Abstract
Screening tests of hydroethanolic crude extracts of six species of Aspidosperma (Apocynaceae) against Staphylococcus aureus, Escherichia coli, Bacillus subtilis, and Pseudomonas aeruginosa were performed. Aspidosperma ramiflorum Muell. Arg. showed good activity against Bacillus subtilis with MIC and MBC of 15.7 and 125 μg/mL, moderate activity against Staphylococcus aureus with MIC and MBC of 250 and 500 μg/mL, and weak activity against Escherichia coli with MIC and MBC of 1000 μg/mL. Aspidosperma pyricolum Muell. Arg. (MIC/MBC 125/250 μg/mL) and Aspidosperma olivaceum Muell. Arg. (MIC/MBC 250/ > 1000 μg/mL) displayed moderate antibacterial activity against Bacillus subtilis. Separation of the crude extract of Aspidosperma ramiflorum was performed according to the usual acid–base process, which produces alkaloid mixtures and closely related metabolites. The basic fraction was active against Bacillus subtilis, Staphylococcus aureus, and Escherichia coli, with MICs of 31.2, 62.5, and 250 μg/mL, respectively. The basic fractions were more active than the acid fractions, probably because they contained some active alkaloids and/or closely related metabolites absent from the other fractions, or they contained a higher concentration of these active compounds.
Introduction
The species of Aspidosperma (Apocynaceae) are widespread, common trees in tropical America. The trees are 2–60 m tall, and grow in a variety of habitats from the dry fields of south-central Brazil, Paraguay, and Argentina to the Amazon valley floodplain, and at elevations from a few meters above sea level to approximately 2000 m in eastern Peru and Bolivia (CitationWoodson, 1951). Extracts of parts of these plants have been used in traditional medicine for the treatment of liver disorders and colds with fever, and as an analgesic (CitationObitz et al., 1997). Previous studies have also shown that the extracts of three Aspidosperma species used in folk medicine in Surinam display antimicrobial activity against Gram-positive bacteria (CitationVerpoorte et al., 1982).
Prompted by these reports, the present study investigated the in vitro antibacterial activity of ethanol extracts of stem bark from Aspidosperma dispermum Muell. Arg., A. olivaceum Muell. Arg., A. pyrifolium Martius, A. pyricolum Muell. Arg., A. polyneuron Muell. Arg., and A. ramiflorum Muell. Arg. In addition, we report our studies on the antibacterial activity of alkaloid extracts separated from the hydroethanolic crude extract of Aspidosperma ramiflorum. Separation was performed according to the usual acid–base process (CitationMaldoni, 1991), which produces alkaloid mixtures and closely related metabolites.
Material and methods
Plant material
The plants were collected from March to August 2004, at several places in Brazil. Aspidosperma dispermum was collected in Diamantina, state of Minas Gerais, by Dr. W. M. F. Neto; A. olivaceum was collected in Curitiba in Paraná by Dr. A. J. de Araújo; A. pyrifolium was collected in Piauí by Dr. W. M. F. Neto; A. pyricolum was collected iin Maricá, state of Rio de Janeiro, and A. polyneuron in Londrina, state of Paraná, by Dr. M. Faccione; and A. ramiflorum was collected in Porto Ferreira, state of São Paulo, by Dr. H. F. L. Filho. The plants were identified by W. M. F. Neto, A. J. Araújo, M. Faccione, and H. F. L. Filho, who made the collections, and were deposited and authenticated at the Herbarium of the State University of Campinas, Campinas, Brazil. The Herbarium voucher numbers are: Aspidosperma dispermum (86,367 Neto), Aspidosperma olivaceum (99,844), Aspidosperma pyrifolium (46,125), Aspidosperma pyricolum (49,176), Aspidosperma polyneuron (78,273), and Aspidosperma ramiflorum (32,802).
