Abstract
Escin, a group of chemically related triterpenic glycosides, is widely used in commercial preparations for the treatment of venous insufficiency. Since the zygotic embryo cotyledons accumulate the highest amount of escin, it is currently extracted from the seeds of horse chestnut, Aesculus hippocastanum L. (Hippocastanaceae), on a large scale. As this material is available during only short period of the year, we studied the possibility of using plant tissue culture to obtain escin. For this purpose, the content of escin in androgenic embryos and hairy root cultures of horse chestnut was studied. Escin content was found to be dependent on the stage of androgenic embryo development and the type of phytoregulator supplemented to the nutritive medium. In the absence of phytoregulators, androgenic embryos at the globular stage of development contained approximately four times less escin than those at the cotyledonary stage. Inclusion of various phytoregulators in the nutritive media stimulated escin production. Among them, 2,4-dichlorophenoxyacetic acid (2,4-D) showed the most pronounced effect, with escin content almost reaching that found in zygotic embryos (6.77% versus 6.96%). Two hairy root clones produced substantial amounts of escin (3.57% and 4.09%), less than zygotic embryos, but higher than cotyledonary embryos on phytoregulator-free medium.
Introduction
Plants serve as a valuable source of many compounds used in the medical, pharmaceutical and cosmetic industries. Horse chestnut – Aesculus hippocastanum L. (Hippocastanaceae) – seed extract is widely used for the treatment of peripheral vascular disorders (CitationBombardelli et al., 1996), and in cosmetics as a remedy against cellulitis (CitationMatsuda et al., 1997). It exhibits potent anti-inflammatory (CitationMatsuda et al., 1997), antitumor, antiviral (Yang et al., Citation1999a) and antioxidative (CitationMasaki et al., 1995; CitationWilkinson & Brown, 1999) effects as well.
An active component of the horse chestnut seed extract is a group of chemically related triterpenic glycosides, known as escin. The main source of these compounds are the seed cotyledons, but they are also present in trace amounts in the seed integuments, the barks, buds, leaves and the immature fruit pericarps of A. hippocastanum (CitationBombardelli et al., 1996) and other species within the genus Aesculus (CitationWei et al., 2004, Yang et al., Citation1999b). A small amount of escin was detected in native roots of Aesculus turbinata Blume (CitationYoshikawa et al., 1999) and seedling roots of Aesculus parviflora Walt. (CitationKarting et al., 1966).
As the zygotic embryos are the main site of escin accumulation, horse chestnut seeds are the sole industrial source of escin. This raw material is available only for a short period during the year and the pharmacologically active tissue (zygotic embryo) presents only a small part of the seed (up to 500 mg per seed fresh weight). Furthermore, CitationCaldas and Machado (2004) found high levels of lead in leaves and seed extracts of horse chestnut. Taking all this into consideration, plant cell and organ culture may be an alternative solution for escin production.
CitationProfumo et al. (1991) and CitationGastaldo et al. (1994) demonstrated the presence of substantial amounts of escin in embryogenic calli and somatic embryos of A. hippocastanum, which remained high after two years of culture (CitationProfumo et al., 1991), and could be increased further by applying certain plant growth regulators (CitationProfumo et al., 1992).
Our previous studies showed significant growth and multiplication, as well as simple nutritive demands of both androgenic embryos (CitationĆalić et al., 2003) and hairy roots (CitationZdravković-Korać et al., 2003) of horse chestnut. Therefore, we assume that these cultures can be used as an alternative source for large-scale escin production. The present study was aimed at determining the content of escin in horse chestnut hairy roots and androgenic embryos grown on media supplemented with various phytoregulators.
