM groups treated with vehicle for 2.5 months starting 6 months after simulated surgery (SHAMF2 n = 16 and SHAMM2 n = 10); castrated groups treated with vehicle for 2.5 months starting 6 months after surgery (OVX2 n = 10 and ORX2 n = 15); and, finally, castrated groups treated with PTH for 2.5 months starting 6 months after surgery (OVX2 + PTH n = 12 and ORX2 + PTH n = 15) ().
Rat PTH (1-34) was obtained as a characterised or certified drug from Sigma-Aldrich Laboratories, Germany. PTH was dissolved in albumin in acetic glacial acid solution (0.1%); it was aliquoted, lyophylised and stored at 4°C until used. The day of its use, the corresponding lyophylised aliquot was dissolved in sterile saline solution as an administration vehicle. Rats received daily subcutaneous doses of PTH at a dose of 4 μg/kg/day or vehicle, starting the day after surgery (study A), or 6 months after surgery (study B).
On the day following the last day of treatment, the experimental animals were killed by exsanguination under ether anaesthesia. Blood samples were obtained by cardiac puncture and serum samples were immediately frozen at –80°C as aliquots for measuring markers of bone turnover and biochemical parameters. Once the blood was collected, lumbar vertebra and the two tibias of some animals were extracted for bone histomorphometry studies as will be detailed below. Right femurs of these rats were also extracted for mechanical tests. The rest of the animals were frozen at –20°C until bone mineral density (BMD) determination was performed. Animals were thawed at 4°C 2 days before BMD measurement.
All procedures were carried out in accordance with European Community Standards on the Care and Use of Laboratory Animals.
Biochemical parameters
Serum creatinine levels were measured using an autoanalyser method (modular Roche DDPP). Sensitivity of this method was 0.1 mg/dl. Intra- and inter-assay coefficients of variation of the method were <0.7% and <2.3%, respectively.
Serum calcium levels were measured using an autoanalyser method (modular Roche DDPP). Sensitivity of this method was 0.2 mg/dl. Intra- and inter-assay coefficients of variation of the method were <0.9% and <1.5%, respectively.
Biochemical markers of bone turnover
Serum C-telopeptide of type I collagen (CTX) was measured by an ELISA specific for rat CTX (RatLaps ELISA, Nordic biosciences, Denmark). Sensitivity of the assay was 2.0 ng/ml. Intra- and inter-assay variation coefficients of the method were <5.6% and <10.5%, respectively.
Serum Bone Gla Protein (BGP) was measured by an immunoradiometric assay (IRMA) for the specific quantitative determination of rat osteocalcin levels (Rat Osteocalcin IRMA kit, Immutopics, San Clemente, CA). Sensitivity of this assay was 0.01 ng/ml. Intra- and inter-assay coefficients of variation of the method were <2.0% and <2.3%, respectively.
Serum alkaline phosphatase (ALP) was determined using an autoanalyser method (modular Roche DDPP). Sensitivity of this method was 1 UI. Intra- and inter-assay coefficients of variation of the method were <0.67% and <0.67%, respectively.
Bone mineral density
Bone mineral density was determined in situ in the lumbar spine (L2, L3 and L4) and in the whole left femur by DEXA using a HOLOGIC QDR-1000 TM (S/N 277) (Hologic, Inc., Waltham, MA, USA) with small-animal software [Citation23]. Previously, the whole left femur was extracted and cleaned of adjacent tissue. Intra-assay and inter-assay variation coefficients were <0.53% and <1.2%, respectively. The scans of the femur were analysed for BMD of the whole femur and the scans of the L2, L3 and L4 vertebrae were analysed for BMD of the whole three vertebrae and results were expressed as the mean of the values obtained.
Bone histology and histomorphometry
Lumbar vertebrae and tibias were isolated and fixed in neutral formol for 24h at 4°C. Lumbar vertebrae (L4) were embedded, undecalcified, in glycol-methacrylate/methyl-methacrylate (1:1). Serial sections (2.5 μm thick) were cut in the medial plane with a heavy-duty microtome (Microm HM360, Germany) by using 8-mm-length diamond knives (Diatome, Switzerland), and stained with toluidine blue or with von Kossa. In tibias, metaphyseal cancellous bone was studied using stereoscopic zoom microscopy (Nikon SMZ1500, Japan). For that, metaphysis was sawed into-500-μm thick sections by using a linear precision saw (Buehler Isomet 4000, USA) equipped with a 15LC diamond wafering blade. Sawed sections were rendered anorganic by sodium hypochlorite treatment. For tibia cortical bone studies, samples of tibia diaphysis, next to fibula junction, were embedded undecalcified in poly methyl-metacrylate. Two ground sections, 100 μm thick, were prepared by using a grinding-polishing machine (Buehler Phoenix Beta, USA) and stained with toluidine blue or with von Kossa.
Bone histomorphometry was performed in von Kossa-stained sections from vertebra and tibia diaphysis, and from anorganic tibia metaphysis sections. All sections were digitalised by using an optical scanner (HP scanjet 8200, USA) at 9600pp resolution (pixel size = 2.6 μm) [Citation24]. Digital images were converted in a 1-bit black/white image after thresholding with the Image J (NIH, USA) programme (). This image was used first to calculate bone areas and bone perimeters by pixel counting. Then structural indices such as cancellous bone volume BA/TA (%), trabecular thickness Tb.Th (μm), separation Tb.Sp (μm), and number Tb.N (n°/mm) for lumbar vertebra; apparent bone trabecular area (mm2) for tibia metaphysis, and cortical bone tissue area (mm2), medullar bone area (mm2) and cortical thickness (μm) for tibia diaphysis were determined in all groups.
