Abstract
Efficient delivery of stem cells to target tissues is a major problem in regenerative medicine. Adipose derived stem cells have been proposed as important tools in cell therapy for recovering tissues after damage. Nevertheless, the ability of these ASCs to migrate or invade in order to reach the tissue of interest has not been tested so far. In this study we present evidence that the ASCs derived from obese subjects present a detrimental ability to migrate and invade in comparison with ASCs derived from control subjects. Besides, obese-derived ASCs are unable to respond to certain stimuli and to form enough capillaries after stimulation. We propose that the use of specific cytokines could overcome these deficiencies of the obese environment, offering a tool to optimize cell therapy.
Introduction
Most adult tissues are a reservoir of mesenchymal stem cell (MSCs) subpopulation that represents a proportion of the total cell number. This cell population is an attractive stem cell source for the regeneration of damaged tissues in clinical applications (Kern et al., Citation2006). Adipose tissue represents a promising source for MSCs that can be obtained by a less invasive method and in large quantities. This adipose stem cells (ASCs) have the potential to differentiate into adipogenic, osteogenic, chondrogenic and other mesenchymal lineages (Cheng et al., Citation2009; Galvez et al., Citation2009; Krampera et al., Citation2007; Rodriguez et al., Citation2004), and therefore could be used in regenerative medicine (Zuk et al., Citation2002).
ASCs can be easily isolated from adipose tissue by the explants technique (Galvez et al., Citation2009) and count with a distinct immune-phenotype (Rodeheffer et al., Citation2008). It has been shown that ASCs have different functional capability according to age, adipose tissue depot site and gender of subject (Madonna et al., Citation2011; Zhu et al., Citation2009). Besides, ASCs from non-obese or obese subjects show different properties related to their survival, proliferation, pluripotency maintenance and differentiation capacity (Onate et al., Citation2012).
A relevant characteristic of MSCs is their ability to migrate to areas of tissue injury. Numerous in vitro and in vivo studies have shown that cytokines and growth factors can influence in the trafficking of MSCs (Baek et al., Citation2011; Bernal et al., Citation2012; Galvez et al., Citation2006). Successful stem cell therapies require cells to transmigrate across the endothelium and invade their target tissue (Steingen et al., Citation2008). Nevertheless, transmigration capability of ASCs has not yet been investigated neither their dependence on their tissue origin. Besides, the vascular network also plays a critical role in the development and expansion of adipose tissue (Lin et al., Citation2008) and this process depends not only on the cell type but also on the environment (Efimenko et al., Citation2011). It has been described that several factors can stimulate both angiogenesis and migration capacities of cells (Galvez et al., Citation2001) but again the role of ASCs in this context is still largely unknown.
Therefore, we decided to study the migration, invasion and capillary-tubes formation capacity of ASCs in order to determine their potentiality as tools for regenerative medicine. We also hypothesized that obesity may affect the intrinsic properties of ASCs both in mouse and humans and that the treatment with different cytokines might improve their abilities.
Materials and methods
Reagents
The cells were grown in Dulbeco’s modified Eagle’s medium from Sigma (St Louis MO) supplemented with 10% FBS from Sigma (St Louis, MO), Pen/Strep, L-glutamine and Hepes from Lonza (Basel, Switzerland). The flow cytometry antibodies were from BDbiosciences (Franklin Lakes, NJ) and anti-NF-κB p65 was from Millipore Corp. (Billerica, MA). 4′,6-diamino-2-phenylindole dihydrochloride (DAPI) was from Invitrogen (Carlsbad, CA). The immunomount medium was ProLong from Invitrogen (Carlsbad, CA). Matrigel Basement Membrane Matrix Phenol Red Free was purchased from BD biosciences (Franklin Lakes, NJ) and PMA was from Sigma (St. Louis, MO). Transwell filters were from Corning Incorporated (Acton, MA) and all the cytokines used were obtained from Peprotech Inc. (Rocky Hill, NJ).
Culture of mouse and human adipose stem cells
Adipose stem cells were obtained from non-obese or obese mouse and human subjects. The mouse tissues were collected from adult C57BL6 mice as non-obese subject (non-obese ACSs) and from 4-months old diet-induced obese mice as obese subject (obese ACSs). Mice were maintained and used in accordance with the National Institutes of Health Animal Care and Use Committee. DIO rodent purified high fat diet (formula 58Y1) was obtained from TestDiet (IPS Product Supplies Ltd, London, UK).
