Abstract
In the present study, the anxiolytic and sedative-hypnotic activities of polygalasaponins extracted from Polygala tenuifolia Willdenow (Polygalaceae) were determined in mice using hole-board, elevated plus maze, open field, and sodium pentobarbital-induced hypnosis tests. Moreover, the acute toxicity of polygalasaponins was also estimated in mice. Sixty minutes after p.o. administration of polygalasaponins (40, 80, 160 mg/kg) in mice, the central crossing counts and percentage of central/total ambulation significantly increased and the number of rearings and defecations was evidently inhibited in the open field test. Polygalasaponins also increased the head-dips of mice in the hole-board test and the time spent by mice in the open arms of the X-maze, prolonged sleep duration and shortened sleep latency in the test of synergetic effect on sodium pentobarbital (45 and 25 mg/kg, respectively). Acute toxic study showed the oral median lethal dose (LD50) of polygalasaponins was 3.95 g/kg and 0% lethal dose 2.6 g/kg. These results suggest that polygalasaponin possesses evident anxiolytic and sedative-hypnotic activities and has a relatively safe dose range, which supports the use of Polygala tenuifolia root as an anxiolytic and sedative-hypnotic drug in folk medicine.
Introduction
In western countries, many sedatives, anxiolytics, and hypnotics have been created. The therapeutic value of these agents is well established, and they continue to play an important role in managing many anxiety symptoms in the context of both psychiatric disorders and medical illness. However, the toxic effects of these drugs have also been established, including various withdrawal syndromes, dependence, tolerance, and impairment of cognitive function, memory, and general daytime performance (CitationThomas & Christopher, 2004). On the other hand, there are numerous herbal medicines exerting good anxiolytic, sedative, and hypnotic effects with few side effects in Asian countries, especially in China, Japan, and Korea, such as Fructus schisandrae Chinensis (Magnoliaceae) (CitationHuang et al., 2007), Rhodiola sachalinensis A. Bor. (Crassuaceae) (CitationLi et al., 2007), Acorus gramineus Schott. (Acoraceae) (CitationWu, 2007; CitationZhang et al., 2007), Polygala tenuifolia Willdenow (Polygalaceae) (CitationShin et al., 2004), and so on.
Radix polygalae (RP), the root of Polygala tenuifolia, has extensively been used as a traditional Chinese medicine in clinics with the actions of tranquilizing the mind, eliminating phlegm for resuscitation, and dissipating carbuncles. Accumulating evidence shows that RP has sedative, antipsychotic, cognitive improving/neuroprotective, and anti-inflammatory therapeutic effects on the central nervous system (CitationChung et al., 1992, Citation2002; CitationJang et al., 1997; CitationKim et al., 1998; CitationZhang et al., 2008). The ethanol extract of Polygala tenuifolia root can evidently attenuate cocaine-induced locomotor activity and conditioned place preference in mice (CitationShin et al., 2004), which indicates that RP contains active ingredients having potential antipsychotic, sedative, and hypnotic actions. However, it is not exactly known which compounds possess these activities.
Polygalasaponins, one of the main constituents of RP, could improve learning and memory abilities of dementia-like rats induced by combined injection of α-amyloid peptide and ibotenic acid into the right nucleus basalis magnocellularis (CitationChen et al., 2002), increase total protein and total antioxidant capacity in the brain tissues of senescence-accelerated mice, and decrease acetylcholinesterase activity in the brain and interleukin-2 level in their blood serum (CitationTian et al., 2004). Polygalasaponins also ameliorates learning and memory in aged and dysmnesic mice, which is associated with increases of norepinephrine (NE) and dopamine (DA) levels in the hippocampus and a decrease of acetylcholine esterase (AChE) activity in the cortex of mice (CitationZhang et al., 2008). As far as we are aware, however, no reports have been issued on the anxiolytic and sedative-hypnotic effects of polygalasaponins in mice or an acute toxicity study.