Bone mineral content profile
Bone mineral content profile was determined only in study B where changes in total bone mineralisation degree were expected to occur after a long period of oestrogen or androgen depletion. Femurs from female rats and tibias from male rats were embedded in poly methyl-methacrylate. Single blocks were sawed transversally, and further trimmed and re-embedded together in such a way that blocks containing femurs (four femurs each group) or tibias (four tibias each group) were done. The blocks were cut plane parallel with a linear precision saw (Buehler Isomet 4000, USA) and the block surface containing bone was then diamond-polished. Carbon-coated blocks were observed under a scanning electron microscope (FEI Quanta 200, The Netherlands) fitted with a solid-state backscattering detector. The microscope was operated under high pressure at 20 kV, 35 μA probe current and a working distance adjusted to 10.1 mm. All femurs were scanned (50× magnification) in the same session to have equal contrast and brightness detection. Tibias were scanned at 60× magnification. Mineral profiles were measured as mean grey levels, grouped in 16 bins, and results expressed as percent cortical mineralised bone in each bin/total cortical mineralised bone [Citation25]. Grey-level images were also processed by the Image J (NIH, USA) to display colour-coded mineralisation maps [Citation26].
Biomechanical testing
The right femur of the animals was cleaned of adjacent tissue and the biomechanical test was performed. The mechanical test involved low-rate torsion (10°/min). A Schenk-Trebel RTP 0.6 model electromechanical testing machine was used with an M1602 digital indicator (Schenk-Trebel, Germany). A high-definition sensor was fitted to the machine in order to measure torque in a nominal range of <2 Nm. A torque-angle turned curve was obtained for each femur. From this curve, the following information was obtained in each test: maximum value of the torque until fracture; angular deformation for the value of the maximum torque; stiffness of the sample calculated as the slope of the torque-angle turned curve measured by interpolation between points corresponding to 10–50% of the angular deformation of maximum torque (elastic zone), and absorbed energy until fracture.
Statistical analyses
The results of the experiments were expressed as the mean ± SD of the different parameters. A non-parametric method, Mann–Whitney test (Medcalc Software Program, Belgium), was used to compare the different treatment groups. A p value <0.05 was accepted as denoting a significant difference.
Results
Biochemical parameters
Parathyroid hormone treatment did not modify creatinine levels in any studied group. Creatinine values were maintained between 0.44 mg/dl and 0.58 mg/dl. Calcemia was maintained in normal range (between 10.09 and 10.78 mg/dl) in all groups throughout the study.
Bone remodelling biochemical markers
Females
shows that a significant increase was produced in bone remodelling at resorption level (CTX) and at formation level (BGP, ALP) 2.5 months after ovariectomy (OVX group).
This increase in bone remodelling was maintained in rats with an established low bone mass 6 months after ovariectomy; 8.5 months after ovariectomy only BGP and ALP maintained their increase, while CTX did not.
PTH treatment administered immediately after ovariectomy (OVX + PTH) maintained increased levels of bone formation markers (BGP and ALP). These levels were significantly higher than those of the SHAM group (SHAMF) and not significantly higher than those of the OVX group (OVX). CTX levels stayed increased in ovariectomised rats in preventive treatment (OVX + PTH), being even higher than those of the OVX group without any treatment (OVX). However, PTH did not modify CTX values when rats with an induced osteopenia were treated (OVX2 + PTH). BGP and ALP levels in this group (OVX2 + PTH) were increased with respect to the SHAMF2 group.
Table I. Biochemical markers of bone turnover.
Males
shows a significant increase in bone remodelling both in bone resorption (CTX) and in bone formation (BGP, ALP), 2.5 months after orchidectomy (ORX). This increase, however, was only maintained for BGP after 6 (ORX1) and 8.5 months (ORX2) from surgery. CTX levels were similar in ORX1 (6 months after surgery) and ORX2 (8.5 months after surgery) to SHAMM1 and SHAMM2 groups, respectively. ALP levels of ORX1 were not different from those of SHAMM1, 6 months after surgery. However, ALP in ORX2 group was significantly decreased with respect to their control group (SHAMM2).
Parathyroid hormone treatment immediately after orchidectomy for 2.5 months (ORX + PTH) maintained the significant increase in bone remodelling (BGP, ALP, CTX) with respect to control rats (SHAMM) and with a tendency, although without significance, to be even higher than that of orchidectomised animals without treatment (ORX).
Parathyroid hormone treatment to rats orchidectomised 6 months previously (ORX2 + PTH) did not increase bone resorption (CTX) level with respect to ORX2 or SHAMM2 groups. BGP was elevated with respect to SHAMM2, but levels were similar to their paired ORX2 rats. On the contrary, ALP levels were similar to those of the ORX2 and lower than those of SHAMM2 groups.
Bone mineral density
Females
shows femoral BMD (FBMD) and lumbar BMD (LBMD) measured by DEXA in rats of the prevention and treatment groups.
No significant differences in femoral or lumbar BMD were found between OVX and SHAM groups 2.5 months after ovariectomy (OVX group). However, these parameters were significantly decreased in rats that were sacrificed 6 months after ovariectomy (OVX1) and 8.5 months after ovariectomy (OVX2) with respect to their age-matched SHAM group (SHAMF1 and SHAMF2).
Table II. Bone mineral density (BMD) (g/cm2) measured in femur (FBMD) and in lumbar vertebra (LBMD).
Parathyroid hormone treatment for 2.5 months starting immediately after ovariectomy (OVX + PTH) produced a significant increase in FBMD and LBMD with respect to the SHAM group (SHAMF). Administration of PTH to rats with established osteopenia 6 months after ovariectomy restored the levels of LBMD of OVX rats but could not restore the normal levels of FBMD.
