Abstract
Context: Although clinical data for beneficial effects of Betaferon, human recombinant-interferon (r-IFN) β-1b, are accumulating, what is less evident is how and why it works.
Objective: The present study was carried out to examine whether Betaferon suppresses progression of experimental autoimmune encephalomyelitis (EAE).
Materials and methods: The EAE model was employed in guinea pigs in vivo, and mononuclear cell proliferation and 2′,5′-oligoadenylate synthetase activity were assessed in vitro.
Results: Betaferon was more reactive in two assays of guinea pigs, mitogen-induced proliferation of peripheral blood mononuclear cells and 2′,5′-oligoadenylate synthetase activity of blood, than in rats and rabbits. Guinea pigs were immunized actively by antigen, porcine myelin basic protein. The neurological deficits were assessed by clinical signs scored daily. Guinea pig Betaferon, replaced with guinea pig albumin (GPA), at 1.2 and 12.0 MIU/kg/day or vehicle was administered subcutaneously daily for 20 days in the immunized guinea pigs. GPA-Betaferon suppressed the manifestation of ataxia or more progression of chronic neurological deficits significantly at 1.2 MIU/kg (p <0.05). Two out of 10 animals manifested no clinical signs in the GPA-Betaferon-treated group with the higher dose, while all animals were worsened with typical clinical signs of EAE in the vehicle group where mononuclear cell infiltrates around blood vessels were seen in the spinal cord of vehicle-treated animals.
Discussion and conclusion: Human r-IFN β-1b attenuates progression of neurological deficits in the EAE model of guinea pigs with evidence for higher susceptibility of animal cells/tissues to the human cytokine, in contrast with rodents and rabbits.
Introduction
Multiple sclerosis (MS) is induced by destruction of the protective coating on nerve cells, the myelin sheath for promoting the transmission of nerve impulses in the CNS. As the myelin sheath becomes impaired by inflammation for demyelination, the impulse progress down the bare axon is slowed, eliciting nervous system dysfunction, e.g. tingling, numbness or weakness of a leg, and loss of balance (CitationArnason et al., 1996; CitationYong et al., 1998). It was demonstrated in the pivotal clinical trial that Betaferon, a recombinant and modified form of human IFN β-1b, reduces the rate of exacerbation of relapsing-remitting MS (CitationIFN β Multiple Sclerosis Study Group, 1993; CitationPaty et al., 1993), curtailing accumulating disease burden drastically as assessed by magnetic resonance imaging (MRI) scans over at least 5 years (CitationLublin et al., 1996; CitationIFN β Multiple Sclerosis Study Group, 1995).
Although clinical data for beneficial effects of Betaferon are accumulating, what is less evident is how and why it works (CitationYong et al., 1998). In correspondence with the human results, “murine IFN β”, which is not the same as the cytokine drug employed in humans, can alleviate experimental autoimmune encephalomyelitis (EAE) (CitationYu et al., 1996), an inflammatory condition in animals that bears similarities to MS. Human IFN beta, to the contrary, had no effect in the rat EAE model, while “rat IFN β” was active in reducing the severity of clinical symptoms of paralysis (CitationHertz & Deghenghi, 1985). Thus, the animal studies in vivo would make the interpretation more difficult, inasmuch as human-derived cytokines are used in conditions without evidence for corresponding sensitivity of animal cells/tissues to them.
The present study was carried out first to determine if animal-derived 2′,5′-oligoadenylate synthetase (2-5 AS), directly involved in the impairment of viral replication (CitationMannering & Deloria, 1986), and mononuclear cells, are sensitive or reactive to human r-IFN β-1b, comparing the sensitivity in three animal species: guinea-pigs, rabbits and rats. Secondly, IFN β-1b material was formulated with guinea-pig albumin instead of human albumin, i.e., GPA-Betaferon, in order to avoid the heterogeneous protein-related shock by repeated administration. It was followed by a study of guinea pig EAE model in vivo with GPA-Betaferon. In other words, these two in vitro studies are performed to ensure the guinea-pig models are closer to human data in comparison with other animal species in a sense of response direction and sensitivity. An understanding in guinea-pig models in vivo and in vitro, in which IFN β-1b is active, could pave the way for more relevant mechanism for Betaferon in multiple sclerosis.