Preparation of extracts
Plant crude extracts were obtained by soaking 1 kg of air-dried stem bark in 70% ethanol at room temperature. After ethanol removal, the crude extract was added to a 10% acetic acid solution and kept at 5°C overnight. After filtration, the aqueous phase was first extracted with chloroform (acid fraction). The remaining aqueous phase was then basified to pH 10 and the resulting solution was extracted with chloroform (basic fraction). The extracts were dried under reduced pressure, yielding the hydroethanolic crude extract, acid fraction, and basic fraction (CitationMaldoni, 1991). The basic fraction was then spotted on Whatman paper (Whatman Cat. No. 1001-110) and the presence of alkaloids was determined using Dragendorff reagent (CitationStermitz et al., 1989). The masses obtained for the crude extract (CE) and their respective acid (AF) and basic (BF) fractions from the six species were: Aspidosperma dispermum (CE = 80.0 g, AF = 0.78, BF = 2.25), A. olivaceum (CE = 40.7 g, AF = 5.33, BF = 0.76), A. pyrifolium (CE = 67.2 g, AF = 17.0, BF = 7.42), A. pyricolum (CE = 96.6 g, AF = 8.40, BF = 0.96), A. polyneuron (CE = 111 g, AF = 8.70, BF = 12.1), and A. ramiflorum (CE = 122 g, AF = 7.70, BF = 11.6).
Microorganisms and growth conditions
The following microorganisms were used for detecting antibacterial compounds: Escherichia coli ATCC 25922, Pseudomonas aeruginosa ATCC 27853, Bacillus subtilis ATCC 6623, and Staphylococcus aureus ATCC 25923. Cultures of these bacteria were grown in nutrient broth (Difco Laboratories, Detroit, MI, USA) at 37°C and maintained on nutrient agar slants at 4°C. The following chemotherapeutic agents (Sigma Chemical Co., St. Louis, MO, USA) were included in the test as controls: tetracycline, vancomycin, and penicillin.
Antibacterial susceptibility testing
The minimum inhibitory concentrations (MICs) of all compounds and reference antibiotics were determined by microdilution techniques in Mueller–Hinton broth (Merck S.A., São Paulo, Brazil) as described by the CitationNational Committee for Clinical Laboratory Standards (2000). Inoculates were prepared in the same medium at a density adjusted to a 0.5 McFarland turbidity standard (108 colony-forming units (CFU)/mL) and diluted 1:10 for the broth microdilution procedure. Microtiter trays were incubated at 37°C, and the MICs were recorded after 24 h of incubation. Two susceptibility endpoints were recorded for each isolate. The MIC was defined as the lowest concentration of compound at which the microorganism tested did not demonstrate visible growth. Minimum bactericidal concentration (MBC) was defined as the lowest concentration yielding negative subcultures or only one colony.
Time-kill curve methodology
Prior to the experiments, the lower limit of bacterial quantification and the potential of compound carryover during the plating process were determined by methods proposed by the CitationNational Committee for Clinical Laboratory Standards (1997). Bacterial suspensions of Staphylococcus aureus and Bacillus subtilis were prepared in sterile water from a 24 h culture plate, and the resulting suspensions were adjusted to a 0.5 McFarland turbidity standard (approximately 108 CFU/mL). These suspensions were then serially diluted to produce bacterial concentrations of approximately 500, 100, 50, and 30 CFU/mL for each isolate. Portions (10 μL) were then removed from each suspension and plated on Mueller–Hinton agar plates for colony count determination. The plates were incubated at 37°C for 24 h. The lower limit of reproducibly detectable CFU per milliliter was defined as the most-dilute suspension that enabled viable-colony counting with a reproducibility coefficient of variation of <25%. For the compound carryover experiments, the standardized bacteria suspensions obtained as described above were diluted to inoculates of approximately 103 CFU/mL. An aliquot (100 μL) of each bacteria suspension was then added to 900 μL of either sterile water alone or sterile water plus the basic fraction from Aspidosperma ramiflorum (20 or 40 μg/mL), for a cell concentration of 102 per mL. Immediately following addition of the bacterial suspension to the aqueous solutions, portions (10 and 30 μL) were removed and streaked across Mueller–Hinton agar plates for colony count determination. Compound carryover was defined as a reduction in colony count from a compound–bacteria suspension of >25% compared to the colony count from a control sample (no basic fraction). The effects of the basic fraction of Aspidosperma ramiflorum on the growth of Staphylococcus aureus were assayed by preparing a standardized suspension (0.5 McFarland) of each isolate as described above. A 1:10 dilution of these suspensions was made by adding 1 mL of bacterial suspension to 9 mL of Mueller–Hinton broth with or without the desired amount of basic fraction. The antibacterial activity of the basic fraction was studied over a range of multiples of MIC encompassing 1–8 × MIC. Tests were performed in triplicate and incubated at 37°C. At predetermined time points (0, 3, 6, 12, and 24 h), a 0.1 mL sample was removed from each test solution, serially diluted in sterile water, and plated (10 μL) on Mueller–Hinton agar plates for colony count determination. Plates were incubated at 37°C for 24 h before the colonies were counted. Data from triplicate runs were averaged and plotted as log CFU per milliliter versus time, for each time point.