The human cells were obtained from non-obese patients (BMI < 25 kg/m2) and from obese patients (BMI 25–30 kg/m2). Human adipose samples were obtained from insulin-resistant patients after bariatric surgery (Princesa Hospital; Females aged between 35 and 45 years), with a total of five non-obese patients (BMI < 25 kg/m2) and five obese (BMI > 30 kg/m2). Informed consent was obtained from all subjects and the sample collection conformed to the principles set out in the WMA Declaration of Helsinki and the NIH Belmont Report. Adipose stem cells were isolated from mouse and human tissues, sorted and expanded as described previously (Rodeheffer et al., Citation2008). Cells were incubated and maintained at 37 °C and they were sub-cultured under the same conditions. Cultures designated as eartly passage have received until 20 passages and those designated as late passage received more than 20 passages (to a maximum of 40 passages).
Flow cytometry analysis
The ASCs from non-obese and obese subjects were detached from flasks with trypsin and washed with PBS. Then the cells were stained with antibodies against surface markers CD34-PE, CD44-PeCy5, CD45-v450 and Sca1-PeCy7. The antibody dilution was 1:100, incubated for 25 min at 4 °C and was analysed using flow citometry in a BD FACS Canto II cytometer (BDbiosciences, Franklin Lakes, NJ).
Cell migration assay
The migration assay was performed using a 8-mm thickness transwell chambers (Corning Incorporated, Acton, MA). The cells were plated in the upper chamber of the Transwell at a density of 2.0 × 104 cells/80 μl and 600 µl were added in the lower chamber. Then, cells were incubated for 24 h at 37 °C in a humidify atmosphere containing 5% CO2, in order to allow migration across the membrane. In the lower chamber, 50 ng/ml IL8, 50 ng/ml SDF-1, 80 ng/ml MCP-1, 30 ng/ml TNF-α or 50 ng/ml HMGB1 were added to the medium in some experiments.
After 24 h of incubation, migrated cells appeared in the lower side of the Transwell membrane and were fixed with 4% glutaraldehide for 2 h and stained overnight with 2% Tolouidine Blue. Transwells were rinse in water and the membrane was cleaned in the upper side with a cotton swab to eliminate non-migratory cells. Finally the membrane was cut and placed onto a slide. We counted the number of cells at the lower side, in five different randomly selected 10× fields using a bright-field microscope Nikon 90i (Nikon Instruments, Burgerweeshuispad, Amsterdam, The Netherlands).
Wound-healing assay
In vitro wound-healing assay was used to assess cell motility in two dimensions in contrast to chamber assay. Cells were seeded into wells of 24-multiwell plates with medium containing 10% FBS. After the cells grew to confluence, wounds were made by sterile micropipette tip and then were washed with PBS to remove floating cells. The medium with or without 20 ng/ml TNF-α were added and the cultures continued for 24 h at 37 °C. Wound healing was photographed at time 0 h and 24 h by a IX51 Inverted Microscope (Olympus America Inc., Center Valley, PA) using a 4× magnification. The distance was determined by measuring the wound area at different time points with ImageJ and the values obtained were expressed as percentage migration. The experiments were done in triplicates.
Cell invasion assay
The invasion capacity was performed such as migration assay but previously the membrane was coated with 1% gelatin in PBS for 1 h at 37 °C.
In vitro angiogenesis assay
Matrigel basement membrane matrix was diluted 1:2 in cold medium DMEM. Then 60 μl was plated into a 96 well plates and allowed to gel for 30 min at 37 °C. The cells were seeded atop the Matrigel in absence or presence of PMA (100 ng/ml). After 24 h of incubation images were taken with a IX51 Inverted Microscope (Olympus America Inc., Center Valley, PA) using a 4× magnification. Capillary tubules were counted by branch point in a random field. Experiments were done in triplicates.