Males
No differences in femoral or lumbar BMD were found between ORX and SHAMM groups 2.5 months after orchidectomy (). However, FBMD was significantly decreased in rats that were sacrificed 6 months after orchidectomy but LBMD was not. After 8.5 months of orchidectomy, both FBMD and LBMD were significantly decreased.
PTH treatment for 2.5 months starting immediately after orchidectomy (ORX + PTH) produced a significant increase in FBMD and LBMD with respect to the SHAMM group. Administration of PTH to rats with established osteopenia 6 months after orchidectomy (ORX2 + PTH) restored BMD levels at femoral and lumbar level.
Bone histology and histomorphometry
Females
shows bone histomorphometry measurements from lumbar vertebra, tibial metaphysis and diaphysis. OVX rats had a decrease in bone mass with BA/TA rates lower than their paired SHAMF. Trabecular bone loss was more severe in tibia metaphysis than in lumbar vertebra, and these changes were depicted in . In OVX1 and OVX2 rats an osteopenia was established, but there were no differences among them. In metaphysis, bone loss occurred chiefly in central zone and trabecular elements, as seen directly by stereoscopic microscopy (). In OVX rats bone loss also affected cortical bone, higher values being apparent for medullar area and decreased cortical thickness in all groups.
Table III. Bone histomorphometry of lumbar vertebra (L4) and tibia.
Figure 3. Tibial metaphysis from female rats. Representative anorganic sections from female rats in prevention (a–c) and intervention experiments (d–h). Note that in OVX groups (b,d,e) there is trabecular bone loss in the central zone. Detail of the trabecular microarchitecture (i–m) as viewed by stereoscopic zoom microscopy. Note in OVX2 (l) the extreme trabecular thinning.
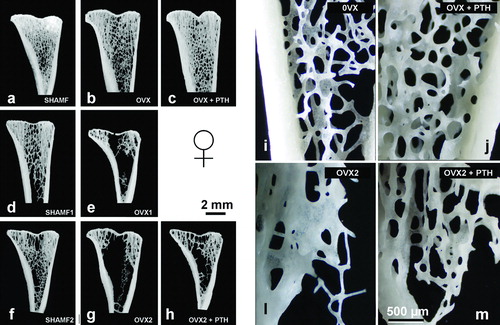
In the preventive experiment (study A), PTH effects were noted in vertebral trabecular bone and metaphysis with values that were higher than OVX group and similar to SHAMF group. Nevertheless, in cortical bone PTH had a dual effect increasing resorption at the endosteal surface (higher medullar area values) and new bone formation at the periosteal side, though the net effect was a thicker cortical. These histological changes, i.e., endosteal resorption and periosteal bone formation were assessed in toluidine-blue-stained sections (). In the intervention experiment (study B), PTH treatment did not produce observable effects on vertebral trabecular bone and tibial metaphysis when compared with OVX2 group. On the contrary, PTH effects on cortical bone were noted as lower medullar area and higher cortical thickness. confirmed histologically that new bone was deposited in both endosteal and periosteal surfaces.
Figure 4. Tibia cortical bone from female rats. Periosteal and endosteal surfaces as view in 100 μm thick ground sections stained with toluidine blue from prevention (a–f) and intervention experiments (g–l). Note that in prevention study PTH-treatment has a dual effect as new bone formation at periosteal envelope (c) and resorption at endosteal surface (f). On the contrary, in intervention experiment, PTH-treatment produces new bone both in periosteal (i) and endosteal (l) surfaces.
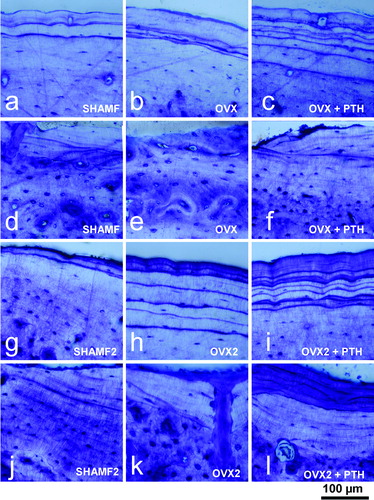
Males
shows bone histomorphometry measurements for male groups. In ORX rats, there was a trabecular-bone decrease in lumbar vertebra and in metaphysis. Bone loss was even continued in ORX2 group (). Beside this bone loss, another histological trait was significant in ORX rats, viz., the existence of wide osteoid seams (), indicating that following androgen depletion, there was an impaired mineralisation. Nevertheless, cortical bone responded differently, bone cortical loss was only noticed in ORX2 (higher medullar area and lower cortical thickness). Wider osteoid seams in cortical bone were also detected ().
Figure 5. Tibia metaphysis from male rats. Representative anorganic sections from male rats in prevention (a–c) and intervention experiments (d–h). Note that in ORX groups (b,d,e) there is a progressive bone loss with time, and that in ORX2 group only lateral trabeculas remained. Detail of the microarchitecture of the metaphysis (i–m) as viewed by stereoscopic zoom microscopy. Note that the trabecular thinning in ORX2 (l), and in ORX2 + PTH (m) have a similar thickness to baseline ORX1 (h).
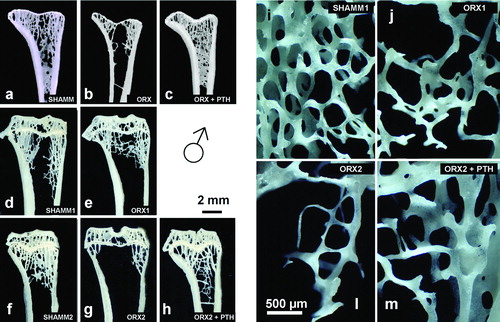
Figure 6. Lumbar vertebra from male rats. Early bone formation surfaces with cuboidal osteoblasts and osteoid seams (a,c,e) and late bone formation surfaces with elongated osteoblasts and osteoid seams (b,d,f) as shown in 2.5 μm sections stained with toluidine blue. Note that ORX group has wide osteoid seams throughout, but PTH treatment reverses impaired mineralisation.
