Abstract
Earthworm fibrinolytic enzymes (EFEs), an ideal drug for cardiovascular diseases, have a very low oral bioavailability. In order to improve the absorption of EFEs, six different enhancers were selected to increase the intestinal absorption of EFEs. In vitro (Caco-2 monolayers) and in vivo (mice) experiments were carried out to find the optimum concentration and action time of these enhancers for EFE absorption. We found that EFEs could be transported into blood across intestinal endothelial membrane after administration via intragastric administration with low bioavailability. These results obtained from in vitro experiments were similar to those in vivo. Moreover, menthol and glucose showed absorption enhancement properties with a relatively low cytotoxicity.
Introduction
Earthworm fibrinolytic enzyme (EFE) is a water-soluble protein isolated and purified from earthworms (Eisenia fetida (Lumbricidae)), with low membrane permeability. The enzyme possesses strong fibrinolytic and thrombolytic activities in vivo, broad pH optima (pH 4-10). These features are effective and useful for EFEs to treat cardiovascular diseases. Oral drug delivery is considered the preferred route of administration because of its non-invasive nature. It reduces the risk of infection and does not require trained personnel. However, hydrophilic drugs, which are difficult to absorb by enterocytes unless they are transported by carrier proteins across the apical membrane of enterocytes, usually present low bioavailability (<1%) after oral administration. One cause of the low bioavailability is their poor intestinal permeation through the paracellular pathway restricted by the tight junctions at the apical side of the enterocytes (CitationCano-Cebrian et al., 2005). In recent years great efforts have been made to find agents that can modulate tight junctions and magnify the paracellular permeability of hydrophilic compounds without causing significant intestinal damage. It was postulated that the transient and reversible opening of the tight junctions with permeation enhancers could increase the permeation and intestinal absorption of therapeutic macromolecules (CitationCano-Cebrian et al., 2005; CitationRoss & Toth, 2005).
Recently, an in vitro method of using human colon carcinoma Caco-2 cells to estimate in vivo drug transport and metabolism as well as efficacy of absorption enhancers had emerged as a leading method to test potential drugs in the early development stages. Although Caco-2 cells lack some protecting properties of the intestinal tissue (e.g., mucus, dilution effects), correspondence of in vitro and in vivo data were reported by several authors (CitationAnderberg et al., 1992; CitationFricker et al., 1996).
The aim of our investigation was to evaluate the transport characteristics of the six different absorption enhancers on the hydrophilic FITC-labeled protein EFEs. The experiments were carried out on Caco-2 cells and mice. We attempted to find several safe and effective absorption enhancers to stimulate the absorption of EFEs.
Materials and methods
Chemicals, reagents and drugs
EFEs were isolated by Nanjing Agricultural University, P.R. China. The fluorescence markers (FITC, FD70s, and FD2000s), glucose, EDTA, sodium cholate, menthol, SDS, and MTT were purchased from Sigma (St. Louis, MO).
Cell culture
Caco-2 cells were obtained from the American Type Culture Collection (ATCC, Rockville, MD). Caco-2 cells (passages 45-65), maintained in Dulbecco’s modified Eagle’s medium (DMEM) supplemented with 10% fetal bovine serum, were incubated at 37°C in a humidified atmosphere of 5% CO2 in air. The confluent monolayers of Caco-2 cells were trypsinized, washed and resuspended, and then seeded on the HA Millicell filter inserts (Millipore, Bedford, MA) at a seeding density of 104 cells/cm2. Cells reached confluency in 7-8 days and were used for experimentation between days 14 and 21. The medium was changed every other day.
Evaluation of cytotoxicity of enhancers
The acute cytotoxic effects of the six enhancers on cell viability were measured by the MTT colorimetric assay. Caco-2 cells, seeded in 96-well culture plates, were washed three times with PBS. Subsequently, different absorption enhancers (glucose 750 mmol/L, EDTA 2.5 mmol/L, sodium cholate 10 mmol/L, SDS 0.2 mmol/L, ethanol 10%, menthol 1%) were added to each well. Then Caco-2 cells were incubated at 37°C for 2, 6, and 24 h. Thereafter, cell viability was determined by the MTT test according to the procedure described by CitationMosmann (1983). Briefly, 20 μL of MTT solution at 5 mg/mL in DMEM were added to each well and incubated at 37°C for 3 h. After medium was removed, 100 mL of acid-isopropanol (0.04 N HCl in isopropanol) and 150 μL of DMSO were added to each well and mixed thoroughly to completely dissolve the dark blue crystals. Then the plates were measured with a BioTek FL600-fluorescence/absorbance plate reader. Cell viability was expressed as the percentage of control. The same plate contained additional wells with media and chemical only (without cells), processed in parallel as reference blanks.