Inmunofluorescence
ASCs grown on sterile coverslips were serum starved for 24 h in DMEM and stimulated with 50 ng/ml TNF-α, 100 ng/ml MCP-1 or 100 ng/ml IL8 for 60 or 180 min. After stimuli, cells were fixed with 4% paraformaldehyde and permeabilized with 0.5% Triton X-100. Samples were then blocked with 1% donkey serum and incubated with the primary antibody, anti-NF-κB p65 and the appropriate secondary antibody conjugated with Cy3. After staining, the cells were counterstained with DAPI and the coverslips were mounted onto glass slides with Prolong. Confocal images were obtained with a Leica DM2500–TSC.SPE microscope at 40×.
Data analysis
Statistical analysis was performed using Graph Prism 5. Comparison of groups was performed by two ways ANNOVA. Values were expressed as mean ± standard error of the mean and data was considered significantly different when p < 0.05.
Results
Adipose stem cells isolation and characterization
Adipose stem cells (ASCs) were isolated from non-obese and obese adipose tissue of mouse and human, following the method previously described (Rodeheffer et al., Citation2008). Briefly, after dissecting the adipose tissue by enzymatic methods, cells were sorted and collected. The different cells lines (non-obese and obese ASCs derived from mouse or human) were characterized by flow cytometry, and all of them were positive for CD34+, CD44+, Sca1+ while negative for CD45- (). This expression profile was described in previous studies and identified an specific ASCs population (Rodeheffer et al., Citation2008). The following experiments were performed with these cells by using passages from 10 to 20 as early passage and from 20 to 40 as late passage cultures.
Table 1. Flow cytometry analysis of surface molecule expression on non-obese or obese mouse and human adipose stem cells. The table represents the mean of three independent experiments.
ASCs have the ability to migrate in response to stimuli
To analyse the ability of the ASCs to migrate and therefore reach the area of interest in certain tissues, migration assays were performed by using the Transwell system.
ASCs were plated in the upper chamber and their migration capability was analysed by counting cells at the lower side from randomized fields. Non-obese ASCs showed significant higher basal migration capacity than obese ASCs both in mouse and human samples (). Moreover the response of ASCs to different chemoattractant was measured by adding different cytokines to the lower chamber. These factors were SDF-1, TNF-α, MCP-1, HMGB1 and IL8; all of them have been described as chemoattractant signals that are capable of inducing migration in different stem cells lines (Bernal et al., Citation2012; Galvez et al., Citation2009). Intriguingly, mouse non-obese derived ASCs showed a significant enhanced response to MCP1 and IL8, while mouse obese derived ASCs responded better to MCP1, HMGB1 and IL8 (). Human non-obese ASCs enhance their migration capability in response to MCP-1 and HMGB1 () and obese ASCs increased similarly their migration after SDF-1, TNF-α, MCP-1, HMGB1 or IL8 stimulation (). Moreover, we tested if higher doses of these cytokines increased further their migration ability (). An slightly enhanced response to SDF-1 and MCP-1 was observed in mouse non-obese ASCs, while higher concentrations of TNF-α, HMGB1 or IL8 seemed not to have any effect on the mouse non-obese ASC response (, left side). Interestingly, higher doses of these cytokines had no effect on mouse obese ASC migration (, right side). In human non-obese ASCs a higher concentration of SDF-1 and TNF-α promoted an increase in migration while higher doses of, MCP-1 or IL8 decreased this capability (, left side). In the case of the human obese ASC, higher doses of MCP1 and HMGB1 doubled their ability to migrate across the membranes, while changes in the doses of SDF-1, TNF-α or IL-8 had no major effect on cells migration (, right side). Therefore, we can conclude that mouse and human obese derived ASCs show an impaired migration capability under basal condition. In contrast, in response to stimuli both mouse and human obese derived ASCs can enhance their migration ability. Finally, in order to study the possible pathway implicated on the cell migration response to different cytokines, we performed an inmunofluoresce to detect p65 translocation into the nucleus, in presence of TNF-α, MCP-1 or IL8 (Supplementary Figure 3). Both non-obese and obese ASCs showed a perinuclear localization of the p65 subunit in mouse and human samples (Supplementary Figure 3). Although both types of cells responded effectively to the different cytokines, NF-κB activation might not be the main pathway for controlling ASC migration.