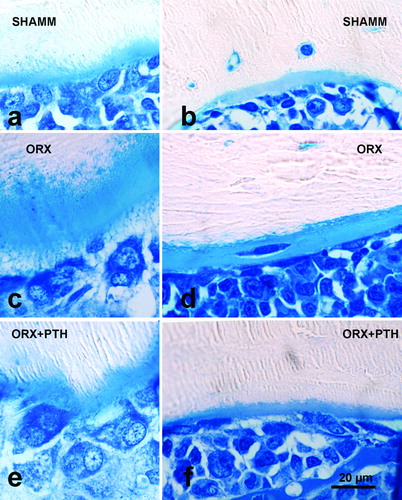
Figure 7. Tibia cortical bone from male rats. Endosteal surfaces in male rats as viewed in 100 μm-thick ground sections stained with toluidine blue from preventive experiment (a–c) and from intervention experiment (d–f). Note that ORX1 cortical bone has a wide osteoid seam in an intracortical (remodelled) osteon (e), and that PTH treatment produces new bone formation both in prevention (c) and intervention experiments (f).
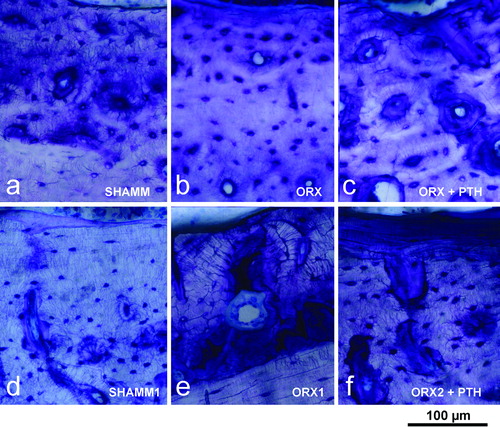
In study A, ORX + PTH bone mass values were similar to SHAM and higher than ORX group. Particularly, Tb.Th values were higher, a circumstance explained by the fact that PTH notably improved mineralisation of the osteoid (). In cortical bone a significant reduction in medullar area was seen. It depended on new bone formation at the endosteal envelope that was confirmed by histology (). In study B, bone trabecular rates were maintained at similar level to ORX1, both in lumbar vertebra and in metaphysis. In cortical bone, PTH new bone formation both at endosteal and periosteal surfaces was confirmed by histology (). Consequently cortical bone had higher values for bone cortical area and cortical thickness, and lower values for medullar area.
Bone mineralisation degree
Females
In study B, baseline OVX1 group showed lower mineralisation degree than their paired SHAMF1. shows mineral content profile for females at the end of experiment, OVX2 and OVX2 + PTH showed a decrease in mineral content profile (curves shifted to the left). With PTH treatment, curves are even more shifted, but in this case, it was the result of new bone formation (that is always less mineralised than old bone).
Males
ORX1 showed lower mineralisation degree than SHAMM1. The same trends were seen in ORX2 and ORX2 + PTH with curves shifted to left (). In PTH treated rats, colour-coded mineralisation maps showed new bone formation (blue colour) both at endosteal and periosteal surfaces that was confirmed at higher magnifications ().
Figure 9. Colour-coded mineralisation maps from SHAMM2 (a), ORX2 (b), and ORX2 + PTH (c) obtained by BSI at ×60 magnification (pixel size = 5.6 μm). In the scale, blue colour indicates less mineralised bone, i.e., sites of new bone formation. Detail of new formation at endosteal (d) and periosteal (e) surfaces viewed at ×1000 magnification.
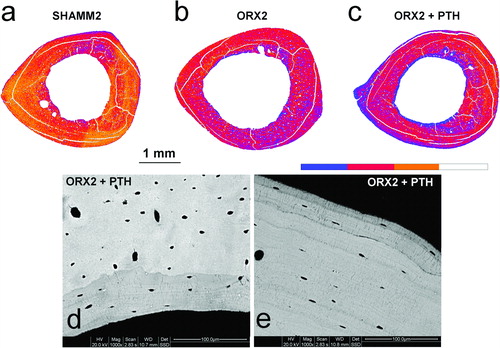
Biomechanical testing
Females
The results obtained when torsion assays were performed show () that ovariectomy produced a significant decrease in maximum torque in rats 2.5 months (OVX), 6 months (OVX1) and 8.5 months (OVX2) after ovariectomy. Six months (OVX1) and 8.5 months after ovariectomy (OVX2), maximum angle of torsion necessary to produce bone fracture, was significantly decreased with respect to the respective SHAMF groups. However, 2.5 months after ovariectomy we did not find a significant decrease of this parameter. Absorbed energy was also lower than that of the SHAMF group in all ovariectomised rat groups.
Table IV. Bone biomechanics measured in femurs.
Parathyroid hormone treatment administered immediately after ovariectomy (OVX + PTH) avoided the decrease in maximum torque and absorbed energy. However, in our experimental conditions, PTH treatment for 2.5 months could not reverse the effects produced in maximum torque or absorbed energy in rats with established low bone mass (OVX2 + PTH) (). We found no differences in stiffness among all the studied groups.
Males
We found no significant difference in biomechanical parameters among orchidectomised, PTH-treated and their respective SHAMM groups ().
Discussion
Previous studies, in which ovariectomised or orchidectomised rats were used as experimental models, employed rats younger than in the present study [Citation27–30]. These works used rats between 3 and 6 months old, with sexual maturity but that were still growing. However, there are few studies in which old rats were used [Citation31,Citation32] and in the present work we used 9-month-old rats which had finished their growing stage. In these conditions, experiments were more similar to the metabolic situation of humans who suffer osteoporosis for post-menopausal or androgenic-depletion reasons.