Methods
Animals
Male Hartley guinea pigs, 5 weeks of age (Japan SLC, Shizuoka, Japan), male Lewis rats, 5 weeks of age (Seac Yoshitomi, Fukuoka, Japan) and male Japanese White rabbits, 2.5–3 kg (Shimizu Laboratory Supplies, Kyoto, Japan) were used for studies on proliferation of peripheral blood mononuclear cells (PBMCs) and enzyme activity of 2′,5′-oligoadenylate synthetase (2-5 AS). Thirty male guinea pigs of Hartley strain at age of 3 weeks (Japan SLC) were used for EAE study. Animals were housed to a cage, and maintained on standard laboratory chow and tap water ad libitum at a room temperature of 19–25°C and humidity of 30–70% with the light/dark cycle. The study was carried out in accordance with the Guide for the Care and Use of Laboratory Animals endorsed by The Japan Pharmacological Society.
Human recombinant interferon β-1b (human r-IFN β-1b)
Human r-IFN β-1b (Betaferon®, Chiron, Emmeryville, CA) was used in PBMC proliferation and 2-5 AS-induction studies. Betaferon contains human serum albumin and dextrose as a stabilizer, so that anaphylactic shock may be induced following repeated administration to animals with human albumin for EAE testing. IFN β-1b for guinea pigs EAE was thus prepared from an intermediate without human albumin and dextrose during formulation processes of Betaferon production. It was dissolved in sodium dodecyl sulfate (SDS), supplied by Chiron. Human r-IFN β-1b, without human albumin, in SDS was desalted and eluted through a G-25 cartridge column (Pharmacia Biotech, USA), and then stabilized with guinea-pig albumin (Fraction V; Sigma, St. Louis, MO) and dextrose (Baxter, Deerfield, IL). Hereafter, this compound used for EAE study is referred to as GPA-Betaferon.
Peripheral blood mononuclear cell proliferation assays (PBMC assay)
PBMCs were prepared from heparinized peripheral blood of animals, guinea pigs, rats, and rabbits. The mononuclear cells were purified by density gradient centrifugation on Ficoll-Paque (Pharmacia, Piscataway, NJ) according to the method reported elsewhere (CitationWakabayashi et al., 1994; CitationLowry et al., 1993). Briefly, whole blood cells were placed in an equivalent volume of RPMI 1640 medium (Gibco, Grand Island, NY) and underlayered with the Ficoll-Paque. After centrifugation (400 g, 30 min), the buffy coat of PBMC layer was harvested and a small amount of red blood cells lysed by treatment with 0.83% NH4Cl solution. Thereafter, PBMCs were washed twice with phosphate buffered saline (PBS), and resuspended at 5 × 105 cells/mL in RPMI 1640 medium, including 10% heat-inactivated fetal bovine serum (Intergen, New York), 100 μg/mL streptomycin (Sanko Junyaku, Tokyo), and 100 U/mL penicillin (Sanko Junyaku). PBMCs isolated from each animal were dispensed into 96-well round-bottomed culture plates (Corning, MA) at 4 × 104 cells/well. Betaferon (1.92 × 106 IU) was first dissolved in 0.53% saline and then diluted in the culture medium, and added to the cell suspension at final concentrations of 102, 103 and 104 IU/mL; they were determined by antiviral activity in a separate experiment according to the methods reported elsewhere (CitationRubinstein et al., 1981). PBMC suspensions were treated with Betaferon or vehicle at 37°C in a humidified atmosphere with 5% CO2 for 1 h. After the pretreatment, guinea-pig-PBMC and rabbit-PBMC in culture were stimulated with 10 μg/mL of phytohemagglutinin (PHA, Vector Laboratories, Burlingame, CA), while rat-PBMC in culture were stimulated with 3 μg/mL of concanavalin A (Con A, Seikagaku, Tokyo). The cells in culture were pulsed with 1 μCi [3H]thymidine (5.0 Ci/mM, Amersham, Buckinghamshire, UK) in 48 h treatment with PHA or Con A, and were harvested 24 h later. The incorporated amount of radioactivity was determined in a scintillation counter. Results were expressed as mean counts per minute (cpm).