Figure 1. Adipose stem cells migration and invasion. Migration Transwell assay performed in mouse adipose stem cells (A) and human adipose stem cells (B). ASCs were induced to migrate in presence of different cytokines at low concentration (50 ng/ml IL8 and SDF-1; 80 ng/ml MCP-1; 30 ng/ml TNF-α; 50 ng/ml HMGB1) and at high concentration (150 ng/ml IL8 and SDF-1; 240 ng/ml MCP-1; 90 ng/ml TNF-α; 150 ng/ml HMGB1). The graphic represents the mean of five independent experiments. Invasion assay performed in mouse adipose stem cells (C) and human adipose stem cells (D). Invasion capacity was analysed using gelatin coated membrane and ASCs were induced to transmigrate in presence of different cytokines (50 ng/ml IL8 and SDF-1; 80 ng/ml MCP-1; 30 ng/ml TNF-α; 50 ng/ml HMGB1). *p < 0.05; **p < 0.01; ***p < 0.001; +++p < 0.001 (+ differences between non-obese and obese ASCs and * differences inside the group). The graphic represents the mean of five independent experiments.
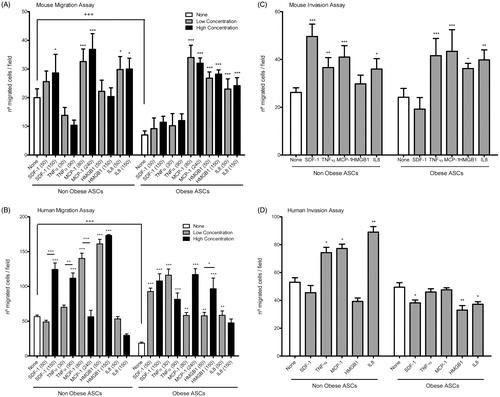
We also analysed the effect of ageing on the ASCs migration properties by using late cells passages (Supplementary Figures 1A and 1B). Both mouse non-obese and obese ASCs decreased their migration capability in late passages in comparison with early passages (Supplementary Figure 1A). In contrast, while a decrease in migration was observed in human non-obese ASCs late passages, human obese ASCs presented an increased migration in late passages (Supplementary Figure 1B). Similar results were obtained when we analyze the migration capacity in those cells by wound-healing assay (Supplementary Figure 2A and 2B). In both mouse non-obese and obese ASCs the migration capacity was affected by the passage used (Supplementary Figure 2A). In human ASCs, similarly to what we observed in the Transwell assay, only non-obese ASCs were negatively affected by late passages while obese ASCs increased their capability (Supplementary Figure 2B). In all cases, the presence of TNF-α increased their migratory properties. Collectively, these data indicate that ageing of ASCs may modify negatively their migratory properties.
Analysis of cell invasion capacity of ASCs
To reach the tissue of interest cells need not only to have the ability of migration but also should be able to cross the matrix and invade the tissue. Therefore, we analysed the ability of ASCs to transmigrate by using the previous Transwell migration assay but with membranes coated of 1% gelatine. ASCs showed no differences between non-obese and obese models both in mouse and human in relation to their transmigration capacity ().
Nevertheless, we also analysed the induced transmigration ability of ASCs in response to different cytokines. In the case of mouse ASCs, no major differences were observed between non-obese and obese models, except for the lack of response to SDF-1 of obese ASCs (). In contrast, in non-obese human ASCs the number of transmigrated cells after cytokines stimulation was significantly higher in comparison with obese human ASCs, indicating a possible defect in their responsiveness to stimuli (). To note that non-obese mouse ASCs can response stronger to SDF-1, while human non-obese ASCs response better to IL8 (), indicating that two different stimuli should be needed for each type of cell.
We also tested the invasion capacity of ASCs late passages (Supplementary Figures 1C and 1D). Both, in mouse and human ASCs the culture passage repeatedly decreased the invasion capacity in non-obese and obese ASCs.
With these results we can conclude that obese ASCs present an impaired responsiveness to certain cytokines stimuli that could imply a reduction in their invasion capacity. Moreover, ageing of ASCs may affect negatively their invasive responsiveness.