In previous works, the dosages of PTH administered to the rats were high (40, 80 μg/kg/day) [Citation30,Citation33] with respect to those used in the clinical practice, that usually are no higher than 2 μg/kg/day [Citation34–37]. In our experimental design we used 4 μg/kg/day of PTH, a dosage closer to that used in humans. In a previous work, Turner et al. [Citation38] demonstrated that high doses rates (80 μg/kg/day) of the hormone result in excessive stimulation of bone formation and an increase in trabecular thickness. At this point, it is important to emphasise that the dose of PTH employed in this work did not produce alterations in serum creatinine in any animal. In the same way, hypercalcemia was not produced in any case. Some authors observed in clinical studies that hypercalcemia after PTH treatment is dose-dependent [Citation39,Citation40]. It is also interesting to mention the fact that in this work we used rat PTH (1-34) instead of human PTH (1-34).
As expected, ovariectomy produced a high bone turnover, which slows down after the initial 6 months [Citation25]. Initially, bone remodelling is increased, both at formation and resorption level. This fact has been described both in human and experimental animal model studies [Citation19,Citation41,Citation42]. It is known that oestrogen lack produces a disorder in bone remodelling that negatively affects bone integrity [Citation43]. Although an increase in bone resorption is positive with respect to bone microfracture repair, the increase in bone resorption over bone formation exerts a bone loss net effect.
In the present work, bone loss 2.5 months after ovariectomy was not detectable by DEXA technique, but it was by means of histomorphometry. Previous studies demonstrated that trabecular area and BA/TA determinations were more sensitive than BMD [Citation22]. However, induced osteopenia was severe 6 and 8.5 months after ovariectomy, and thus it was detectable both by DEXA and histomorphometry.
In our study, in untreated OVX groups, cancellous bone is lost in metaphysis and in lumbar vertebra, reaching a plateau 6 months after ovariectomy when bone turnover has reduced. In the same way, bone loss was detected in cortical bone due to resorption at endosteal envelopes. In OVX rats, bone mineralisation degree was also lower, as it was reported previously [Citation25]. In fact, lower mineral profiles are expected in bone with high turnover [Citation20] as it is the case of ovariectomy. Our results showed that bone biomechanical properties of OVX rats were affected. In this work, we only studied biomechanical properties performing torsion assays. Other authors [Citation29] found similar results after ovariectomy with bending studies.
Parathyroid hormone treatment for 2.5 months immediately after ovariectomy avoided bone loss in tibial metaphysis and partially in lumbar vertebra. Our results agree with those of Sato et al. [Citation44] who administered 8 and 40 μg/kg/day of PTH to ovariectomised, 9-month-old Sprague-Dawley rats, for 6 months, immediately after surgery.
However, PTH effects in cortical bone were different. Increased resorption at the endosteal surfaces (higher medullar area) and increased formation at periosteal surfaces (higher bone tissue area and cortical thickness) were seen at the same time. Nevertheless, tibia as a whole had net bone gain, a fact also reflected in biomechanical test since OVX + PTH returned to normal maximum torque values.
A different pattern is found when PTH treatment is given to rats 6 months after ovariectomy with induced osteopenia. In this case, PTH was not able to significantly reverse the negative effects produced by ovariectomy on BMD, BA/TA or trabecular area. In a previous work by Stewart et al. [Citation29] PTH treatment (40 μg/kg/day) reversed the loss in trabecular area in rats ovariectomised at 5 months old and treated with PTH from 1 month to 7 months later. Moreover, after treatment, trabecular area of rats was higher than that of the SHAM group. In another work, Tsuchida et al. [Citation45] administered PTH (6 μg/kg/day) and got increased trabecular connectivity in ovariectomised 7-month-old rats, without getting SHAM levels.
The differences between these results and those found by us in 18.5-old-month rats could be due to the different dose of PTH, treatment instauration time and duration as well as the age of rats used. In Stewart's report dose was 10 times higher than ours, and treatment lasted 6 months. In Tsuchida's report, dose is similar but treatment duration was 4 months. The PTH dose seems to be an important factor. Another important factor in PTH treatment could be the moment in which treatment is begun. In Stewart's report [Citation29] PTH treatment was initiated only 1 month after ovariectomy, but in the present work, treatment was initiated 6 months after surgery. In this respect, Tsuchida et al. [Citation45] found that the recuperation of trabecular connectivity in ovariectomised rats with PTH treatment was better if this was started 1 month after ovariectomy rather than 3 months after surgery. These findings led these authors to suggest that there is a limit to the loss of trabecular connectivity after which it is not possible to recover bone mass with PTH treatment. On the other hand, it is important to take into account that PTH induces strong bone modelling in young rats while in mature rats remodelling becomes more important [Citation46]. At this respect, Frieldl et al. [Citation47] studied the effects of PTH on rats with different ages, and concluded that while in fast-growing rats, PTH increased osteoblast activity, which resulted in retention of new trabeculae, in aged rats, in which quiescent bone surfaces covered with lining cells are increased, PTH is capable to increase osteoblast number, leading to a larger relative increase in surface referent bone formation rate than in younger rats.
In the intervention study, in spite of cancellous bone mass not being changed by PTH treatment in OVX rats, a significant increase in tibia cortical bone tissue area and cortical thickness were detected. In this situation, cortical bone responded with new bone formation at endosteal and periosteal envelopes, a fact also evidenced in our study of bone mineral profile. Although total mineralisation degree was even lower than in ovariectomised rats, the curve was broader indicating that new bone, always less mineralised [Citation25], had appeared. However, maximum torque and absorbed energy were not improved by new cortical bone formation.