In the present study we investigated neurobehavioral effects of polygalasaponins in mice using hole-board, elevated plus maze, open field, and sodium pentobarbital-induced hypnosis tests. Additionally, the acute toxicity of polygalasaponins in mice was also estimated. The aim of the present study was to assess whether polygalasaponins from RP possesses anxiolytic and sedative-hypnotic activities.
Materials and methods
Plant material and extraction
Radix polygalae (RP) was purchased from Shanghai Tong-ren-tang Pharmaceutical Group, and identified as the root of Polygala tenuifolia by H.-C. Zheng, a pharmacognosist, from the School of Pharmacy of the Second Military Medical University (Shanghai, China). A voucher specimen was deposited with the number SY3218 in the Department of Pharmacognosy, School of Pharmacy, Second Military Medical University. Polygalasaponins, the mixture of saponins with similar structure, was purified in accordance with the procedure of CitationZhang et al. (2008) and appeared as yellow-brown powder. Nearly all of polygalasaponins can be converted into tenuifolin, which is therefore often used as a chemical reference substance for the quality control of polygalasaponins (CitationDong et al., 2007). The content of tenuifolin in polygalasaponins was determined after alkaline hydrolysis by high performance liquid chromatography (HPLC) (CitationDong et al., 2007) and the average content was 83.68% (RSD = 1.08%, n = 5). The HPLC chromatograms of samples are shown in to .
Animals
Male KM mice (20-24g), obtained from the Experimental Animal Center of the Second Military Medical University (Shanghai, China), were housed in a regulated environment (20° ± 2°C), with a 12 h light/dark cycle (08:00-20:00, light). Food and water were given ad libitum, except for the duration of the experimental period. All animal treatments were strictly in accordance with international ethical guidelines concerning the care and use of laboratory animals, and the experiments were carried out under the approval of the Committee of Experimental Animal Administration of the University.
Treatment
Polygalasaponins were dissolved in distilled water prior to administration. Four groups of animals (n = 10) were orally treated with 40, 80, and 160 mg/kg polygalasaponins and 2 mg/kg diazepam, respectively, by intubation. Another group of animals (control group, n = 10) was given distilled water, and it was run concurrently with the polygalasaponin- and diazepam-treated groups, all of which were given in a volume of 10 mL/kg body weight irrespective of dose. Sixty minutes after oral administration, polygalasaponins and diazepam were evaluated.
Drugs
The following drugs were used: sodium pentobarbital (purity 99%; Shanghai Chemical Reagent Corporation, China), diazepam (purity 99%; Shanxi Linfen Pharmaceutical Factory, China).
Open field test
To study the effects of polygalasaponins on locomotion activity, 50 mice were used. The apparatus was made of vinyl chloride 60 cm in length, 60 cm in width, and 25 cm in height. The white floor was a removable tray, which was divided into 36 squares (10 × 10 cm). Sixteen squares were defined as the center and the others adjacent to the walls as the periphery. A 60 W white bulb illuminated the apparatus. One hour after oral administration the mouse was gently placed at the center of the open field and then the amount of locomotion was measured for 2 min. The mouse was taken out of the apparatus after 2 min and the floor was cleaned with water. The following parameters were recorded for 2 min: total locomotion (a mouse removed all four paws from one square and entered another), the locomotion in the central area. The times of rearing (a mouse raised both front paws from the floor) and defecation for 2 min were also counted (CitationPaulus et al., 1999; CitationWang et al., 2003; CitationHuang et al., 2007).
Hole-board test
The hole-board apparatus was made of a wooden floorboard 40 cm square and 1 cm thick, enclosed by four walls 25 cm high. Sixteen holes with a diameter of 3 cm each were distributed evenly on the floorboard. The apparatus was elevated to a height of 40 cm so that the mouse poking its nose into the hole did not see the bottom. Testing took place under the indirect illumination of a single upturned angle-poise lamp. One hour after the administration of polygalasaponins, the mice were individually placed in the center of a perforated board. A head-dip was scored if both eyes disappeared into the hole. The number of explored holes was recorded for 5 min (CitationXu et al., 2002).