Results and discussion
Samples of air-dried stem bark from Aspidosperma dispermum, A. olivaceum, A. pyrifolium, A. pyricolum, A. polyneuron, and A. ramiflorum were extracted with 70% ethanol at room temperature. The results of screening tests of hydroethanolic crude extracts against Staphylococcus aureus, Escherichia coli, Bacillus subtilis, and Pseudomonas aeruginosa by the microdilution technique are given in . Different results were obtained for the six species against gram-positive and gram-negative bacteria. Aspidosperma ramiflorum showed good activity against Bacillus subtilis with MIC and MBC of 15.7 and 125 μg/mL, moderate activity against Staphylococcus aureus with MIC and MBC of 250 and 500 μg/mL, and weak activity against Escherichia coli with MIC and MBC of 1000 μg/mL. Aspidosperma pyricolum (MIC/MBC 125/250 μg/mL) and A. olivaceum (MIC/MBC 250/ >1000 μg/mL) displayed moderate antibacterial activity against Bacillus subtilis. Aspidosperma dispermum, A. pyrifolium, and A. polyneuron were inactive against standard strains at concentrations >1000 μg/mL. All six species were totally inactive against Pseudomonas aeruginosa (MIC >1000 μg/mL).
Table 1. Minimum inhibitory concentration (MIC) and minimum bactericidal concentration (MBC) of ethanol crude extracts of Aspidosperma species.
The use of and search for drugs and dietary supplements derived from plants have accelerated in recent years (CitationCowan, 1999; CitationSanches et al., 2005). Ethnopharmacologists, botanists, microbiologists, and natural-products chemists are combing the earth for phytochemicals and “leads” which could be developed for the treatment of infectious diseases (CitationCowan, 1999). Although there are dozens of plant secondary metabolites that show activity in the micro- to submicromolar range, at least against Gram-positive species, surprisingly little is known about their mechanisms of action (CitationGibbons, 2004).
Plants produce bioactive molecules that allow them to interact with other organisms in their environment. Many of these substances are important in defense against herbivores, and contribute to disease resistance. Plants, therefore, can be promising sources of antimicrobial agents (CitationSanches et al., 2005; CitationTiuman et al., 2005; CitationMincoff et al., 2006; CitationVidotti et al., 2006).
According to CitationBriskin (2000), the beneficial medical effects of plant materials typically result from the combinations of secondary products present in the plant. That the medicinal actions of plants are unique to particular plant species or groups is consistent with this concept, because the combinations of secondary products in a particular plant are often taxonomically distinct (CitationWink, 1999). In the present study, hydroethanolic crude extracts from six species of the genus Aspidosperma showed different antibacterial activities, and this may relate to reported differences in the pharmacological properties of these plant materials (CitationObitz et al., 1997). Moreover, within a particular species of Aspidosperma, levels of a particular product can also be affected by environmental factors such as soil nutrients.