On the whole, our results with OVX rats suggest that the dose of PTH used is adequate to prevent partially the loss of bone quality after ovariectomy, but this dose is not capable of fully recovering bone when it is severely affected by oestrogen lack. With respect to male rats, 2.5 months after orchidectomy an increase in bone remodelling was observed both at resorption (CTX) and formation (BGP and ALP) levels as previously reported [Citation22]. Six and 8.5 months after surgery, CTX levels declined but levels of BGP were maintained. Interestingly, ALP was reduced, perhaps as a consequence of the impaired mineralisation of osteoid seams as was observed by histology.
Initial high bone turnover after orchidectomy induced a rapid cancellous bone loss. In metaphysis cancellous bone loss continued, and at 8.5 months after orchidectomy only lateral trabecular elements survived. However, cortical bone loss proceeded slowly at endocortical surface, so cortical bone thinning was significant only 8.5 months after orchidectomy.
In a similar way to female rats, the decrease in BMD measured by DEXA, both in femur and vertebra, was not detected 2.5 months after orchidectomy, although it was detectable after 6 or 8.5 months. In the case of orchidectomy, bone loss (chiefly cancellous bone) after surgery did not produce variations in the analysed biomechanical properties, in spite of bone mineral content profile being lower. It is difficult to conclude whether differences between orchidectomy and ovariectomy biomechanics are due to dynamics of the bone remodelling or to other intrinsic factors associated to sex, bone size, geometry, cortical thickness, mineralisation or matrix composition. It is a fact that bone mass, bone size and cortical thickness in males are higher than in females determined in basal conditions and at the same age.
Parathyroid hormone treatment to orchidectomised male rats for 2.5 months, immediately after surgery, did not produce significant changes on bone remodelling with respect to the untreated orchidectomised group. However, in this case, in a similar way to the study with female rats, there is a positive balance of formation over resorption, showing that ORX rats treated with PTH had higher levels of femoral and lumbar BMD than the intact group. The preventive PTH treatment restored tibia metaphysis and lumbar vertebra trabecular bone values similar to its paired SHAM. Thickening of trabeculas was notable, and it is due not only to increased formation but to normal mineralisation of the osteoid. The preventive treatment did not affect biomechanical bone parameters, suggesting that PTH does not produce negative effects on bone fragility.
In the case of PTH treatment applied to orchidectomised male rats with an established osteopenia 6 months after surgery, there were no significant observable variations in biochemical markers of bone turnover with respect to untreated rats. PTH treatment recovered partially control baseline bone cancellous mass in tibia metaphysis and lumbar vertebras. In this context, Gabet et al. [Citation30], using microtomography, found a significant, although incomplete, restoration in trabecular number in 13-week-old orchidectomised rats treated with 80 μg/kg/day from 6 to 12 weeks after surgery. In another study, Oxlund et al. [Citation48] found in 24-month-old male rats, with a deep osteopenia due to their age, that treatment with 62.5 μg/kg/day for 56 days produced a significant increase in BA/TA.
Parathyroid hormone also restored tibia cortical bone thickness due to new bone formation at endosteal and periosteal surfaces, and in consequence mineralisation degree is lower than in the orchidectomised. In the same way as in females, curves are broader indicating a recent mineralisation [Citation20], but these changes in mineralisation did not produce significant variations in the biomechanical parameters studied.
Our results with ORX rats suggest that the dose of PTH used in this work is adequate both to prevent the development of osteopenia after orchidectomy and to reverse the effects produced on bone by androgen lack, although incompletely, because BA/TA and trabecular number are not quite restored.
In conclusion, the results of the present work agree with previous works in the fact that both ovariectomyand orchidectomy produced significant alterations in bone quality, remodelling, trabecular structure, mineralisation distribution and BMD. However, in similar conditions with respect to animal age and time of the experiment after hormonal depletion, oestrogen lack produced significant changes in biomechanical femoral properties in female rats, and androgen lack did not, a fact that indicates that oestrogenic lack produces more serious consequences in bone quality than androgenic lack. A dose of 4 μg/kg/day of PTH administered for 2.5 months to castrated rats (females or males) immediately after surgery avoids the negative effects produced by ovariectomy (this one only partially) and orchidectomy. Nevertheless, the same dose administered to osteopenic rats, beginning the treatment 6 months after castration, reverses the decrease in cortical thickness, but is not capable of fully restoring cancellous bone. These results suggest that it is possible that a limit exists in the level of bone loss and in the moment in which PTH treatment can be started, in order to get complete good results. If we attend to the results of the present work, we cannot reject that we could obtain better results with a higher dose of PTH and/or with more time of treatment.
Although it is not possible to extrapolate the results achieved in animals directly to humans, these experimental models let us study the mechanisms of bone loss produced after sexual hormone depletion, and the ability of PTH treatment to prevent or rescue these disorders.
Acknowledgements
This work was supported by grants PI021038 and PI020646 from FIS, Instituto Carlos III, Spain.
References
- Eastell R. Pathogenesis of postmenopausal osteoporosis. In: Favus MJ, editor. Metabolic bone diseases and disorders of mineral metabolism 5th ed. Washington: ASBMR; 2003. pp 314–316.
- Boonen S, Kaufman JM, Goemaere S, Bouillon R, Vanderschueren D. The diagnosis and treatment of male osteoporosis: Defining, assessing, and preventing skeletal fragility in men. Eur J Intern Med 2007;18:6–17.
- Riggs BL, Melton LJ 3rd. Bone turnover matters: the raloxifene treatment paradox of dramatic decreases in vertebral fractures without commensurate increases in bone density. J Bone Miner Res 2002;17:11–14.
- NIH Consensus Development Panel on Osteoporosis Prevention. Diagnosis and Therapy. JAMA 2001;285:785–795.