Elevated plus-maze test
One hour before the experiment the mice were brought to the testing room. The elevated plus-maze apparatus was made of wood blocks and consisted of four arms (25 cm long × 5 cm wide) fixed to a central platform (5 cm × 5 cm). Two opposite arms had 15 cm high walls (closed-arms) and another two had no border in place of the walls (open-arms). The maze was elevated to a height of 40 cm. For testing, the mice were placed individually in the center of the maze with their head facing an open arm. Between two mice, the apparatus was wiped clean with absorbent cotton. The number of entries and the time spent in each arm were measured during the 5 min session. These measures enabled the computation of the number of entries to open and closed arms and the time spent in open- and closed arms, respectively. The number of entries and time on the open arms was expressed as a percentage of the total number of entries to all arms or of the time spent in all arms, respectively (CitationTokumo et al., 2006).
Sodium pentobarbital hypnosis test
To determine sedative and hypnotic activities of drugs, polygalasaponins and diazepam were administered orally 60 min before the test. Following the pentobarbital injection (45 mg/kg pentobarbital was used as a hypnotic dosage and 25 mg/kg as a sub-hypnotic dosage), each mouse was observed for sleep latency (time from the injection of pentobarbital to sleep onset) and sleep duration (the criterion for sleep is defined as the loss of righting reflex). The time between loss and recovery of the righting reflex was recorded as sleeping time (CitationGonzález-Trujano et al., 1998; CitationXu et al., 2002; CitationAguirre-Hernández et al., 2007).
Acute toxicity study (LD50 estimation)
This method estimated the dose of the polygalasaponins that killed 50% of a reduced sample of animals by a given route. In the first phase, a series of doses of polygalasaponins were administered orally to mice. Each dose was tested in three mice each time and three mice of either sex (selected randomly) were included. Lethal doses of 0% and 100% were estimated as 2.6 and 5.5 g/kg, respectively, and recorded. Then five different doses of polygalasaponins were established according to the following formula.
where R is the grouping coefficient; n is the number of divided groups (n = 1, 2, 3, 4, 5); a is 0% lethal dose; b is 100% lethal dose.
Five different doses were divided, which were 2.6, 3.14, 3.78, 4.56 and 5.5 g/kg, respectively. Five groups of animals (half male and half female, n = 14) were orally treated with the above five doses respectively. Daily cage-side observations were made till the end of the 14-day observation period, post exposure. If any dead mouse was found it was immediately removed from the cage and the number of dead mice was registered.
Statistical analysis
The data were analyzed using a statistical package (SPSS 10.0). The data for multiple comparisons were performed by one-way ANOVA followed by LSD t-test. P <0.05 was considered statistically significant and all results are presented as mean ± SEM.
Results
Effects of polygalasaponins on spontaneous behavior of mice in the open field test
The spontaneous behavior in mice was observed in the open field and the results are shown in . Sixty minutes after oral administration, the medium and higher doses of polygalasaponins (80, 160 mg/kg) significantly increased the number of central locomotions and the ratio of central to total, and decreased the counts of rearing and defecation compared with the control group of mice. However, the lower dose of polygalasaponins (40 mg/kg) did not show similar activity.
Table 1. Effects of polygalasaponins on spontaneous behavior of mice in the open field test.
Effects of polygalasaponins on head-dips of mice in the hole-board test
Diazepam (2 mg/kg) markedly increased the number of head-dips of mice compared with the control group, as shown in . In contrast to the control group, the head-dips of mice given 80, 160 mg/kg doses of polygalasaponins also evidently increased except for the lower dose of polygalasaponins (40 mg/kg).