Species of the genus Aspidosperma are known to contain indole alkaloids, several of which possess important pharmacological properties (CitationOndetti & Deulofeu, 1961; CitationKutney et al., 1981). These reports prompted us to carry out separation of the hydroethanolic crude extract of Aspidosperma ramiflorum. Separation was performed according to the usual acid–base process (CitationMaldoni, 1991), which produces both alkaloid mixtures and closely related metabolites. Alkaloids were detected by Dragendorff reagent. The antibacterial activities of the BF and AF against the standard strains are given in . The basic fraction was active against Bacillus subtilis, Staphylococcus aureus, and Escherichia coli with MICs of 31.2, 62.5, and 250 μg/mL, respectively. The acid fraction showed weak activity against Bacillus subtilis and Staphylococcus aureus (MICs at 500 μg/mL) and was inactive against Escherichia coli (MIC > 1000 μg/mL). The MICs of the reference drugs used in this study were similar to those reported elsewhere.
Table 2. Minimum inhibitory concentration (MIC) of basic and acid fractions of Aspidosperma ramiflorum.
As a result of this finding, time-kill studies were performed to determine whether the basic fraction of Aspidosperma ramiflorum had bactericidal activity. The kinetics of bacterial killing was evaluated against Staphylococcus aureus at four concentrations of the basic fraction (1 ×, 2 ×, 4 ×, and 8 × the MIC). No antibacterial carryover was noted with the sampling methodology used. The lowest reproducibly quantifiable concentration of bacteria was 50 CFU/mL. Cell viability at predetermined time points (0–24 h) was measured. Viable cells of Staphylococcus aureus were reduced by 2 log-units within 6 h after exposure to 4–8 times the MIC of the basic fraction. Control (no drug) showed a 6 log-unit increase in viable count/mL by 6 h for Staphylococcus aureus (). The minimum concentration of an antimicrobial necessary to kill an organism should be equal to or greater than the MIC for that microbe. In this study, however, the concentrations of 1 × and 2 × the MIC had similar inhibitory effects on the growth of these organisms. Time-kill data that correspond to the MIC, plus or minus one dilution, represent a transition from minimum activity (subinhibitory concentrations) to maximal antibacterial effect (suprainhibitory concentrations), and encompass the concentration that produces 50% of the maximal effect.
Figure 1. Time-kill of basic fraction against S. aureus. ▴, control; •, 1 × MIC, ○, 2 × MIC; □, 4 × MIC; ▪, 8 × MIC.
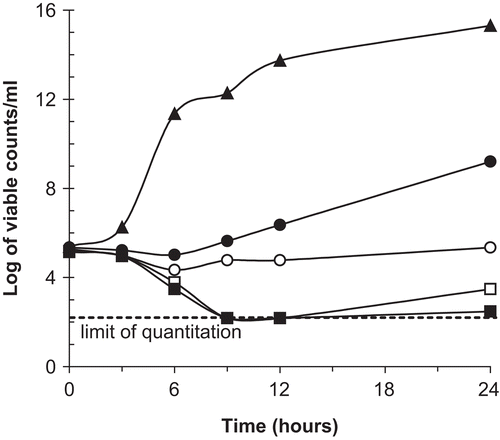
In the screening of a series of medicinal plants from Surinam for antimicrobial activity, extracts from three species of Aspidosperma were among the most active antimicrobial samples (CitationVerpoorte et al., 1982). In a preliminary report, these authors mentioned that the active compounds in Aspidosperma marcgraviarum and A. excelsum were dimeric alkaloids identical to aspidexcine and aspidexcelsine. Further, they identified these alkaloids as tetrahydrosecamine and decarbomethoxytetrahydrosecamine.
In the present study, the basic fractions were more active than the acid fractions, probably because they contained some active alkaloids and/or closely related metabolites that were absent in the other fractions, or they contained a higher concentration of these active compounds. Refractionation of the alkaloid crude fractions and further bioassay will reveal the nature of these active compounds.