2′,5′-Oligoadenylate synthetase (2-5 AS) induction
It was determined whether there was interferon-specific gene modulation in different species of animals, assaying 2-5 AS activity in peripheral blood in response to Betaferon ex vivo. Betaferon at doses of 0.53 MIU/kg to 26.67 MIU/kg IFN-β1b was given subcutaneously into the abdomen of the animals. At 0, 24, 48 and 96 h after the Betaferon injection, the peripheral blood samples (100–200 μL/time) were taken out through a vein (paw vein: guinea pigs, tail vein: rats, auricular vein: rabbits). Blood samples were diluted one-tenth with physiological saline and stored at −80°C until assayed. The 2-5 AS activity was determined by using radioimmunoassay kits (2-5A kit, Eiken, Tokyo) according to the instruction manual. First, diluted blood samples were frozen and thawed twice. Lysate at a volume of 50 μL was incubated with poly(I) poly(C) agarose beads for 10 min at a room temperature, and centrifuged (800 g, 5 min). Pellets for 2-5 AS-conjugated beads were incubated in 100 μL of the buffer, containing ATP at concentration of 200 μM, for 30 min at 37°C. 125I-labelled 2′,5′-Oligoadenylate (2-5A) solution (100 μL) and antiserum against 2-5A (100 μL) were added to the buffered pellets, and incubated for an hour at 37°C. Thereafter, samples were centrifuged (4°C, 2000 g, 30 min), and the radioactivity of the precipitate was determined in a gamma counter. The 2-5 AS activity was expressed in pmol/dL, generating, during the 30 min incubation with ATP; calculated based on the standard curve.
Acute experimental autoimmune encephalomyelitis
Porcine myelin basic protein (P-MBP) which was isolated from porcine spinal cords by the method of CitationSwanborg et al. (1974) was used as an antigen in guinea pigs. P-MBP in PBS was emulsified with an equal volume of Freund’s complete adjuvant (FCA) (Difco Laboratories, Burlingame, CA) containing 100 μg of heat-killed Mycobacterium tuberculosis (Difco). The antigen emulsion containing 7.5 μg of P-MBP at a total volume of 0.2 mL was injected into the hind footpads on day 0. Guinea pigs were divided into three groups as follows:
Group 1: Control, vehicle treated with no GPA-Betaferon treatment (n = 10)
Group 2: Treated with GPA-Betaferon at 1.2 MIU/kg/day (n = 10)
Group 3: Treated with GPA-Betaferon at 12 MIU/kg/day (n = 10)
The present study on IFN β was focused on whether or not there are marked changes in effects of very high versus relatively low doses, ten times difference.
Neurological signs for EAE
GPA-Betaferon (1.2 MIU/kg/day, 12 MIU/kg/day) or vehicle (albumin of guinea pigs and dextrose) were administered subcutaneously into abdomen daily for 20 days, starting from the day of active sensitization (day 0). Approved human therapeutic dose is 8 MIU, suggesting 0.1–0.13 MIU/kg sc (60–80 kg of body weight). Preliminary study and published studies elsewhere in animals showed some effect at doses much more than the human dose: 10 times or more. Thus, 1.2 MIU and the very high dose of 12 MIU/kg was chosen. Guinea pigs were weighed and their neurological signs of EAE were assessed for that period of time every day. The behavioral changes for neurological deficits were graded using three clinical scores as follows: 0, normal; 1, ataxia development or loss of body weight; 2, paralysis of the hind legs; 3, complete/severe loss of motor activity or death. All experiments were performed in a blinded manner; scored by a person who had no prior knowledge of drug treatment for each animal. Average daily clinical score was calculated for each group each day. The clinical score for each guinea pig was accumulated for 20 days. The mean cumulative clinical score for each group was calculated by dividing the sum of all the accumulated clinical scores for guinea pigs in a given group by the number of guinea pigs in that group.
Histology
Guinea-pigs under anesthesia were perfused intravenously with 4% paraformaldehyde (PFA) in phosphate buffer (PB) at pH of 7.3. Cerebrums and spinal cords were isolated, fixed in 4% PFA in PB, and embedded in paraffin or epoxy resin. Paraffin sections were stained with hematoxylin and eosin (HE). Sections were examined under light microscopy.
Statistical analysis
The results were expressed as the mean ± SD. The data of 2-5 AS study was analyzed using paired t-test. The other results were analyzed by one-way analysis of variance (ANOVA) or Kruskal-Wallis test, followed by multiple analysis with Dunnett’s multiple comparison test among groups. Statistically significant difference was noted at p <0.05.