Angiogenic potential of adipose stem cells
Angiogenesis involves several cell types and it is responsible for the adipose tissue expansion. To evaluate the potential of ASCs to form capillary tubules, we performed an angiogenesis assay using the basement membrane Matrigel matrix to grow the cells. ASCs formed capillary-like networks in culture with all cells lines tested. Non-obese ASCs showed higher capacity to form capillaries in comparison with obese ASCs in mouse but not significantly in human subjects under basal conditions (). Interestingly, when PMA, an angiogenic stimuli, was added to the cultures, most ASCs were not responsive to this stimuli apart from human non-obese ASCs that increased their capacity to form tubule-like structures (). We next characterized the angiogenic potential of ASCs late passages. The capacity to form tubule like structures was altered by prolonged passaging in culture. Non-obese ASCs showed a decrease in their capacity to form tubule-like structure in late passages, both in mouse and human subjects (). However, obese ASCs late passages seemed not to modify their capacity to form tubules-like structures in mouse but decreased this ability in human obese ASCs.
Figure 2. Angiogenesis of adipose stem cells. Representative images of the capillaries structures formed on Matrigel by mouse adipose stem cells (A) and human adipose stem cells (B) both at early and late passages. ASCs were incubated in presence or absence of PMA (100 ng/ml) stimuli. Capillary tubules were quantified as branch points numbers and are represented in the graphic. +p < 0.05; ++p < 0.01; +++p < 0.001 (differences between non-obese and obese ASCs) and **p < 0.01; ***p < 0.001 (differences inside the group). The graphic represents the mean of six independent experiments.
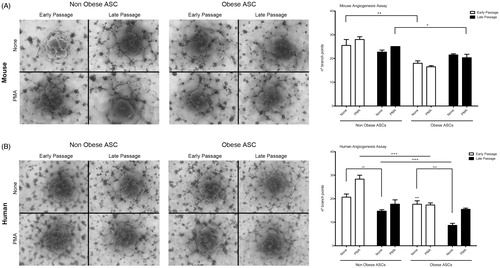
Taken together, these data suggest that obese ASCs cannot form tubular structures as efficiently as non-obese ASC. Besides, ageing of ASCs also affects negatively the response to form tubular structures both in mouse and human ASCs.
Conclusions
Our studies show that obese ASCs have an impaired capacity to migrate and present a lower response to invasion and angiogenic stimuli in comparison with non-obese ASCs, both in mouse and human models. The treatment with cytokines of mouse and human ASCs enhances their migration and invasion ability. Finally, ageing of ASCs affects negatively these migratory and invasive properties. Therefore, in general ASCs derived from obese subjects present lower capacity for therapeutic repair than ASCs derived from non-obese subjects.
Discussion
ASCs are useful for cell therapy and have already been used in several preclinical models of regeneration and in clinical trials (Correia et al., Citation2012; Herreros et al., Citation2012; Kim et al., Citation2011). Previous studies using ASCs from different patients showed impaired properties including differentiation, proliferation and cell fusion (Shu et al., Citation2012; Zhu et al., Citation2009). In the present study, we compared non-obese and obese ASCs from mouse and human, analysing their migration and invasion capacity together with their angiogenic potential. Recently, it has been published that ASCs derived from human morbidly obese patients showed an impaired growth behavior, differentiation capacity and angiogenic potential (Onate et al., Citation2012).
It would be beneficial to obtain stem cells in the shortest period of time to be used for clinical trials. We have isolated ASCs following a method previously described and both non-obese and obese ASCs show a similar surface markers expression (Rodeheffer et al., Citation2008). This ASCs population is positive for mesenchymal and stem cells marker but has no expression of hematopoietic lineage markers.
It has been described that different MSCs can migrate in vitro in response to a large set of chemotactic factors (Baek et al., Citation2011; Galvez et al., Citation2009). In line with these results this study shows that the migration capacity of ASC is affected by the obese environment and that can be modulated by treatment with several cytokines. Interestingly, in comparison with non-obese ASC, in both mouse and human models, obese ASC have less basal migration capacity. In addition, the response with cytokines differs between these cells lines. Mouse non-obese and obese ASCs showed an enhance response especially in the presence of MCP1. In contrast, human non-obese and obese ASCs showed similar migration response after TNF-α, MCP-1, HMGB1 or IL8 stimulation. Taken together, the results might suggest that the treatment with specific cytokines could restore and enhance basal levels of migration in ASCs.