The integrity and tightness of the Caco-2 cell monolayers
We determined the integrity and tightness of the monolayers by measuring transepithelial passage of three different probes. As paracellular markers, fluorescein isothiocyanate (FITC, 389.4 Da) and fluorescein-isothiocyanate dextran of molecular masses of 70 kDa and 2000 kDa (FD70 and FD2000), were unable to permeate the cellular membrane at physiologic pH due to its hydrophilicity. These probes had to be transported exclusively via the paracellular route across intestinal epithelia. The Caco-2 cells were cultured for 21 days on the Millicell filters until formation of confluent monolayers. FITC (1 mg/mL), FD4 (1 mg/mL), or FD70 (1 mg/mL) was added to the apical compartment of the Millicell filters. Then the cultures were placed on a rotary shaker in a 5% CO2 incubator at 37°C to ensure adequate mixing. The permeation was determined over a 120-min period. The permeability of these probes was expressed as the percentage ratio of the concentration in the basolateral chamber versus that in the apical chamber. Samples (100 μL) of the apical and basolateral chambers were diluted in 1 mL water and analyzed for fluorescence with a Bio-tTek fluorescence spectrophotometer (excitation wavelength 495 nm, emission wavelength 525 nm). All measurements were determined in triplicate and expressed as means ± SD.
In vitro transport studies with Caco-2 cell monolayers
Transepithelial transport was studied with cells grown on Millicell filters for 21 days. The culture medium of apical and basolateral compartment were removed and washed three times with HBSS at 37°C. At time 0, six different enhancers (glucose 750 mmol/L, EDTA 2.5 mmol/L, sodium cholate 10 mmol/L, SDS 0.2 mmol/L, ethanol 10%, menthol 1%) were added to the apical (0.5 mL) compartment of the insert individually. The monolayers were incubated at 37°C in 5% CO2 for 2 h. After the monolayers were washed three times with HBSS, FITC-labeled EFEs were added into the upper compartment of Millicell filters and the flux across Caco-2 cell monolayers was determined. At each sampling time (30 min, 2 h, and 24 h), an aliquot of transport medium was withdrawn from the acceptor compartment and replaced by an equal volume of fresh medium. The amount of FITC-labeled EFEs transported across the Caco-2 cell monolayers were detected by counting the samples in a Bio-Tek fluorescence spectrophotometer.
Intragastric administration
In order to investigate the in vivo effect of the six different absorption enhancers, a study on mice was performed. All animal experiments were performed according to the guidelines of the local authorities. After fasting for 24 h, six mice (each group) were intragastrically administrated with FITC-labeled EFEs containing enhancers individually (glucose 750 mmol/L, EDTA 2.5 mmol/L, sodium cholate 10 mmol/L, SDS 0.2 mmol/L, ethanol 10%, menthol 1%). The control mice were administered saline. At 0, 2, 6, and 24 h, blood samples of mice were collected by globe enucleation and serum samples were obtained by centrifugation at 10,000 g and 4°C for 10 min. The samples were counted by Bio-Tek fluorescence spectrophotometer.
Safety evaluation
The damage to the intestinal mucosa (duodenum) was investigated after administration of the different enhancers at double-dose concentration (glucose 1500 mmol/L, EDTA 5 mmol/L, sodium cholate 20 mmol/L, SDS 0.4 mmol/L, ethanol 20%, menthol 2%). The mice of the control group were given 0.25 mL saline. The mice were sacrificed 24 h after administration. Intestinal tissues were removed and fixed in Bouin’s liquid. The fixed samples were embedded in paraffin and cut into 4 μm semi-serial sections. Hematoxylin-eosin (HE) stainings were applied (CitationZhang et al., 2007).