Results
Effects of Betaferon on mitogen-induced proliferation of PBMC
Effects of Betaferon on mitogen-induced proliferation of PBMC and on 2-5 AS induction after treatment with Betaferon were examined in three species of animals: guinea pigs, rabbits and rats. As shown in , Betaferon inhibited PHA-induced proliferation of PBMC isolated from guinea pigs in a concentration-dependent manner. Significant inhibition by Betaferon was observed at the concentrations of 1,000 and 10,000 IU/mL. The inhibitory effect of Betaferon on proliferation of PBMC isolated from guinea pigs was similar to those on human PBMC (CitationRudick et al., 1993). By contrast, Betaferon at 100 and 1,000 IU/mL did not inhibit in rabbits and rats, and at 10,000 IU/mL rather enhanced the PHA-induced proliferation of PBMC isolated from rabbits ( and ). In addition, Betaferon enhanced the Con A-induced proliferation of PBMC isolated from rats in a concentration-dependent manner at 100 to 10,000 IU/mL (, and ).
Figure 1. Effect of IFN beta-1b on mitogen-induced proliferation of peripheral blood mononuclear cells of guinea pigs (A), rabbits (B) and rats (C). The mononuclear cells were treated with 10 μg/ml PHA (Figures A and B) or 3 μg/ml Con A (Figure B) for 72 h. The cell proliferation was assessed by [3H]-TdR uptake. Cells were pulsed with [3H]-TdR 24 h before harvesting. Results represent means ± SD (n = 5). Statistical analysis was made according to Dunnett’s test; significant difference was noted in the figures at p <0.05.
![Figure 1. Effect of IFN beta-1b on mitogen-induced proliferation of peripheral blood mononuclear cells of guinea pigs (A), rabbits (B) and rats (C). The mononuclear cells were treated with 10 μg/ml PHA (Figures A and B) or 3 μg/ml Con A (Figure B) for 72 h. The cell proliferation was assessed by [3H]-TdR uptake. Cells were pulsed with [3H]-TdR 24 h before harvesting. Results represent means ± SD (n = 5). Statistical analysis was made according to Dunnett’s test; significant difference was noted in the figures at p <0.05.](/cms/asset/b632e790-4cff-427e-911c-262455cd2e8f/iphb_a_477535_f0001_b.gif)
Figure 2. Changes in 2′,5′-oligoadenylate synthetase (2-5 AS) activity of peripheral blood after treatment of animals with Betaferon. Betaferon or IFN-β1b was given subcutaneously into the abdomen of the animals. The 2-5AS activity of peripheral blood samples was determined at 0, 24, 48 and 96 h after Betaferon injection. Each point represents the mean ± SD of four animals. *Significantly different from pre-values at the time 0, p <0.05; **p <0.01; ***p <0.001 (paired t-test).
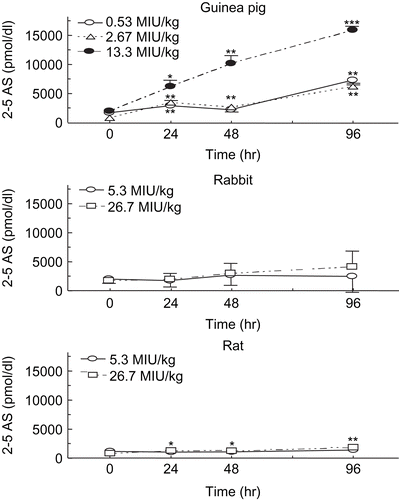
Figure 3. Inhibitory effects of Betaferon on neurological deficits of guinea pigs that were immunized with porcine myelin basic protein (MBP). Vehicle (——) or GPA-Betaferon at 1.2 MIU/kg (- - -) or 12 MIU/kg (- — -) was administered subcutaneously daily for 20 days. The mean of the daily clinical scores for each group of 10 animals is shown. See for the statistical analysis.
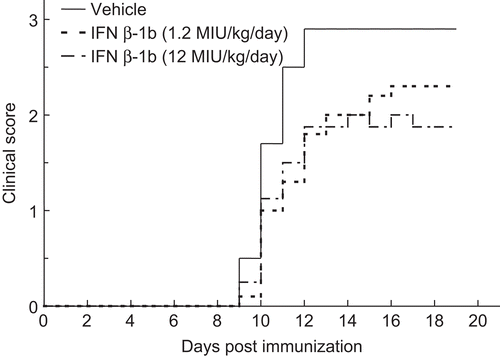
Table 1. Cumulative clinical scores of EAE guinea pigs after repeated subcutaneous administration of GPA-Betaferon.