- González Macías J. Osteoporosis: definición y etiología. In: Manual práctico de osteoporosis y enfermedades del metabolismo mineral, Santander Spain: Jarpyo; 2004. pp 99–103.
- Turner CH. Biomechanics of bone: determinants of skeletal fragility and bone quality. Osteoporos Int 2002;13:97–104.
- Cranney A, Tugwell P, Zytaruk N, Robinson V, Weaver B, Shea B, Wells G, Adachi J, Waldegger L, Guyatt G; Osteoporosis Methodology Group and The Osteoporosis Research Advisory Group. Meta-analyses of therapies for postmenopausal osteoporosis. VI. Meta-analysis of calcitonin for the treatment of postmenopausal osteoporosis. Endocr Rev 2002;23:540–551.
- Cranney A, Tugwell P, Zytaruk N, Robinson V, Weaver B, Adachi J, Wells G, Shea B, Guyatt G; Osteoporosis Methodology Group and The Osteoporosis Research Advisory Group. Meta-analyses of therapies for postmenopausal osteoporosis. IV. Meta-analysis of raloxifene for the prevention and treatment of postmenopausal osteoporosis. Endocr Rev 2002;23:524–528.
- Cranney A, Guyatt G, Krolicki N, Welch V, Griffith L, Adachi JD, Shea B, Tugwell P, Wells G; Osteoporosis Research Advisory Group (ORAG). A meta-analysis of etidronate for the treatment of postmenopausal osteoporosis. Osteoporos Int 2001;12:140–151.
- Cranney A, Tugwell P, Adachi J, Weaver B, Zytaruk N, Papaioannou A, Robinson V, Shea B, Wells G, Guyatt G; Osteoporosis Methodology Group and The Osteoporosis Research Advisory Group. Meta-analyses of therapies for postmenopausal osteoporosis. III. Meta-analysis of risedronate for the treatment of postmenopausal osteoporosis. Endocr Rev 2002;23:517–523.
- Cranney A, Wells G, Willan A, Griffith L, Zytaruk N, Robinson V, Black D, Adachi J, Shea B, Tugwell P, Guyatt G; Osteoporosis Methodology Group and The Osteoporosis Research Advisory Group. Meta-analyses of therapies for postmenopausal osteoporosis. II. Meta-analysis of alendronate for the treatment of postmenopausal women. Endocr Rev 2002;23:508–516.
- Vestergaard P, Jorgensen NR, Mosekilde L, Schwarz P. Effects of parathyroid hormone alone or in combination with antiresorptive therapy on bone mineral density and fracture risk – a meta-analysis. Osteoporos Int 2007;18:45–57.
- Meunier PJ, Slosman DO, Delmas PD, Sebert JL, Brandi ML, Albanese C, Lorenc R, Pors-Nielsen S, De Vernejoul MC, Roces A, Reginster JY. Strontium ranelate: dose-dependent effects in established postmenopausal vertebral osteoporosis – a 2-year randomized placebo controlled trial. J Clin Endocrinol Metab 2002;87:2060–2066.
- Kleerekoper M. Osteoporosis prevention and therapy: preserving and building strength through bone quality. Osteoporos Int 2006;17:1707–1715.
- Tam CS, Heersche JN, Murray TM, Parsons JA. Parathyroid hormone stimulates the bone apposition rate independently of its resorptive action: differential effects of intermittent and continuous administration. Endocrinology 1982;110:506–512.
- Dempster DW, Cosman F, Kurland ES, Zhou H, Nieves J, Woelfert L, Shane E, Plavetic K, Muller R, Bilezikian J, Lindsay R. Effects of daily treatment with parathyroid hormone on bone microachitecture and turnover in patients with osteoporosis: a paired biopsy study. J Bone Miner Res 2001;16:1864–1853.
- Jilka RL. Molecular and cellular mechanisms of the anabolic effect of intermittent PTH. Bone 2007;40:1434–1446.
- Hodsman AB, Bauer DC, Dempster DW, Dian L, Hanley DA, Harris ST, Kendler DL, McClung MR, Miller PD, Olszynski WP, Orwoll E, Yuen CK. Parathyroid hormone and teriparatide for the treatment of osteoporosis: a review of the evidence and suggested guidelines for its use. Endocr Rev 2005;26:688–703.
- Eastell R, Barton I, Hannon RA, Chines A, Garnero P, Delmas PD. Relationship of early changes in bone resorption to the reduction in fracture risk with risedronate. J Bone Miner Res 2003;18:1051–1056.
- Ruffoni D, Fraztl P, Roschger P, Klaushofer K, Weinkamer R. The bone mineralization as a fingerprint of the mineralization process. Bone 2007;40, 1308–1319.
- Diaz-Curiel MD, Calero JA, Guerrero R, Gala J, Gazapo R, de la Piedra C. Effects of LY‐117018 HCl on bone remodeling and mineral density in the oophorectomized rat. The Am J Obstet Gynecol 1998;178:320–325.
- Erben RG, Eberle J, Stahr K, Goldberg M. Androgen deficiency induces high turnover osteopenia in aged male rats: a sequential histomorphometric study. J Bone Miner Res 2000;15:1085–1098.
- Gala Paniagua J, Díaz-Curiel M, de la Piedra Gordo C, Castilla Reparaz C, Torralbo García M. Bone mass assessment in rats by dual energy X-ray absorptiometry. Brit J Radiol 1998;71:754–758.
- Thomsen JS, Ebbesen EN, Mosekilde L. A new method of comprehensive static histomorphometry applied on human lumbar vertebral cancellous bone. Bone 2000;27:129–138.
- Kneissel M, Boyde A, Gasser JA. Bone tissue and its mineralization in aged estrogen-depleted rats after long-term intermittent treatment with parathyroid hormone (PTH) analog SDZ PTS 893 or human PTH (1-34). Bone 2001;28:237–250.
