Abstract
Context: Tianhua (TH-R) is extracted from Trichosanthes kirilowii Maxim (Cucurbitaceae) containing trichosanthin, a traditional Chinese medicine, which has been locally reported to have good anticancer effects in vivo in both animal and human models. However, there have been several reports that trichosanthin has an anticancer effect involving apoptosis.
Objective: To investigate other anticancer effects of TH-R, various tumorigenesis parameters were verified.
Materials and methods: Telomerase activity, anti-apoptosis, anti-migration and immunomodulatory activity were estimated by telomeric repeat amplification protocol assay (TRAP), flow cytometry, Boyden chamber assay and ELISA assay, respectively.
Results: In our studies, we are the first to find that TH-R had a cytotoxic effect on lung cancer cells in MTS assays; it could change the cell cycle distribution of human lung cancer cells (A549 cell line) and induce apoptosis. Further anti-telomerase effects in human lung adenocarcinoma A549 cells using the TRAP assay were noted. TH-R also had an aggregation effect on peripheral blood lymphocytes, but no effect on stimulating peripheral lymphocytes to produce human interferon-γ(IFN-γ). TH-R could inhibit the migration, or metastatic ability, of A549 cells by Boyden chamber assay. In the oral feeding therapy of an in vivo mouse model, there was an initial inhibition of A549 cancer cell growth, but no statistical difference after one month of therapy.
Discussion and conclusion: It has been proven that medicinal herbs such as Tianhua have positive effects against cancer through preventing or inhibiting the process of lung tumorigenesis.
Introduction
Trichosanthes kirilowii Maxim is a type of liana of the Cucurbitaceae family whose root tuber has been used in China to reset menstruation and expel retained placenta. Trichosanthes kirilowii containing trichosanthin has been used for centuries in China for abortion and for the cure and treatment of ectopic pregnancy and choriocarcinoma (CitationZhang et al., 2001). Hepatoprotective activity of Trichosanthes cucumerina var. cucumerina L. is against carbon tetrachloride-induced liver injury has been reported (CitationSathesh Kumar et al., 2009). Trichosanthin (TCS) is a type I ribosome-inactivating protein that inactivates the ribosome by enzymatically depurinating the A(4324) at the α-sarcin/ricin loop of 28S rRNA (CitationChan et al., 2007; CitationMaraganore et al., 1987). A previous study demonstrated that the ethyl acetate extract of tian hua fen had a significant protective effect on HeLa cells infected with coxsackie virus B3 (CitationLi et al., 2004). Cucurbitacin D, isolated from Trichosanthes kirilowii, could be a valuable candidate as an antitumor drug (CitationTakahashi et al., 2009). Trichosanthin (TCS) is an active component extracted from the root tubers of traditional Chinese medical herb tian hua fen of the Cucurbitaceae family (CitationZhang et al., 2007). TCS could inhibit the proliferation of various leukemia/lymphoma cell lines, but the effect of TCS on T-lymphocyte and macrophage cell lines showed induction of cell apoptosis, and the effect of TCS on B lymphocyte cell line showed inhibition of cell growth (CitationWang et al., 2007). The purified trichosanthin component can markedly inhibit melanoma cells by the suppression of DNA synthesis in S phase and cell mitosis, as well as induction of apoptosis (CitationBi et al., 1998).
There are at least six hallmarks of cancer development, including evading apoptosis, limitless replication, tissue invasion, and metastasis. Most, if not all, cancers acquire the same set of functional capabilities during their development, albeit through various mechanistic strategies (CitationHanahan & Weinberg, 2000). Turning on telomerase will promote the limitless replicative potential of cancer cells. High telomerase activity correlates with the degree of malignancy and the likelihood of tumor progression. Therefore, inhibiting the activity of telomerase will induce the loss of telomeres from the DNA fragments in cell division and delay tumor growth, and even result in cell death before DNA repair and antiapoptosis. In our previous study, we found that reactivated telomerase activity may be a poor prognostic factor in non-small cell lung cancer (CitationWu et al., 2003). Lung cancer patients without telomerase activity survived for a significantly longer period than those with telomerase activity. There is now accumulating evidence of the anti-cancer effects of natural medicines. Here we document a compound that potentially possesses anti-tumor effects.
Tianhua™ (TH-R) is modified from tian hua fen. Strict and systematic pre-clinical studies are necessary and essential to establish the efficacy and safety of Oriental herbs and formulas in order to transform traditional herbal practices into evidence-based medicine. The aim of this study is to demonstrate the mechanism and immunomodulation of TH-R in inhibiting the growth of human lung cancer cells using a cell viability assay for the inhibition of cell growth, a flow cytometry for cell cycle distribution change and apoptosis, an ELISA test for the analysis of cytokines such as human IFN-γ stimulated by TH-R, a TRAP assay to evaluate the effect of TH-R on telomerase activity, Boyden chamber assay to evaluate the effect of TH-R on the migration of lung cancer cells, and an in vivo mouse model to evaluate the effects of orally fed TH-R.