Effects of Betaferon on 2-5AS induction
At a dose of 13.3 MIU/kg, 2-5AS activity in peripheral blood of guinea pigs was significantly induced 24 h after the treatment with Betaferon, compared to 0 h (). 2-5AS induction was furthermore augmented in a time-dependent manner in a period of 48 to 96 h after the injection of Betaferon at doses of 0.53 MIU/kg or more. On the other hand, Betaferon failed to enhance 2-5AS induction in rats and rabbits, or enhanced to a markedly lesser extent.
Effect of GPA Betaferon in guinea pig EAE model
Guinea pigs were immunized with porcine MBP. As shown in , all guinea pigs treated with vehicle developed neurological deficits for EAE manifestation that began on day 10–12 post immunization and the deficits remained or were exacerbated until day 20. When guinea pigs were treated with GPA-Betaferon at a dose of 1.2 MIU/kg/day, all of them also showed neurological signs of EAE, but the peak response for the neurological deficits was less severe than in the vehicle-treated group. There was significant difference (p <0.05) of cumulated clinical scores seen between 1.2 MIU/kg/day GPA-Betaferon- and vehicle-treated groups (). Guinea pigs treated with GPA-Betaferon at a higher dose (12 MIU/kg/day) also showed fewer peak responses for the neurological deficits. No significant difference was observed statistically between the group of GPA-Betaferon at high dose and the vehicle-treated group, but it is noted that for the former guinea pigs, two out of ten animals used did not manifest any clinical signs for neurological deficits, compared to manifestation in all the animals used in the latter group. Progression of neurological deficits and their inhibition was linked to changes in body weight of guinea pigs as shown in , in relation to days, in particular 10 days or later after their immunization with P-MBP.
Figure 4. Effects of GPA-Betaferon on body weight in guinea pigs sensitized with porcine myelin basic protein (P-MBP). Vehicle (•) or GPA-Betaferon at 1.2 MIU/kg (○) or 12 MIU/kg (□) was administered subcutaneously daily for 20 days. Each value represents the mean ± SD, and figures in parentheses indicate the number of surviving animals.
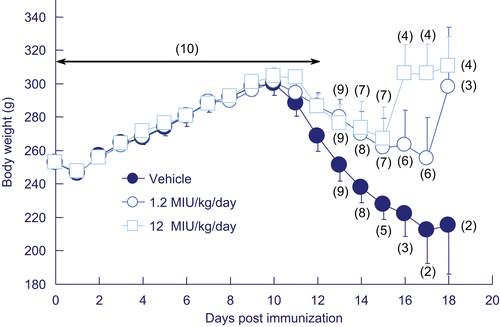
Discussion
Active immunization of guinea pigs with porcine MBP elicited neurological deficits as a model of EAE, associated with loss of body weight. Behavioral changes began to take place 10 to 12 days after the immunization, thereafter clinical signs of disease were made worse up to death; as many as 8 out of 10 animals used in control. Accumulation of mononuclear cells around blood vessels in the spinal cord in histological examination suggests that inflammatory processes went on toward exacerbation of disease, presumably accompanying increased permeability of the blood–brain barrier (BBB). An immune-mediated attack on the oligodendrocytes that synthesize MBP is made by the Th 1 cells and the tissue macrophages/microglias that become activated through Th 1 cell cytokine action, mediating demyelination (CitationCantorna et al., 1996). The onset of clinical signs in the guinea pig model appears in a similar period of time for rats and mice; 6-12 days, [6-7 days reported by CitationHertz and Deghenghi (1985), 9-12 days by Citationvan der Meide et al. (1998) and 10 days by CitationCantorna et al. (1996)] after immunization. Hind limbs paralysis was also defined as a major sign in the rodent’s model as well. Thus, the present model does not seem to differ among animal models in terms of gross behavioral changes.