Another mechanism that might be influenced by obesity is the invasion process (Schmidt et al., Citation2006; Teo et al., Citation2012). Numerous in vivo studies have shown that MSCs have the capability to migrate from the blood, cross the endothelial barrier and be incorporated into tissues (Chamberlain et al., Citation2007; Schmidt et al., Citation2006). We then explored the capacity of these ASCs to cross the matrix and invade the tissue. ASCs showed no differences between non-obese and obese models both in mouse and human, in relation to their transmigration capacity. In addition, in non-obese ASCs the cytokines stimulation was higher than obese ASCs, suggesting a possible impairment in their responsiveness to stimuli.
It has also been described that MSCs can participate in regeneration via paracrine effect and even help in angiogenic processes (Efimenko et al., Citation2011; Konno et al., Citation2010; Oswald et al., Citation2004). Interestingly, ASCs have been demonstrated to promote revascularization of ischemic tissue (Lee et al., Citation2012; Rehman et al., Citation2004). Besides, human obese ASCs have been demonstrated to have lower angiogenic capacity (Onate et al., Citation2012). In this work we present evidence that the potential of ASCs to form capillaries after angiogenic stimulation could be affected by the obese environment.
Finally, ageing has been demonstrated to be detrimental in different diseases including obesity, diabetes and inflammatory diseases (Ahima, Citation2009; Chung et al., Citation2009). In this study we demonstrate that ageing of ASCs could be negative for their migratory, invasive and angiogenic properties. These data should be taken into account for the isolation of ASCs from old patients.
In summary, ASCs derived from non-obese subjects show an impairment of their migration ability and have less responsiveness to invasion and angiogenesis stimuli, both in mouse and human. Even though obtained populations were phenotypically similar, they present major differences at the functional level. Our observations indicate that the therapeutic strategies based on ASC implantation would be inefficient when the cells origin involves an obese environment and that could be overcome by the use of specific cytokines.
Declaration of interest
The authors declare no potential conflicts of interest.
This study was supported by grants from the Spanish Ministry of Science and Innovation (SAF 2010-15239) to BGG. BGG acknowledges support from the “Ramon y Cajal” tenure track program of the Spanish Ministry of Science and Innovation (RYC2009-04669). The CNIC is supported by the Spanish Ministry of Economy and Competition and the Pro-CNIC Foundation. LMP and AB are supported by FPI fellowships from the Spanish Ministry.
Author contributions: L.M.P. and A.B. generated data. B.G.G. conceived the study, wrote the manuscript and discussed data.
Supplementary Material
Download PDF (139 KB)References
- Ahima RS. (2009). Connecting obesity, aging and diabetes. Nat Med, 15:996–7
- Baek SJ, Kang SK, Ra JC. (2011). In vitro migration capacity of human adipose tissue-derived mesenchymal stem cells reflects their expression of receptors for chemokines and growth factors. Exp Mol Med, 43:596–603
- Bernal A, San Martin N, Fernandez M, et al. (2012). L-selectin and SDF-1 enhance the migration of mouse and human cardiac mesoangioblasts. Cell Death Differ, 19:345–55
- Chamberlain G, Fox J, Ashton B, Middleton, J. (2007). Concise review: Mesenchymal stem cells: Their phenotype, differentiation capacity, immunological features, and potential for homing. Stem Cells, 25:2739–49
- Cheng NC, Estes BT, Awad HA, Guilak F. (2009). Chondrogenic differentiation of adipose-derived adult stem cells by a porous scaffold derived from native articular cartilage extracellular matrix. Tissue Eng Part A, 15:231–41
- Chung HY, Cesari M, Anton S, et al. (2009). Molecular inflammation: Underpinnings of aging and age-related diseases. Ageing Res Rev, 8:18–30
- Correia C, Grayson W, Eton R, et al. (2012). Human adipose-derived cells can serve as a single-cell source for the in vitro cultivation of vascularized bone grafts. J Tissue Eng Regen Med, [Epub ahead of print]. doi: 10.1002/term.1564