Results
Effects of enhancers on cell viability
The aim of these studies was to determine the suitable concentrations and action time of the six enhancers. Cytotoxicity was evaluated by measuring mitochondrial dehydrogenase (MDH) activity based on MTT assay on confluent Caco-2 cells in 96-well plates (CitationLiu et al., 1999). shows cytotoxic effects of the six different enhancers on Caco-2 cells. We demonstrated that enhancers at a certain concentration had cytotoxic effects in a time-dependent manner. The six enhancers induced no significant mitochondrial toxicity towards Caco-2 cells at 2 h (more than 95% cell viability). These results suggested that the enhancers did not induce a subsequent decrease in cell viability. A 6 h exposure to some enhancers led to a marked decrease in cell viability: menthol (74.6%), sodium cholate (68.4%), glucose (78.7%), whereas EDTA, SDS, and ethanol were still safe to the cell monolayers. However, a 24 h exposure to the enhancers, except for ethanol, all exhibited a more drastic decrease in cell viability.
Figure 1. Cytotoxic effects of the six enhancers on Caco-2 cell monolayers. Cytotoxicity was measured by the MTT assay following 2, 6, and 24 h incubation at 37°C, 5% CO2 and 95% relative humidity. For the MTT assay, PBS and DMSO were used as positive (100% cell viability) and negative (0% cell viability) controls, respectively. All measurements were expressed as mean ± SD.
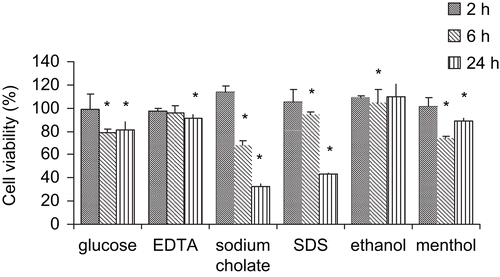
Integrity of the Caco-2 cell monolayers
The integrity and tightness of the monolayers were determined by measuring the permeability of paracellular markers at different graded mass: FITC, FD70, FD2, 000. If the cell monolayers were disrupted, the fluorescence markers (FITC, FD70s, and FD2000s) would transport the Caco-2 cell monolayers with large amount. The experiment demonstrated that the FD70 (0.12%) and FD2000 (0.18%) almost could not transport through the Caco-2 cell monolayers. So the results suggested that the cell monolayers were intact integrity.
Transport studies in vitro
EFEs, mostly absorbed by passive diffusion through the paracellular route, were added to the apical (AP) compartment. Permeability of EFEs across Caco-2 monolayers was examined in the apical-to-basolateral (absorptive) direction. There was a linear relationship between time and the amount of EFEs absorption (). We demonstrated that in the control group, the absorption of EFEs was 6.6% ± 1.7% of the initial dose after 2 h. The ratio reached 9.82% ± 1.21% after 24 h.
Figure 2. Cumulative permeation of EFEs across the Caco-2 cell monolayers. Six different enhancers were added to the apical compartment of the insert individually to increase the EFEs permeation.
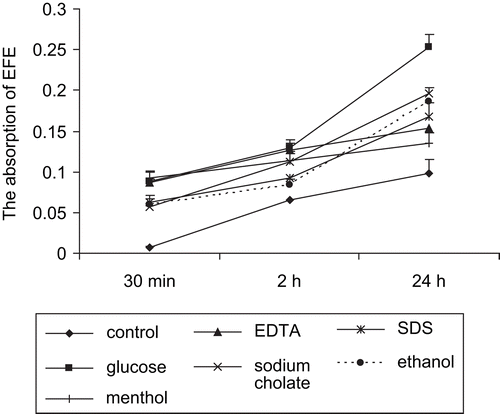
In order to determine the effect of different enhancers on the contribution of paracellular transport of EFEs, the apical side of Caco-2 cell monolayers was exposed to different enhancers for different periods, from 0.5 to 24 h. Results indicated that the permeability of EFEs was increasing gradually over time. When Caco-2 monolayers were incubated with enhancers for 2 h, the absorption of EFEs (%) were: glucose (12.87% ± 1%), EDTA (12.71% ± 0.74%), menthol (11.42% ± 0.7%), sodium cholate (11.26% ± 1.21%), SDS (9.17% ± 1.74%), ethanol (8.37% ± 0.74%). These results showed that enhancers clearly enhanced the permeability of EFEs across intestinal Caco-2 cell monolayers. Among the six different enhancers, glucose expressed the most obvious improvement effect.