Human r-IFN β-1b in a form of GPA-Betaferon suppressed significantly the progression of neurological deficits in a course of EAE development at the dose of 1.2 MIU/kg/day. Although there was no significant difference in suppressing at a higher dose of GPA-Betaferon, two cases with no exacerbation seen were in contrast to all cases with the most severe degree of impairment in the vehicle-treated group, and this would be contributing to the increased variance. As far as we know, our findings are the first demonstration that human r-IFN β-1b is efficacious in an animal EAE model, while it failed to suppress in the rat EAE model in contrast to rat IFN β effective (CitationHertz & Deghenghi, 1985). Since mononuclear cells were markedly less infiltrated into the brain parenchyma by chronic treatment with GPA-Betaferon (preliminary data), it is conceivable that the antigen specific T-cells and activated macrophages are suppressed for their migration into the brain in association with BBB damage (CitationSwanborg, 1995). IFN beta is recognized to inhibit the proliferation of T lymphocytes (CitationYong et al., 1998; CitationNoronha et al, 1993). Susceptibility to EAE correlates with a predominant Th1 responses to myelin antigens, and resistance to disease induction correlates with a predominant Th2 immune response in mice and Lewis rats (CitationYong et al., 1998; CitationSmeltz & Swanborg, 1998), suggesting that Th1 cytokines may propagate inflammation in MS. In the present study, PHA-induced proliferation of peripheral blood mononuclear cells in guinea pigs in vitro was attenuated concentration-dependently in a similar manner to that of humans, suggesting that Betaferon may reduce proliferation and activation of inflammatory monocytes, presumably in response to T cell activation. T lymphocytes are known to produce matrix metalloproteinases by which the basement membrane is degraded for the inflammatory cells to migrate into the CNS parenchyma.
Mononuclear cells and blood derived from guinea pigs were markedly more sensitive or reactive to human r-IFN β-1b, i.e. Betaferon, in terms of the cellular proliferation and 2-5AS activity against viral replication than those from any other animal species. The inhibitory effect of Betaferon on proliferation of the peripheral blood monocytes may be linked with differentiation-dependent regulation of class II MHC antigen expression observed in astrocytes/microglias (CitationFertsch et al., 1987; CitationTraugott et al., 1985), whether it exerts directly or indirectly. In active demyelinating lesions of MS, both class II MHC antigen and ICAM-1 are expressed prominently in astrocytes, along with IFN-r, a potent inducer of MHC antigens and ICAM-1 (CitationTraugott & Lebon, 1988; CitationSobel et al., 1990). These would result in activation of the immune system, which may lead to exacerbation of autoimmune processes (CitationNepom & Erlich, 1991). Modulation of IFNγ-MHC antigen-axis (CitationLing et al., 1985) may be a key for the inhibitory effects of Betaferon in the autoimmune encephalomyelitis model. Thus, further investigation of guinea pig models in vivo and in vitro together would generate useful information for inflammatory processes at three different points of stages, before, on-going, and after manifestation.
Conclusion
Human r-IFN β-1b attenuates progression of neurological deficits in the EAE guinea pig model with evidence for higher susceptibility of animal cells/tissues to the human cytokine, in contrast with rodents and rabbits.
Declaration of interest
The authors declare no conflict of interest.
References
- Arnason BG, Dayal A, Qu ZX, Jensen MA, Genc K, Reder AT (1996): Mechanisms of action of interferon-beta in multiple sclerosis. Springer Semin Immunopathol 18: 125–148.
- Cantorna MT, Hayes CE, DeLuca HF (1996): 1,25-Dihydroxyvitamin D3 reversibly blocks the progression of relapsing encephalomyelitis, a model of multiple sclerosis. Proc Natl Acad Sci USA 93: 7861–7864.
- Fertsch D, Schoenberg DR, Germain RN, Tou JY, Vogel SN (1987): Induction of macrophage Ia antigen expression by rIFN-gamma and down-regulation by IFN-alpha/beta and dexamethasone are mediated by changes in steady-state levels of Ia mRNA. J Immunol 139: 244–249.
- Hertz F, Deghenghi R (1985): Effect of rat and beta-human interferons on hyperacute experimental allergic encephalomyelitis in rats. Agents Actions 16: 397–403.
- IFNβ Multiple Sclerosis Study Group, University of British Columbia MS/MRI Analysis Group (1995): Interferon beta-1b in the treatment of multiple sclerosis, final outcome of the randomized controlled trial. Neurology 45: 177–185.