Effects of polygalasaponins on anxiety behavior of mice in the elevated plus maze test
As shown in , 60 min after oral administration of polygalasaponins (80, 160 mg/kg), the time spent in the open arms was noticeably prolonged in mice compared with the control group, but the number of entries to the open arms did not change (). Diazepam (2 mg/kg) also significantly increased the number of entries and the time spent in the open arms compared with the control group.
Figure 5. Effects of polygalasaponins on anxiety behavior of mice in the elevated plus maze test. Values are mean ± SEM, n = 10. *P <0.05, **P <0.01, significance versus control. (A) Effects of polygalasaponins on time on the open arms in mice; (B) Effects of polygalasaponins on entries to the open arms in mice.
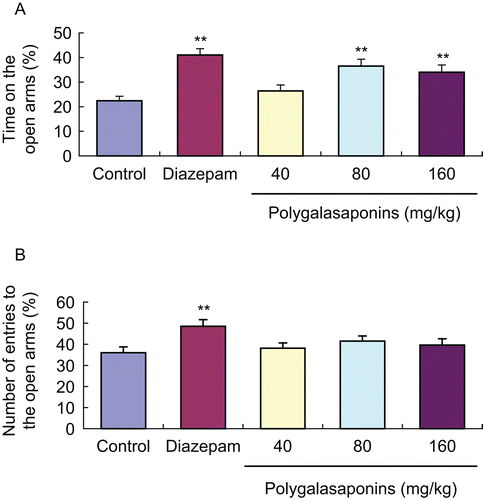
Effects of polygalasaponins on pentobarbital-induced hypnosis in mice
Effects of pre-treatment with polygalasaponins on sleep latency and sleep duration induced by sodium pentobarbital (25 and 45 mg/kg, i.p.) are shown in and . These results showed that polygalasaponins (40, 80, 160 mg/kg) significantly prolonged sleep duration in mice in a dose-dependent manner, but did not evidently decrease sleep latency compared with the control group of mice. Sub-hypnotic dose of pentobarbital (25 mg/kg, i.p.) did not cause the loss of righting reflex in control mice, whereas the medium and higher doses of polygalasaponins (80, 160 mg/kg) markedly shortened latency to persistent sleep and prolonged sleep duration compared with the control group. Diazepam (2 mg/kg) evidently decreased sleep latency and increased sleep duration induced by pentobarbital.
Table 2. Effects of polygalasaponins on the onset and duration of sleep in mice induced by pentobarbital.
Table 3. Effects of polygalasaponins on the onset and duration of sleep in mice induced by sub-hypnotic dose of pentobarbital.
Acute toxicity study (LD50)
In the first phase test, 0% and 100% lethal doses of polygalasaponins were respectively estimated as 2.6 and 5.5 g/kg. Then five doses were determined according to the given formula, which were 2.6, 3.14, 3.78, 4.56, and 5.5 g/kg. After oral administration of the above doses of polygalasaponins, a marked reduction in spontaneous activity of mice was respectively observed in each dose group. Regression analysis revealed the LD50 of polygalasaponins was 3.95 g/kg, 95% confidence limits 3.62-4.33 g/kg ().
Table 4. Effects of polygalasaponins on the determination of the median lethal dose p.o. (LD50).
Discussion
In this study, we investigated the anxiolytic and sedative-hypnotic effects of polygalasaponins and its acute toxicity in mice. The open field test is designed to examine responses of mice or rats to a new and unfamiliar environment (novel environment). A novel environment causes mice or rats to move around to explore the environment and increase locomotion in consequence. On the other hand, mice or rats tend to avoid brightly illuminated, novel, open spaces. Therefore, the novel environment acts as an anxiogenic stimulus and causes intense anxiety and fear in mice or rats, which exhibit rearing, grooming, defecation, locomotion, and so on. These parameters are often applied by an open field test as a measure of anxiety and fear. The inhibition in ambulating, rearing and preening behavior suggests that the drug has a sedative activity. The locomotion in the central area is more sensitive than the total (CitationPaulus et al., 1999; CitationWang et al., 2003; CitationHuang et al., 2007), which was proved in this study.