Materials and methods
Plant material
For androgenic embryo induction, anthers were obtained from a 100-year-old horse chestnut tree (No. P1/15) growing in the Botanical Garden “Jevremovac”, University of Belgrade, according to previously described protocol (CitationĆalić et al., 2003). In brief, anthers bearing microspores at the uninucleated stage of development were macerated, suspended in 100 mL of liquid Murashige and Skoog`s (MS) mineral solution (CitationMurashige & Skoog, 1962) medium containing 2,4-dichlorophenoxyacetic acid (2,4-D) and 6-furfurylaminopurine (Kin), 1 mg/L each, and sieved through a 50 μm mesh. The cultures were shaken on a platform shaker (85 rpm) in darkness for four weeks. Cell suspensions produced after four weeks were mixed with equal volumes of cooled (30°C) MS medium supplemented with 0.01 mg/L 2,4-D, 1 mg/L Kin and 0.7% agar and dispensed in Petri dishes. Solid medium of the same composition was used to cultivate regenerated embryos and to maintain the cultures. Development of androgenic embryos is asynchronous, so that embryos at different developmental stages (globular, heart-like, torpedo-like and cotyledonary) were observed in the same culture. Embryos at early heart-like and torpedo stages were transferred to phytoregulator-free MS medium and cultivated for a month. After one month, those among them reaching the cotyledonary stage (5-10 mm, ) were transferred to MS medium supplemented with 0.5 mg/L each of various phytoregulators: 2,4-dichlorophenoxyacetic acid (2,4-D), naphthalene acetic acid (NAA), indole-3-butyric acid (IBA), 6-furfurylaminopurine (Kin), 6-benzylaminopurine (BA), or abscisic acid (ABA), and grown for another month.
Figure 1. Horse chestnut androgenic embryo at the cotyledonary stage of development cultured on MS solid phytoregulator-free medium.
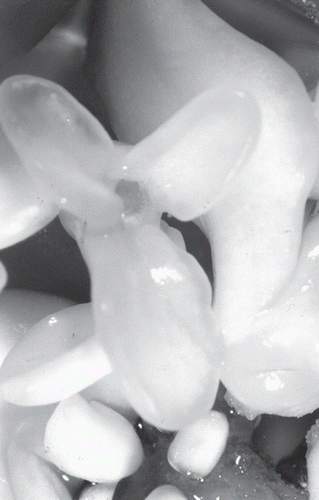
The horse chestnut hairy root clones were obtained by inoculation of androgenic embryos with Agrobacterium rhizogenes strain A4GUS, as was previously described (CitationZdravković-Korać et al., 2003, Citation2004). Briefly, the androgenic embryos were wounded by puncturing with a hypodermic injection needle, immersed in a 2-day-old bacteria suspension for 5 min, blotted dry on a sterile filter paper tissue for a few seconds, and placed on solid MS phytoregulator-free medium. After 72 h co-cultivation, the androgenic embryos were transferred to medium of the same composition, supplemented with 500 mg/L cefotaxime (Jugoremedia, Zrenjanin, Serbia) for bacteria elimination. Single roots emerging from wounded sites were isolated and transferred to fresh medium of the same composition. Transgenic clones were selected for the highest growth. The best clones 31, 39, 43, and 47 were further propagated and the rest of them were discarded. The selected clones were grown in liquid MS phytoregulator-free medium on a platform shaker at 85 rpm and subcultivated biweekly.
For estimation of the growth capacity of hairy root clones, approximately 500 mg of both hairy roots and untransformed roots were cultivated in a 100 mL Erlenmeyer flask containing 50 mL of liquid MS phytoregulator-free medium for four weeks. Ten samples were taken for each clone, and the experiment was repeated three times (n = 30). The growth index was calculated using the following formula: W-W0/W0, where W0 is the initial root weight and W is root weight after four weeks in culture.
Basal medium contained MS mineral salts, 2% sucrose, and was supplemented with the following (mg/L): pantothenic acid 10, nicotinic acid 5, vitamin B1 2, adenine sulfate 2, myo-inositol 100 and casein-hydrolysate 200. All media were sterilized by autoclaving at 0.9 × 105 KPa and 114°C for 25 min. The cultures were maintained at 25 ± 1°C under 16 h photoperiod, at irradiance of 33–45 μmol m−2 s−1 provided by cool white fluorescent tubes.
Analysis of escin
Escin content was determined in globular and cotyledonary embryos and four hairy root clones grown on phytoregulator-free medium and cotyledonary embryos grown on media with various phytoregulators. Leaves and seeds collected from the nature were used as a control. Leaves were harvested in June and seeds were collected in October. The seeds were stored in dry and cooled storage till January, and then the zygotic embryos were isolated and subjected to escin analysis. The analysis of androgenic embryos and hairy roots was conducted after four weeks of culture.