- IFNβ Multiple Sclerosis Study Group (1993): Interferon beta-1b is effective in relapsing-remitting multiple sclerosis. I. Clinical results of multicenter, randomized, double-blind, placebo-controlled trial. Neurology 43: 655–661.
- Ling PD, Warren MK, Vogel SN (1985): Antagonistic effect of interferon-beta on the interferon-gamma-induced expression of Ia antigen in murine macrophages. J Immunol 135: 1857–1863.
- Lowry PW, Sabella C, Koropchak CM, Watson BN, Thackray HM, Abbruzzi GM, Arvin AM (1993): Investigation of the pathogenesis of varicella-zoster virus infection in guinea pigs by using polymerase chain reaction. J Infect Dis 167: 78–83.
- Lublin FD, Whitaker JN, Eidelman BH, Miller AE, Arnason BG, Burks JS (1996): Management of patients receiving interferon beta-1b for multiple sclerosis: Report of a consensus conference. Neurology 46: 12–18.
- Mannering GJ, Deloria LB (1986): The pharmacology and toxicology of the interferons: An overview. Annu Rev Pharmacol Toxicol 26: 455–515.
- Nepom GT, Erlich H (1991): MHC class-II molecules and autoimmunity. Annu Rev Immunol 9: 493–525.
- Noronha A, Toscas A, Jensen MA (1993): Interferon beta decreases T cell activation and interferon gamma production in multiple sclerosis. J Neuroimmunol 46: 145–153.
- Paty DW, Li DK, UBC MS/MRI Study Group (1993): IFNβ Multiple Sclerosis Study Group. Interferon beta-1b is effective in relapsing-remitting multiple sclerosis. II. MRI analysis results of a multicenter, randomized, double-blind, placebo-controlled trial. Neurology 43: 662–667.
- Rubinstein S, Familletti PC, Pestka S (1981): Convenient assay for interferons. J Virol 37: 755–758.
- Rudick RA, Carpenter CS, Cookfair DL, Tuohy VK, Ransohoff RM (1993): In vitro and in vivo inhibition of mitogen-driven T-cell activation by recombinant interferon beta. Neurology 43: 2080–2087.
- Smeltz RB, Swanborg RH (1998): Concordance and contradiction concerning cytokines and chemokines in experimental demyelinating disease. J Neurosci Res 51: 147–153.
- Sobel RA, Mitchell ME, Fondren G (1990): Intercellular adhesion molecule-1 (ICAM-1) in cellular immune reactions in the human central nervous system. Am J Pathol 136: 1309–1316.
- Swanborg RH, Swierkosz JE, Saieg RG (1974): Studies on the species-variability of experimental allergic encephalomyelitis in guinea pigs and rats. J Immunol 112: 594–600.
- Swanborg RH (1995): Experimental autoimmune encephalomyelitis in rodents as a model for human demyelinating disease. Clin Immunol Immunopathol 77: 4–13.
- Traugott U, Lebon P (1988): Multiple sclerosis: Involvement of interferons in lesion pathogenesis. Ann Neurol 24: 243–251.
- Traugott U, Scheinberg LC, Raine CS (1985): On the presence of Ia-positive endothelial cells and astrocytes in multiple sclerosis lesions and its relevance to antigen presentation. J Neuroimmunol 8: 1–14.
- Wakabayashi G, Cannon JG, Gelfand JA, Clark BD, Aiura K, Burke JF, Wolff SM, Dinarello CA (1994): Altered interleukin-1 and tumor necrosis factor production and secretion during pyrogenic tolerance to LPS in rabbits. Am J Physiol 267: R329–336.
- Yong VW, Chabot S, Stuve O, Williams G (1998): Interferon beta in the treatment of multiple sclerosis: Mechanisms of action. Neurology 51: 682–689.
- Yu M, Nishiyama A, Trapp BD, Tuohy VK (1996): Interferon-beta inhibits progression of relapsing-remitting experimental autoimmune encephalomyelitis. J Neuroimmunol 64: 91–100.
- van der Meide PH, de Labie MC, Ruuls SR, Groenestein RJ, Botman CA, Olsson T, Dijkstra CD (1998): Discontinuation of treatment with IFN-beta leads to exacerbation of experimental autoimmune encephalomyelitis in Lewis rats. Rapid reversal of the antiproliferative activity of IFN-beta and excessive expansion of autoreactive T cells as disease promoting mechanisms. J Neuroimmunol 84: 14–23.