Transport studies in mice
Although we demonstrated that EFEs could be transported by paracellular route on Caco-2 cell monolayers, it was necessary to investigate whether the enzymes could be absorbed into blood in vivo. After absorption, proteins might be metabolized and excreted out the body soon. In our experiments, FITC-labeled EFEs and enhancers were simultaneously intragastrically administrated to mice. After 2 h, the absorption of EFEs into blood reached the peak in all groups (). In the glucose group, the absorption was 29.25% ± 3.44% of the total injection, nearly three times higher than the control group (10.5% ± 1.98%). Later, EFEs were detected decreasingly with the lapse of time. The sequence of enhanced efficiencies of different enhancers in vivo was as follows: glucose > EDTA > sodium cholate > menthol > SDS > ethanol > control.
Safety evaluation
From the pictures of duodenal mucosa (), none of the animals of the tested groups showed any signs of inflammation and epithelial damages after intragastrically administrated with enhancers.
Discussion
Cardiovascular disease is the leading cause of death throughout the world. According to data provided by the World Health Organization (WHO) in 2005, an estimated 17.5 million people died from cardiovascular disease in 2005, representing 30% of all global deaths. A tremendous amount of research has been done in the area of prevention and treatment of cardiovascular disease. Fibrinolytic enzymes, isolated from earthworms, have been used as an oral drug for prevention and treatment of thrombosis in East Asia for several thousand years. The enzymes could degrade fibrinogen and fibrin directly and activate plasminogen (PLg). Being a strong protease, EFEs were resistant to degradation by gastrointestinal and cellular enzymes. Thus, EFEs could be absorbed into blood through intestinal epithelium as an intact form and exhibited its biological activity in circulation, remaining approximately 5% of the initial activity (CitationFan et al., 2001). However, the oral delivery of therapeutic proteins has always been a significant challenge for pharmaceutical technologists because of its very poor bioavailability through gastrointestinal tract (CitationMustata & Dinh, 2006). It has been demonstrated that the EFEs’ bioavailability was very low (<0.1%) after oral administration because of its low oil/water partitioning and low membrane permeability (CitationLi et al., 2006).
Caco-2 monolayers, with the advantages of simplicity and reproducibility, have been widely used with in vitro assays to investigate drug permeability. We determined the integrity and tightness of the Caco-2 monolayers by measuring transepithelial passage of paracellular markers: FITC, FD70, and FD2000. As paracellular markers, fluorescein isothiocyanate (FITC, 389.4 Da) and fluorescein- isothiocyanate dextran of molecular masses of 70 kDa and 2000 kDa (FD70 and FD2000), were unable to permeate the cellular membrane at physiologic pH due to its hydrophilicity. These probes had to be transported exclusively via the paracellular route across intestinal epithelia.
In order to determine the suitable concentration and action time of the six enhancers, the acute cytotoxic effects of six enhancers on cell viability were measured through MTT assay. An acceptable balance between absorption enhancing and cytotoxic effects of absorption enhancing principles needed to be considered. After 2 h treatment, the six enhancers (glucose 750 mmol/L, EDTA 2.5 mmol/L, sodium cholate 10 mmol/L, SDS 0.2 mmol/L, ethanol 10%, menthol 1%) induced no significant mitochondrial toxicity towards Caco-2 cells (more than 95% cell viability), which demonstrated that they were safe for the oral delivery of EFEs. In the present study six enhancers have been tested to enhance the oral absorption of EFEs. Under suitable concentrations and action times of various enhancers, the results obtained from in vitro and in vivo experiments showed that the six different enhancers could all improve the intestinal absorption of EFEs without damage to the intestinal tissues.