- Efimenko A, Starostina E, Kalinina N, Stolzing A. (2011). Angiogenic properties of aged adipose derived mesenchymal stem cells after hypoxic conditioning. J Transl Med, 9:10--15
- Galvez BG, Matias-Roman S, Albar JP, et al. (2001). Membrane type 1-matrix metalloproteinase is activated during migration of human endothelial cells and modulates endothelial motility and matrix remodeling. J Biol Chem, 276:37491–500
- Galvez BG, Sampaolesi M, Brunelli S, et al. (2006). Complete repair of dystrophic skeletal muscle by mesoangioblasts with enhanced migration ability. J Cell Biol, 174:231–43
- Galvez BG, San Martin N, Rodriguez C. (2009). TNF-alpha is required for the attraction of mesenchymal precursors to white adipose tissue in Ob/ob mice. PLoS One, 4:e4444 . doi: 10.1371/journal.pone.0004444
- Herreros MD, Garcia-Arranz M, Guadalajara H, et al. (2012). Autologous expanded adipose-derived stem cells for the treatment of complex cryptoglandular perianal fistulas: A phase III randomized clinical trial (FATT 1: Fistula Advanced Therapy Trial 1) and long-term evaluation. Dis Colon Rectum, 55:762–72
- Kern S, Eichler H, Stoeve J, et al. (2006). Comparative analysis of mesenchymal stem cells from bone marrow, umbilical cord blood, or adipose tissue. Stem Cells, 24:1294–301
- Kim M, Kim I, Lee SK, et al. (2011). Clinical trial of autologous differentiated adipocytes from stem cells derived from human adipose tissue. Dermatol Surg, 37:750–9
- Konno M, Hamazaki TS, Fukuda S, et al. (2010). Efficiently differentiating vascular endothelial cells from adipose tissue-derived mesenchymal stem cells in serum-free culture. Biochem Biophys Res Commun, 400:461–5
- Krampera M, Marconi S, Pasini A, et al. (2007). Induction of neural-like differentiation in human mesenchymal stem cells derived from bone marrow, fat, spleen and thymus. Bone, 40:382–90
- Lee HC, An SG, Lee HW, et al. (2012). Safety and effect of adipose tissue-derived stem cell implantation in patients with critical limb ischemia. Circ J, 76:1750–60
- Lin G, Garcia M, Ning H, et al. (2008). Defining stem and progenitor cells within adipose tissue. Stem Cells Dev, 17:1053–63
- Madonna R, Renna FV, Cellini C, et al. (2011). Age-dependent impairment of number and angiogenic potential of adipose tissue-derived progenitor cells. Eur J Clin Invest, 41:126–33
- Onate B, Vilahur G, Ferrer-Lorente R, et al. (2012). The subcutaneous adipose tissue reservoir of functionally active stem cells is reduced in obese patients. FASEB J, 26:4327–36
- Oswald J, Boxberger S, Jorgensen B, et al. (2004). Mesenchymal stem cells can be differentiated into endothelial cells in vitro. Stem Cells, 22:377–84
- Rehman J, Traktuev D, Li J, et al. (2004). Secretion of angiogenic and antiapoptotic factors by human adipose stromal cells. Circulation, 109:1292–8
- Rodeheffer MS, Birsoy K, Friedman JM. (2008). Identification of white adipocyte progenitor cells in vivo. Cell, 135:240–9
- Rodriguez AM, Elabd C, Delteil F, et al. (2004). Adipocyte differentiation of multipotent cells established from human adipose tissue. Biochem Biophys Res Commun, 315:255–63
- Schmidt A, Ladage D, Steingen C, et al. (2006). Mesenchymal stem cells transmigrate over the endothelial barrier. Eur J Cell Biol, 85:1179–88
- Shu W, Shu YT, Dai CY, Zhen QZ. (2012). Comparing the biological characteristics of adipose tissue-derived stem cells of different persons. J Cell Biochem, 113:2020–6
- Steingen C, Brenig F, Baumgartner L, et al. (2008). Characterization of key mechanisms in transmigration and invasion of mesenchymal stem cells. J Mol Cell Cardiol, 44:1072–84
- Teo GS, Ankrum JA, Martinelli R, et al. (2012). Mesenchymal stem cells transmigrate between and directly through TNF-alpha-activated endothelial cells. Stem Cells, 30:2472–86
- Zhu M, Kohan E, Bradley J, et al. (2009). The effect of age on osteogenic, adipogenic and proliferative potential of female adipose-derived stem cells. J Tissue Eng Regen Med, 3:290–301
- Zuk PA, Zhu M, Ashjian P, et al. (2002). Human adipose tissue is a source of multipotent stem cells. Mol Biol Cell, 13:4279–95