Among five groups of animals, total ambulation number had no difference, but polygalasaponins and diazepam groups were evidently different from the control group in central ambulation number. According to our results, diazepam as a sedative drug did not influence the total crossing counts, but significantly increased the central crossing counts and percent of central/total ambulation in mice, and inhibited the other parameters. Polygalasaponins also showed a similar activity. These findings suggest that polygalasaponins, similar to diazepam, could alleviate intense anxiety and fear in mice in open field, so their central crossing counts evidently increased.
In order to further verify the sedative and anxiolytic activities of polygalasaponins, another two models were also used. The hole-board apparatus offers a simple method for measuring the responses of mice to a novel environment and has gained popularity as a model of anxiety used for assessing drug effects. The reliability of the hole-board for testing naive mice was established by many other investigators (CitationFile & Wardill, 1975; CitationTakeda et al., 1998). The elevated plus-maze is used to determine the rodent’s unconditioned response to a potentially dangerous environment. Curiosity leads the rodent to spend more time in the open arms while anxiety may make them stay in the closed, protected arms of the model (CitationLister, 1987; CitationHogg, 1996; CitationFile, 2001; CitationCrawley, 1999). The plus-maze is a validated and reliable test for detecting both anxiolytic- and anxiogenic-like effects of agents (CitationPellow & File, 1986; CitationRodgers & Cole, 1994; CitationHogg, 1996). An increase of the proportion of the entries and the time spent in the open arms without a changed locomotor activity is considered as a powerful marker for an “anxiolytic” substance effect (CitationPellow et al., 1985).
In the hole-board experiment, the medium and higher doses of polygalasaponins evidently increased the number of head-dips in mice. In the elevated plus-maze test, polygalasaponins increased the time spent in the open arms at the doses of 80 and 160 mg/kg, but did not change the number of entries to the open arms. Additionally, polygalasaponins did not change total counts and time of entries to all arms in comparison to control animals. These results reveal the anxiolytic activity of polygalasaponins. Similar to previous reports (CitationTakeda et al., 1998; CitationNaderi et al., 2008), diazepam also evidently increased the number of head-dips in the hole-board test, and the entries and time on the open arms significantly increased. It was also reported that the higher dose of diazepam suppressed the counts of head-dips in mice (CitationAguirre-Hernández et al., 2007), which was associated with a decrease of total locomotor activity in mice. But 2 mg/kg dose of diazepam and 40, 80, 160 mg/kg doses of polygalasaponins did not evidently decrease total locomotion activity of mice in our study.
The test of pentobarbital-induced sleep is a well known experimental approach to screening hypnotics. In the present study, polygalasaponins significantly prolonged the sleep duration induced by pentobarbital (45 mg/kg, i.p.) in a dose-dependent manner, but did not evidently shorten sleep latency of mice. Generally, the test is considered to be unspecific, since the drugs that inhibit liver metabolism of pentobarbital also prolong sleeping time of mice. So we applied another experimental method i.e. sub-hypnotic test induced by pentobarbital, in which the drugs only inhibiting the activity of metabolic enzymes in the liver do not increase pentobarbital-induced sleep duration and latency (CitationXu et al., 2002). The results indicate that polygalasaponins not only evidently prolonged the sleep duration induced by the sub-hypnotic dose of pentobarbital (25 mg/kg, i.p.), but also decreased the latency of falling asleep and increased the rate of sleep onset.
Although in the experiments mentioned above, polygalasaponins evidently revealed anxiolytic and sedative-hypnotic effects, it was not eliminated that these effects were induced by its toxicity on central nervous system in mice. Therefore, it is very important to assess its acute toxicity. In the present study, the LD50 of oral polygalasaponins was 3.95 g/kg, which was far from the effective dose of 160 mg/kg. 0% lethal dose of 2.6 g/kg suggests that anxiolytic and sedative-hypnotic effects of polygalasaponins in mice have nothing to do with central nervous toxicity and have a relatively safe dose range.