However, the different enhancers’ mechanisms on opening the paracellular route to increase the EFEs permeability were different. A mechanism for the Na+-co-transported-nutrient effect that caused the expansion of tight junctions has been proposed (CitationMadara & Pappenheimer, 1987; CitationPappenheimer, 1987; CitationPappenheimer & Reiss, 1987). High glucose content in the gut lumen activated the Na+-glucose co-transporter, which was followed by cell swelling and an intercellular phosphorylation cascade. This, in turn, resulted in perijunctional cytoskeleton condensation and a subsequent expansion of tight junctions (CitationKarlsson et al., 1999; CitationTurner, 2000). EDTA could deplete the extracellular Ca2+ and induce tight junction separation by the physical disruption of cell−cell adhesion (CitationWard et al., 2000). EDTA also had been suggested to function by altering protein kinase-C (PKC) signaling which induced the disruption of junctional integrity (CitationTomita et al., 1996). The ability of SDS to increase paracellular permeability might be due to the transient depletion of cellular ATP. After SDS treatment, the cellular morphology was changed, including shortening of microvilli and induction of apical membrane wounds. However, these injuries were reversible (CitationAnderberg & Artursson 1993; CitationCanfield et al., 1991). Ethanol, increasing the paracellular permeability in Caco-2 cells, also produced a disassembly and displacement of perijunctional actin and myosin filaments from the perijunctional areas. After ethanol removal, actin and myosin filaments rapidly reassembled at the cellular borders (CitationMa et al., 1999). Ethanol, at the doses used (<10%), did not cause cytotoxicity to the Caco-2 cells. Bile salts could increase paracellular permeability by acting directly on the junctional components, or indirectly via the cell membrane and cytoskeleton (CitationBleakman & Naftalin, 1990; CitationFreel et al., 1983). Fasano also reported that both chenodeoxycholate (0.5 mM) and ursodeoxycholate (0.5 mM) significantly increased mucosal permeability to lactulose in jejunum and ileum with alterations in tight junctions. The effect of chenodeoxycholate was also shown to be reversible, as it completely disappeared within 40 min after its withdrawal (CitationFasano et al., 1990). Menthol, a white crystalline organic compound, is obtained from peppermint oil or synthesized. It was used individually as mucosa absorption enhancer extensively. The mechanism of menthol in enhancing effect was mainly due to it forming an eutectic with the penetrating compound that was more soluble in the aqueous solution than drug itself (CitationMiguel, 1994; CitationWagner et al., 1994). Furthermore, menthol could enhance the fluidity of the 1oca1 lipid bilayers. A major benefit of using menthol as a permeation enhancer was its safety profile.
The villus in the small intestine contained more than one cell type and the Caco-2 cell line did not produce the mucus as observed in the small intestine. Testing of compound solubility might pose a problem in Caco-2 assays because of the assay conditions. Therefore, we did the transport studies in mice to amend the demerit of Caco-2 monolayers. We found that the absorption enhancement effect obtained from Caco-2 cell monolayers was consistent with the results of mice. Glucose, at concentration of 750 mM, displayed the highest improvement of absorption of EFEs without gross damage to the cells. The order of enhanced efficiencies of various enhancers in vitro and in vivo was as follows: glucose > EDTA > sodium cholate > menthol > SDS > ethanol > control. These results indicated the absorption of EFEs had been enhanced by various enhancers.
Conclusion
Menthol and glucose showed absorption enhancement properties and a relatively low cytotoxicity. It offered an alternative as absorption enhancer as compared to conventional bile acids that might have a potential cocarcinogenic risk. These observations opened new perspectives for using menthol and glucose to improve the transport of other therapeutic molecules by the oral route.
Acknowledgements
We thank Jinghua Zhang and Pengcheng Li for their suggestions in the discussion.
Declaration of interest
This work was supported by the National Science Grant of P.R. China (No. 30871858) and the program of Jiangsu province (BE200830155).
References
- Anderberg EK, Artursson P (1993): Epithelial transport of drugs in cell culture. VIII: Effects of sodium dodecyl sulfate on cell membrane and tight junction permeability in human intestinal epithelial (Caco-2) cells. J Pharm Sci 82: 392–398.
- Anderberg EK, Nystrom C, Artursson P (1992): Epithelial transport of drugs in cell culture. VII: Effects of pharmaceutical surfactant excipients and bile acids on transepithelial permeability in monolayers of human intestinal epithelial (Caco-2) cells. J Pharm Sci 81: 879–887.
- Bleakman D, Naftalin RJ (1990): Hypertonic fluid absorption from rabbit descending colon in vitro. Am J Physiol 258: G377–390.
- Canfield PE, Geerdes AM, Molitoris BA (1991): Effect of reversible ATP depletion on tight-junction integrity in LLC-PK1 cells. Am J Physiol 261: 1038–1045.
- Cano-Cebrian MJ, Zornoza T, Granero L, Polache A (2005): Intestinal absorption enhancement via the paracellular route by fatty acids, chitosans and others: A target for drug delivery. Curr Drug Deliv 2: 9–22.