Acknowledgements
This study was supported by Conselho Nacional de Desenvolvimento Científico e Tecnológico (CNPq), Capacitação e Aperfeiçoamento de Pessoal de Nível Superior, (Capes), Fundação Araucária, and Programa de Pós-graduação em Ciências Farmacêuticas da Universidade Estadual de Maringá. The authors would like to thank Marinete Martinez Vicentin for skillful technical assistance.
Declaration of interest: The authors report no conflicts of interest.
References
- Briskin DP (2000): Medicinal plants and phytomedicines. Linking plant biochemistry and physiology to human health. Plant Physiol 124: 507–514.
- Cowan MM (1999): Plant products as antimicrobial agents. Clin Microbiol Rev 12: 564–582.
- Gibbons S (2004): Anti-staphylococcal plant natural products. Nat Prod Rep 21: 263–277.
- Kutney JP, Noda M, Lewis NG, Monteiro B, Mostowicz D, Worth BR (1981): Studies in the dihydropyridine series 5. Synthesis of pyridocarbazole alkaloides-olivacine and guatambuine. Heterocycles 16: 1469–1472.
- Maldoni B (1991): Alkaloids: isolation and purification. J Chem Educ 68: 700–703.
- Mincoff PC, Cortez DAG, Nakamura TU, Nakamura CV, Dias Filho BP (2006): Isolation and characterization of a 30 kDa antifungal protein from seeds of Sorghum bicolor. Res Microbiol 157: 326–332.
- National Committee for Clinical Laboratory Standards (1997): Methods for determining bactericidal activity of antimicrobial agents. Proposed guideline M26-P. Wayne, PA, NCCLS.
- National Committee for Clinical Laboratory Standards (2000): Methods for dilution antimicrobial susceptibility tests for bacteria that grow aerobically. Approved standard M7-A5. Wayne, PA, NCCLS.
- Obitz P, Mendonza LA, Aimi N, Sakai S, Stöckigt J (1997): Alkaloids from cell cultures of Aspidosperma quebracho-blanco. In: Pelletier SW, ed., Alkaloids: Chemical and Biological Perspectives, Vol. 9. Oxford, Pergamon, pp. 236–245.
- Ondetti MA, Deulofeu V (1961): Alkaloids from Aspidosperma austreale Mull Argov – structure of olivacine and u-alkaloid C (guatambuine). Tetrahedron 15: 160–166.
- Sanches NR, Garcia Cortez DA, Schiavini MS (2005): An evaluation of antibacterial activities of Psidium guajava (L). Braz Arch Biol Technol 48: 429–436.
- Stermitz FR, Belofsky GN, Ng D, Singer MC (1989): Quinolizidine alkaloids obtained by Pedicularis semibarbata (Scrophulariaceae) from Lupinus fulcratus (Leguminosae) fail to influence the specialist herbivore Euphydryas editha (Lepidoptera). J Chem Ecol 15: 2521–2530.
- Tiuman TS, Nakamura TU, Cortez DAG, Dias Filho BP, Diaz JAM, Souza W, Nakamura CV (2005): Antileishmanial activity of parthenolide, a sesquiterpene lactone isolated from Tanacetum parthenium. Antimicrob Agents Chemother 49: 176–182.
- Verpoorte R, Ruigrow CLM, Svendsen B (1982): Medicinal plants of Surinam. II Antimicrobial active alkaloids from Aspidosperma marcgravianum. Planta Med 46: 149–152.
- Vidotti GJ, Zimmermann A, Sarragiotto MH, Nakamura CV, Dias Filho BP (2006): Antimicrobial and phytochemical studies on Pedilanthus tithymaloides. Fitoterapia 77: 43–46.
- Wink M (1999): Functions of plant secondary metabolites and their exploitation in biotechnology. Annual plant reviews, Vol. 3. 370 pp. Sheffield: Sheffield Academic Press Ltd.
- Woodson RE (1951): Studies in the Apocynaceae VIII: an interim revision of genus Aspidosperma Mart. & Zucc. Ann Mo Bot Gard 38: 119–204.