Escin analysis was conducted according to the German Pharmacopoeia (CitationDAB, 1991). In brief, plant material was lyophilized and 1 g of powdered tissue was extracted with 100 mL of 65% methanol (v/v) 30 min over a water bath under reflux. Then, 30 mL of extract was evaporated till dryness and 20 mL 0.1 N HCl was added. Re-extraction was done with a mixture of 20 mL n-propanol and 50 mL chloroform and the extract was evaporated till dryness and then washed with ether. The dried residue was dissolved in anhydrous acetic acid into a 50 mL volumetric flask. Five mL of solution was transferred into a 25 mL volumetric flask and ferric chloride reagent (75 mg of ferric chloride with 50 mL anhydrous acetic acid and 50 mL concentrated sulfuric acid, freshly prepared) was added till 25 mL. The solution was heated at 60°C over a water bath for 25 min and then cooled to room temperature. Control/blank was prepared with 5 mL of anhydrous acetic acid. Absorbance was measured on 540 nm. The amount of escin was calculated from the expression:
where A540 is the absorbance and m is the amount of sample in grams.
Three replicates, taken from different Petri dishes, were used for each treatment, and the experiment was repeated three times (n = 9). Percentage data were subjected to angular transformation prior to analysis. The means were separated using the LSD test at p ≤0.05, and inversely transformed for the presentation.
Results and discussion
Androgenic embryos grown on phytoregulator-free medium, at both the globular and the cotyledonary stage of development produced escin, but its amount in the cotyledonary embryos was four times higher than in the globular embryos (). Therefore, only embryos at the cotyledonary stage () were used for further studies. Since escin content in the zygotic embryo cotyledons of stored horse chestnut seeds was found to increase gradually, reaching the maximal value in January (CitationProfumo et al., 1987), we used the zygotic embryos at this stage as a control for comparison with our samples. Cotyledonary embryos cultured on phytoregulator-free medium produced an almost three times lower amount of escin than zygotic embryos (2.43% versus 6.96%) and a similar amount to the leaves (2.43% versus 3.05%). The inclusion of various phytoregulators in the nutritive media increased escin production in cotyledonary androgenic embryos 2 to 2.3 times (). Similarly, CitationProfumo et al. (1992) found that embryogenic calli produced 2–3 times more escin when grown on phytoregulator-supplemented media compared to phytoregulator-free medium. In our study, 2,4-D showed the most pronounced effect, with escin production almost reaching that of the zygotic embryos (6.77% versus 6.96%). 2,4-D is a synthetic auxin and strong herbicide and is considered to be a stressor that provokes numerous physiological responses to greater extent than naturally occurring auxins (CitationIkeda-Iwai et al., 2003).
Table 1. Escin content in androgenic embryos and hairy root cultures of Aesculus hippocastanum.
Our results contribute to a notion that escin formation is an intrinsic feature of a horse chestnut embryo, irrespective of the process of its induction (androgenesis, somatic or zygotic embryogenesis), as well as the donor tissue used for embryo induction and the ploidy level of an embryo. CitationProfumo et al. (1994) found only slightly higher escin content in somatic embryos originating from the zygotic embryo cotyledons (9.8%), than in somatic embryos induced from stem segments (7.5%), the organs producing the highest and the lowest amount of escin in nature. In this study, it was demonstrated that haploid androgenic embryos, induced from uninucleated microspores, also produced escin, and its content varied between 2.43% and 6.77%, depending on the phytoregulator supplemented to the nutritive medium. Variations in escin content obtained in these two studies are likely due to different combinations and concentrations of phytoregulators applied.
Horse chestnut hairy root clones exhibited high biomass production (), stable growth and economical nutritive demands. We have chosen transgenic clones with stable growth and the highest biomass production for the determination of escin content. Clones 31, 39, 43 and 47 increased their mass 7.36 ± 0.97, 7.41 ± 1.57, 5.71 ± 1.25 and 7.73 ± 0.78-fold respectively, over the initial mass, in four months of culture in simple liquid phytoregulator-free medium, while the untransformed roots increased their initial mass 0.9 ± 0.03-fold. Furthermore, untransformed roots perished after 1-2 weeks’ subculture in phytoregulator-free medium, whereas the hairy roots grew at an unchanged rate for nine years.
All hairy root clones tested produced escin, but exhibited substantial differences in escin content (). Clones 39 and 47 produced the highest amount of escin, 3.57% and 4.09%, respectively – less than zygotic embryos and cotyledonary androgenic embryos on phytoregulator supplemented media, but higher than leaves and cotyledonary androgenic embryos on phytoregulator-free medium.