Conclusions
Polygalasaponins, extracted from the root of Polygala tenuifolia, increases the central crossing counts and percent of central/total ambulation, and inhibits the number of rearing and defecation in mice in the open field test. The head-dips in the hole-board test and the time spent in the open arms of the X-maze are increased. Polygalasaponins also evidently prolongs the sleep duration and shortens the sleep latency induced by pentobarbital. Acute toxic study identifies the security margin between toxicity and proved efficacy of polygalasaponins. These findings suggest that polygalasaponins possesses evident anxiolytic and sedative-hypnotic activities and has a relatively safe range of dose, which support the use of the root of Polygala tenuifolia as an anxiolytic and sedative-hypnotic drug in folk medicine. It may be a regret that the mechanism is not clear on anxiolytic and sedative-hypnotic activities of polygalasaponins, which needs further study.
Declaration of interest
The authors report no conflicts of interest. The authors alone are responsible for the content and writing of the paper.
References
- Aguirre-Hernández E, Martínez AL, González-Trujano ME, Morenoa J, Vibrans H, Soto-Hernández M (2007): Pharmacological evaluation of the anxiolytic and sedative effects of Tilia americana L. var. mexicana in mice. J Ethnopharmacol 109: 140–145.
- Chen Q, Cao YG, Zhang CH (2002): Effect of tenuigend on cholinergic decline induced by β-amyloid peptide and ibotenic acid in rats. Acta Pharm Sin 37: 913–917.
- Chung IW, Kim SR, Kim EG (1992): Dopamine-2 and serotonine-2 receptor bindings in antipsychotic medicines from natural products. J Korean Neuropsychi Assoc 31: 856–867.
- Chung IW, Moore NA, Oh WK, O’Neill MF, Ahn JS, Park JB, Kang UG, Kim YS (2002): Behavioural pharmacology of polygalasaponins indicates potential antipsychotic efficacy. Pharmacol Biochem Behav 71: 191–195.
- Crawley JN (1999): Behavioral phenotyping of transgenic and knockout mice: experimental design and evaluation of general health, sensory functions, motor abilities and specific behavioral tests. Brain Res 835: 18–26.
- Dong XB, Li J, Jiang Y, Zheng Y, Dong TXT, Tu PF, Tsim WKK (2007): Determination of tenuifolin in Tianwang Buxinwan and Guipiwan by HPLC. China J Chin Mater Med 32: 1647–1649.
- File SE, Wardill AG (1975): The reliability of the hole-board apparatus. Psychopharmacology (Berl.) 44: 47- 51.
- File SE (2001): Factors controlling measures of anxiety and responses to novelty in the mouse. Behav Brain Res 125: 151–157.
- González-Trujano ME, Navarrete CA, Reyes B, Hong E (1998): Some pharmacological effects of the ethanol extract of leaves of Annona diversifolia on the central nervous system in mice. Phytotherapy Res 2: 600–602.
- Hogg S (1996): A review of the validity and variability of the elevated plusmaze as an animal model of anxiety. Pharmacol Biochem Behav 54: 21–30.
- Huang F, Xiong YT, Xu LH, Ma SP, Dou CG (2007): Sedative and hypnotic activities of the ethanol fraction from Fructus schisandrae in mice and rats. J Ethnopharmacol 110: 471–475.
- Jang KJ, Lee KH, Kim SL, Choi DY, Park BK, Im DH, Cho YJ, Jhoo WK, Kim HC (1997): Chongmyungtang attenuates kainic acid-induced seizure and mortal effect in the mouse. Arch Pharmacol Res 20: 375–378.