- Gomez S, and Boyde A. Correlated alkaline phosphatase histochemistry and quantitative backscattered electron imaging in the study of rat incisor ameloblasts and enamel mineralization. Microsc Res Tech 1994;29:29–36.
- Sogaard CH, Mosekilde L, Thomsen JS, Richards A, McOsker JE. A comparison of the effects of two anabolic agents (fluoride and PTH) on ash density and bone strength assessed in an osteopenic rat model. Bone 1997;20:439–449.
- Turner RT, Evans GL, Cavolina JM, Halloran B, Morey-Holton E. Programmed administration of parathyroid hormone increases bone formation and reduces bone loss in hindlimb-unloaded ovariectomized rats. Endocrinology 1998;139:4086–4091.
- Stewart AF, Cain RL, Burr DB, Jacob D, Turner CH, Hock JM. Six-month daily administration of parathyroid hormone and parathyroid hormone-related protein peptides to adult ovariectomized rats markedly enhances bone mass and biomechanical properties: a comparison of human parathyroid hormone 1‐34, parathyroid hormone-related protein 1‐36, and SDZ-parathyroid hormone 893. J Bone Miner Res 2000;15:1517–1525.
- Gabet Y, Kohavi D, Müler R, Chorev M, Bab I. Intermittently administered parathyroid hormone 1-34 reverses bone loss and structural impairment in orchiectomized adult rats. Osteoporos Int 2005;16:1436–1443.
- Qi H, Li M, Wronski TJ. A comparison of the anabolic effects of parathyroid hormone at skeletal sites with moderate and severe osteopenia in aged ovariectomized rats. J Bone Miner Res 1995;10:948–955.
- Banu J, Kalu DN. Effects of cerivastatin and parathyroid hormone on the lumbar vertebra of aging male Sprague-Dawley rats. Bone 2002;31:173–179.
- Zhang KQ, Chen JW, Li GF, Tian XY, Huang LF, Bao LH, Wang ML. Effect of intermittent injection of recombinant human parathyroid hormone on bone histomorphometry of ovariectomized rats. Acta Pharmacol Sin 2002;23:659–662.
- Rittmaster RS, Bolognese M, Ettinger MP, Hanley DA, Hodsman AB, Kendler DL, Rosen CJ. Enhancement of bone mass in osteoporotic women with parathyroid hormone followed by alendronate. J Clin Endocrinol Metab 2000;85:2129–2134.
- Black DM, Greenspan SL, Ensrud KE, Palermo L, McGowan JA, Lang TF, Garnero P, Bouxsein ML, Bilezikian JP, Rosen CJ; PTH Study Investigators. The effects of parathyroid hormone and alendronate alone or in combination in postmenopausal osteoporosis. N Engl J Med 2003;349:1207–1215.
- Finkelstein JS, Hayes A, Hunzelman JL, Wyland JJ, Lee H, Neer RM. The effects of parathyroid hormone, alendronate, or both in men with osteoporosis. N Engl J Med 2003;349:1216–1226.
- Zanchetta JR, Bogado CE, Ferretti JL, Wang O, Wilson MG, Sato M, Gaich GA, Dalsky GP, Myers SL. Effects of teriparatide [recombinant human parathyroid hormone (1‐34)] on cortical bone in postmenopausal women with osteoporosis. J Bone Miner Res 2003;18:539–543.
- Turner RT, Evans GL, Lotinum S, Lapke PD, Iwaniec UT, Morey-Holton E. Dose-response effects of intermittent PTH on cancellous bone in hindlimb unloaded rats. J Bone Miner Res 2007;22:64–71.
- Crandall C. Parathyroid hormone for treatment of osteoporosis. Arch Intern Med 2002;162:2297–2309.
- Neer RM, Arnaud CD, Zanchetta JR, Prince R, Gaich GA, Reginster JY, Hodsman AB, Eriksen EF, Ish-Shalom S, Genant HK, Wang O, Mitlak BH. Effect or parathyroid hormone (1-34) on fractures and bone mineral density in postmenopausal women with osteoporosis. N Engl J Med 2001;344:1434–1441.
- Dempster DW, Lindsay R Pathogenesis of osteoporosis. Lancet 1993;341:797–801.
- Turner RT, Riggs BL, Spelsberg TC. Skeletal effects of estrogen. Endocr Rev 1994;15:275–300.
- Spelsberg TC, Subramaniam M, Riggs BL, Khosla S. The actions and interactions of sex steroids and growth factors/cytokines on the skeleton. Mol Endocrinol 1999;13:819–28.
- Sato M, Zeng GQ, Turner CH. Biosynthetic human parathyroid hormone (1‐34) effects on bone quality in aged ovariectomized rats. Endocrinology 1997;138:4330–4337.
- Tsuchida T, Miyakoshi N, Kudo T, Tamura Y, Kasukawa Y, Suzuki K, Sato K. Restoring effect of human parathyroid hormone (1‐34) on trabecular connectivity in ovariectomized rats. Tohoku J Exp Med 2001;194:213–221.
- Dobnig H, Turner RT. Evidence that intermittent treatment with parathyroid hormone increases bone formation in adult rats by activation of bone lining cells. Endocrinology 1995;136:3632–3638.
- Friedl G, Turner RT, Evans GL, Dobnig H. Intermittent parathyroid hormone (PTH) treatment and age-dependent effects on rat cancellous bone and mineral metabolism. J Orthop Res 2007;25:1454–1464.
- Oxlund H, Dalstra M, Ejersted C, Andreassen TT. Parathyroid hormone induces formation of new cancellous bone with substantial mechanical strength at a site where it had disappeared in old rats. Eur J Endocrinol 2002;146:431–438.