The hairy root lines were obtained from independent transformation events and differ from each other in both the position of the TL-DNA insertion sites and the TL-DNA copy number, as was confirmed by Southern hybridization (CitationZdravković-Korać et al., 2004). The position effect influences the expression of the transgenes and consequently the growth and morphology of the hairy root clones. We found no correlation of escin content and the TL-DNA copy number, as clone 31, with the highest copy number of the TL-DNA (CitationZdravković-Korać et al., 2004) contained substantially lower amount of escin than clones 39 and 47 (). Thus, the differences in escin content among the hairy root clones are probably due to the TL-DNA position effect and the level of the transgene expression, which is in accordance with the results of CitationBatra et al. (2004) and CitationChaudhuri et al. (2005). CitationBatra et al. (2004) pointed out the importance of the TL-DNA presence for ajmalicine production in hairy roots of Catharanthus roseus L. (Apocyanaceae). In a previous study, the presence of the whole rolA, -B, -C and -D cassette was demonstrated for all horse chestnut hairy root clones (CitationZdravković-Korać et al., 2003). This is a prerequisite for high biomass productivity, as hairy roots containing the TL-DNA grow vigorously, whilst those containing the TR-DNA alone generally grow very slowly.
Commercial in vitro production of any particular compound demands stable cell lines exhibiting high yields and growth potential. Although plant cell suspension cultures have been recognized as a method of choice in some cases (CitationLanghansová et al., 2005; CitationMin et al., 2006), insufficient yield and metabolic instability of the suspension cultures often limit this approach. However, differentiated tissues display both genetic and biochemical stability (CitationFlores & Curtis, 1992). For this reason, genetically transformed roots are particularly interesting due to their capacity for long-term growth (CitationFlores et al., 1999; CitationMomčilović et al., 2001; CitationZdravković-Korać et al., 2003), and therefore widely used as a source of many useful compounds (CitationFlores & Curtis, 1992; CitationOksman-Caldentey & Hiltunen, 1996; CitationFlores et al., 1999).
In conclusion, horse chestnut androgenic embryos and hairy roots produce high amounts of escin, which can be manipulated by the addition of phytoregulators. We find this approach promising for resolving the problems associated with commercial production of escin. This method enables high biomass production, even availability of the phytochemical all year round, simplification of the extraction procedure and manipulation of the factors affecting secondary metabolite biosynthesis.
Acknowledgements
The authors are grateful to Dr. Dragan Vinterhalter for critical reading of the manuscript.
Declaration of interest
This work was supported by the Ministry of Science and Environment Protection of Serbia, grant no. 143026B.
References
- Batra J, Dutta A, Singh D, Kumar S, Sen J (2004): Growth and terpenoid indole alkaloid production in Catharanthus roseus hairy root clones in relation to left- and right-termini-linked Ri T-DNA gene integration. Plant Cell Rep 23: 148–154.
- Bombardelli E, Morazzoni P, Griffini A (1996): Aesculus hippocastanum L. Fitoterapia 67: 483–511.
- Caldas ED, Machado LL (2004): Cadmium, mercury and lead in medicinal herbs in Brazil. Food Chem Toxicol 42: 599–603.
- Chaudhuri KN, Ghosh B, Tepfer D, Jha S (2005): Genetic transformation of Tylophora indica with Agrobacterium rhizogenes A4: Growth and tylophorine productivity in different transformed root clones. Plant Cell Rep 24: 25–35.
- Ćalić D, Zdravković-Korać S, Jevremović S, Guć-Šćekić M, Radojević Lj (2003): Efficient haploid induction in microspore suspension culture of Aesculus hippocastanum and karyotype analysis. Biol Plant 47: 289–292.
- DAB (1991): Deutsches Arzneibuch 10, Amtliche Ausgabe, Band III, Hippocastani semen, Deutscher Apotheker Verlag Stuttgart Govi-Verlag GmbH Frankfurt.
- Flores HE, Curtis WR (1992): Approaches to understanding and manipulating the biosynthetic potential of plant roots, in: Pedersen H, Mutharasan R, DiBiasio D, eds. Biochemical Engineering VII: Cellular and Reactor Engineering. Ann NY Acad Sci, pp. 188–209.
- Flores HE, Vivanco JM, Loyola-Vargas VM (1999): “Radicle” biochemistry: The biology of root-specific metabolism. Trends Plant Sci 4: 220–226.
- Gastaldo P, Caviglia AM, Profumo P (1994): Aesculus hippocastanum (horse chestnut): In vitro culture and production of aescin, in: Bajaj YPS, ed., Biotechnology of Medicinal and Aromatic Plants. Berlin, Springer Verlag, pp. 1–12.