- Kim HM, Lee EH, Na HJ, Lee SB, Shin TY, Lyu YS, Kim NS, Nomura S (1998): Effects of Polygala tenuifolia root extract on the tumor necrosis factor-1 secretion from mouse astrocytes. J Ethnopharmacol 61: 201–208.
- Li T, Xu G, Wu L, Sun C (2007): Pharmacological studies on the sedative and hypnotic effect of salidroside from the Chinese medicinal plant Rhodiola sachalinensis. Phytomedicine 14: 601–604.
- Lister RG (1987): The use of a plus-maze to measure anxiety in the mouse. Psychopharmacology (Berl.) 92: 180–185.
- Naderi N, Haghparast A, Saber-Tehrani A, Rezaii N, Alizadeh AM, Khani A, Motamedi F (2008): Interaction between cannabinoid compounds and diazepam on anxiety-like behaviour of mice. Pharmacol Biochem Behav 89: 64–75.
- Paulus M, Dulawa S, Ralph R, Mark AG (1999): Behavioral organization is independent of locomotor activity in 129 and C57 mouse strains. Brain Res 835: 27–36.
- Pellow S, Chopin P, File SE, Briley M (1985): Validation of open: Closed arm entries in an elevated plus-maze as a measure of anxiety in the rat. J Neurosci Methods 14: 149–167.
- Pellow S, File SE (1986): Anxiolytic and anxiogenic drug effects on exploratory activity in an elevated plus-maze: A novel test of anxiety in the rat. Pharmacol Biochem Behav 24: 525–529.
- Rodgers RJ, Cole JC (1994): The elevated plus-maze: Pharmacology, methodology and ethology, in: Cooper SJ, & Hendrie CA, eds, Ethology and Psychopharmacology. London, Wiley, pp. 9–44.
- Shin EJ, Oh KW, Kim KW, Kwon YS, Jhoo JH, Jhoo WK, Cha JY, Lim YK, Kim IS, Kim HC (2004): Attenuation of cocaine-induced conditioned place preference by Polygala tenuifolia root extract. Life Sci 75: 2751–2764.
- Takeda H, Tsuji M, Matsumiya T (1998): Changes in head-dipping behavior in the hole-board test reflect the anxiogenic and/or anxiolytic state in mice. Eur J Pharmacol 350: 21–29.
- Thomas R, Christopher D (2004): Evolution of insomnia: Current status and future direction. Sleep Med 5: S23-S30.
- Tian F, Jiang Y, Zhang K, Liu XW, Zhang ZY (2004): The effect and mechanism of Polygala tenuifolia glycoside on SAM-P/8. Geriatr Health Care 10:13–19.
- Tokumo K, Tamura N, Hirai T, Nishio H (2006): Effects of (Z)-3-hexenol, a major component of green odor, on anxiety-related behavior of the mouse in an elevated plus-maze test and biogenic amines and their metabolites in the brain. Behav Brain Res 166: 247–252.
- Wang YQ, Zhu CY, Wei EQ (2003): A new model for evaluating locomotor activity and central depressive drugs in different strain mice. Bull Sci Technol 19: 292–296.
- Wu JW (2007): Clinical observation on the effects of Chinese herbal drugs and acupuncture plus traditional Chinese herbal medicine in treatment of 295 patients with insomnia. J Chin Integr Med 5: 592–593.
- Xu SY, Bian RL, Chen X (2002): Experimental Methodology of Pharmacology, third edition. Beijing, Peoples’ Medical Publishing House, pp. 802–805.
- Zhang H, Han T, Zhang L, Yu CH, Wan DG, Rahmanc K, Qin LP, Peng C (2008): Effects of tenuifolin extracted from radix polygalae on learning and memory: A behavioral and biochemical study on aged and amnesic mice. Phytomedicine 15: 587–594.
- Zhang H, Han T, Yu CH, Rahman K, Qin LP, Peng C (2007): Ameliorating effects of essential oil from Acori graminei rhizoma on learning and memory in aged rats and mice. J Pharm Pharmacol 59: 301–309.