- Fan Q, Wu C, Li L, Fan R, Wu C, Hou QM, He RQ (2001): Some features of intestinal absorption of intact fibrinolytic enzyme III-1 from Lumbricus rubellus. Biochim Biophys Acta 1526: 286–292.
- Fasano A, Budillon G, Guandalini S, Cuomo R, Parrilli G, Cangiotti AM, Morroni M, Rubino A (1990): Bile acids reversible effects on small intestinal permeability. An in vitro study in the rabbit. Dig Dis Sci 35: 801–808.
- Freel RW, Hatch M, Earnest DL, Goldner AM (1983): Role of tight-junctional pathways in bile salt-induced increases in colonic permeability. Am J Physiol 245: G816–823.
- Fricker G, Fahr A, Beglinger C, Kissel T, Reiter G, Drewe J (1996): Permeation enhancement of octreotide by specific bile salts in rats and human subjects: In vitro, in vivo correlations. Br J Pharmacol 117: 217–223.
- Karlsson J, Ungell A, Grasjo J, Artursson P (1999): Paracellular drug transport across intestinal epithelia: Influence of charge and induced water flux. Eur J Pharm Sci 9: 47–56.
- Li YH, Zhang M, Wang JC, Zhang S, Liu JR, Zhang Q (2006): Effects of absorption enhancers on intestinal absorption of lumbrokinase. Yao Hsueh Hsueh Pao 41: 939–944.
- Liu DZ, LeCluyse EL, Thakker DR (1999): Dodecylphosphocholine-mediated enhancement of paracellular permeability and cytotoxicity in Caco-2 cell monolayers. J Pharm Sci 88: 1161–1168.
- Ma TY, Nguyen D, Bui V, Nguyen H, Hoa N (1999): Ethanol modulation of intestinal epithelial tight junction barrier. Am J Physiol 276: 965–974.
- Madara JL, Pappenheimer JR (1987): Structural basis for physiological regulation of paracellular pathways in intestinal epithelia. J Membr Biol 100: 149–164.
- Miguel R (1994): Eutectic mixture of local anesthetics: Not just for kids. Reg Anesth 19: 75–76.
- Mosmann T (1983): Rapid colorimetric assay for cellular growth and survival: Application to proliferation and cytotoxicity assays. J Immunol Methods 65: 55–63.
- Mustata G, Dinh SM (2006): Approaches to oral drug delivery for challenging molecules. Crit Rev Ther Drug Carrier Syst 23: 111–135.
- Pappenheimer JR (1987): Physiological regulation of transepithelial impedance in the intestinal mucosa of rats and hamsters. J Membr Biol 100: 137–148.
- Pappenheimer JR, Reiss KZ (1987): Contribution of solvent drag through intercellular junctions to absorption of nutrients by the small intestine of the rat. J Membr Biol 100: 123–136.
- Qiao F, Cen W, Li L, Rong F, Cheng W, Quanmin H, Rongqiao H (2001): Some features of intestinal absorption of intact fibrinolytic enzyme III-1 from Lumbricus rubellus. Biochim Biophys Acta 1526: 286–292.
- Ross BP, Toth I (2005): Gastrointestinal absorption of heparin by lipidization or coadministration with penetration enhancers. Curr Drug Deliv 2: 277–287.
- Tomita M, Hayashi M, Awazu S (1996): Absorption-enhancing mechanism of EDTA, caprate, and decanoylcarnitine in Caco-2 cells. J Pharm Sci 85: 608–611.
- Turner JR (2000): Show me the pathway: Regulation of paracellular permeability by Na+-glucose cotransport. Adv Drug Deliv Rev 41: 265–281.
- Wagner RF, Jr-Flores CA, Argo LF (1994): A double-blind placebo controlled study of a 5% lidocaine/prilocaine cream (EMLA) for topical anesthesia during thermolysis. J Dermatol Surg Oncol 20: 148–150.
- Ward PD, Tippin TK, Thakker DR (2000): Enhancing paracellular permeability by modulating epithelial tight junctions. Pharm Sci Tech Today 3: 346–358.
- Zhang X, Zhang X, Yang Q (2007): Effect of compound mucosal immune adjuvant on mucosal and systemic immune responses in chicken orally vaccinated with attenuated Newcastle-disease vaccine. Vaccine 25: 3254–3262.