- Ikeda-Iwai M, Umehara M, Satoh S, Kamada H (2003): Stress-induced somatic embryogenesis in vegetative tissues of Arabidopsis thaliana. Plant J 34: 107–114.
- Karting T, Graune FJ, Herbst R (1966): Saponins distribution during the different stages of germination and development of Aesculus hippocastanum. Planta Med 14: 121–125.
- LanghansováL, Maršík P, Vaněk T (2005): Production of saponins from Panax ginseng suspension and adventitious root cultures. Biol Plant 49: 463–465.
- Masaki H, Sakaki S, Atsumi T, Sakurai H (1995): Active-oxygen scavenging activity of plant extracts. Biol Pharm Bull 18: 162–166.
- Matsuda H, Li Y, Murakami T, Ninimiya K, Araki N, Yoshikawa M, Yamahara I (1997): Effects of aescins Ia, Ib, IIa, IIb from horse chestnut, the seeds of Aesculus hippocastanum on acute inflammation in animals. Biol Pharm Bull 20: 1092–1095.
- Min SR, Woo JW, Jeong WJ, Han SK, Lee YB, Liu JR (2006): Production of human lactoferrin in transgenic cell suspension cultures of sweet potato. Biol Plant 50: 131–134.
- Momčilović I, Grubišić D, Nešković M (2001): Transgenic Gentiana species (Gentian, in: Bajaj YPS, ed., Biotechnology in Agriculture and Forestry, Transgenic Crops III. Berlin, Heidelberg, Springer Verlag, pp. 123–138.
- Murashige T, Skoog F (1962): A revised medium for rapid growth and bioassay with tobacco tissue culture. Physiol Plant 15: 473–497.
- Oksman-Caldentey KM, Hiltunen R (1996): Transgenic crops for improved pharmaceutical products. Field Crops Res 45: 57–69.
- Profumo P, Gastaldo P, Martinucci R (1987): Variations in aescin content in Aesculus hippocastanum seeds during the year. Fitoterapia 58: 184–186.
- Profumo P, Caviglia AM, Gastaldo P, Dameri RM (1991): Aescin content in embryogenic callus and in embryoids from leaf explants of Aesculus hippocastanum. Planta Med 57: 50–52.
- Profumo P, Caviglia AM, Gastaldo P (1992): Formation of aescin glucosides by callus tissue from cotyledonary explants of Aesculus hippocastanum L. Plant Sci 85: 161–164.
- Profumo P, Caviglia AM, Gastaldo P (1994): Aescin formation in calli and embryoids from cotyledon and stem explants of Aesculus hippocastanum L. J Pharm Pharmacol 46: 924–925.
- Wei F, Ma LY, Jin WT, Ma SC, Han GZ, Khan IA, Lin RC (2004): Anti-inflammatory triterpenoid saponins from the seeds of Aesculus chinensis. Chem Pharm Bull 52: 1246–1248.
- Wilkinson JA, Brown AMG (1999): Horse chestnut - Aesculus hippocastanum: Potential applications in cosmetic skin-care products. Int J Cosmetic Sci 21: 437–447.
- Yang X, Zhao J, Cui Y, Liu X, Ma C, Hattori M, Zhaung L (1999a): Anti HIV-1 protease triterpenoid saponins from the seeds of Aesculus chinensis. J Nat Prod 62: 1510–1513.
- Yang XW, Zhao J, Cui YX (1999b): Three new triterpenoid saponins from seeds of Aesculus chinensis. Chin Chem Lett 10: 1031–1034.
- Yoshikawa M, Murakami T, Otuki K, Yamahara J, Matsuda H (1999): Bioactive saponins and glycosides. XIII. Horse chestnut. (3): Quantitative analysis of aescins Ia, Ib, IIa, and IIb by means of high performance liquid chromatography. Yakugaku Zasshi 11: 81–87.
- Zdravković-Korać S, Ćalić D, Druart PH, Radojević LH (2003): The horse chestnut lines harboring the rol genes. Biol Plant 47: 487–491.
- Zdravković-Korać S, Muhovski Y, Druart Ph, Ćalić D, Radojević Lj (2004): Agrobacterium rhizogenes-mediated DNA transfer to Aesculus hippocastanum L. and the regeneration of transformed plants. Plant Cell Rep 22: 698–704.