Material and methods
Cell lines and chemicals
We obtained A549 human lung adenocarcinoma and WI-38 human lung fibroblast cell lines from the American Type Culture Collection (Rockville, MD). The cell lines were maintained at 37°C in a humidified atmosphere of 5% CO2 in Dulbecco’s modified Eagle’s medium (DMEM) (GIBCO, Rockville, MD) containing 10% fetal bovine serum (FBS) (Life Technologies, Rockville, MD) and 100 ng/mL each of penicillin and streptomycin (Life Technologies). Trichosanthes kirilowii was obtained from a plantation of Richmond Farm (Richmond, B.C. Canada). Their authenticity was confirmed by our colleague S.-P. Huang. The root tubers of these plants were harvested and mixed in a fixed ratio and preserved at room temperature to produce TH-R. TH-R is manufactured by the Canadian Phytopharmaceuticals Corporation authorized by Bin Shing Pharmaceutical Co., Ltd (Taipei, Taiwan). It is generated and ameliorated from Tianhua™ (international patent number CN1051861). In brief, the dried plant materials (20 g) were ground using an electric grinder. The powder was extracted with 250 mL cooled distilled water under reflux for 2 h. The insoluble debris was removed after centrifugation (6000 rpm, 10 min). The extract was concentrated into 5 mL using a concentrator. The concentrated extract was applied on a Sephadex G-25 (2 × 50 cm Amersham Bioscience), equilibrated, were eluted (20 mL/h) with distilled water, and 3 mL fractions were aliquoted. The active fraction was determined by MTS assay for cytotoxicity of lung cancer cells. The active fractions were dried using a freeze drier and stored at −20°C until assay.
The TH-R solution was prepared by adding 100 mg of TH-R powder dissolved in 1 mL of distilled water (100 mg/mL), then shaken (Vortex-Genie 2) for 10 s with further shaking in an ultrasound machine (Branson Sonifier 250) for 30 s to increase the solubility, and then centrifuged for 1 min at 15,700 g (Eppendorf centrifuge 5415 R) with the supernatant solution filtered through a 0.2 μm pore filter (Sarorius Minisart) with 500 μL of the remaining solution stored at 4°C temporarily.
Cell viability assay
Counting cell numbers
After seeding the cells at a density of 3 × 105 on a 6 cm Petri dish at 37°C for 16 h, we treated the cells with TH-R for durations of 24, 48 and 72 h. The cells were collected into tubes and washed with PBS twice, and then 0.5 mL TE buffer was added for the collection of the cells. After the cells were centrifuged at 200 g for 5 min, we discarded the supernatant and added 0.95 mL PBS to suspend the pellets, then a 10 μL solution of cell suspension with 10 μL Trypan blue solution was added to count the cell numbers.
MTS assay
We used a CellTiter 96®Aqueous Non-Radioactive Cell Proliferation Assay (Promega, Madison, WI) for the cytotoxic test analysis. After incubating the cells in the 96-well plates (5 × 104 cells/well) for 18 h, the A549 and WI-38 cells were treated with TH-R continuously at 0–8 mg/mL for 48 h. At the end of the process, 20 μL/well MTS/PMS was added and the wells were incubated (2 h, 37°C, in a humidified incubator). The absorbance was analyzed on a VERSAmax microplate reader at 490 nm. Absorbance values are presented as the mean ± SE of three replicates for each treatment.
Cell migration assay
Cell migration assays were performed using a modified Boyden chamber with a 6.5 mm diameter, 10 mm thickness, porous (8 μm) polycarbonate membrane separating the two chambers (Transwell; Costar, Cambridge, MA). Conditioned medium was prepared from A549 cells which were pretreated without or with TH-R (0, 0.25, 0.5, 1, 2, and 4 mg/mL) for 8 h. The culture medium of the bottom chamber was added with 10% FBS-DMEM. Cells were trypsinized, centrifuged, and resuspended at 4 × 105 cells/mL in 0.5% FBS-DMEM. After 8 h of incubation, the cells in the upper well were fixed with methanol and stained with 20% Giemsa solution (Merck, Darmstadt, Germany). The cells that were attached to the lower surface of the polycarbonate filter were counted under a light microscope (magnification ×100). The experiments were performed three times in triplicate.
Telomeric repeat amplification protocol, TRAP assay
TRAP assay was performed as previously described (CitationLiao et al., 2007). The extracted protein 2 μL (50 ng/μL) was combined with 5 μL 10X PCR buffer (100 mM Tris-HCl, pH 9.0, 25 mM MgCl2, 50 mM KCl, 0.1% gelatine), 2 μL 10 mM dNTPs, 2 μL 10 pmol TS (telomerase substrate) primer and Cxa reverse primer, and then incubated with 1 μL Hi-Tag DNA polymerase (5 U/μL) for 30 min at 30°C. The reaction condition (94°C, 1 min; 54°C, 1 min; 72°C 1 min) was repeated for 35 cycles. The PCR product was subjected to electrophoresis through 12% acrylamide gel (2 h, 110 V), and was observed under ethidium bromide (EtBr) stain. Finally, the gel was observed by digital imaging (AlphaImager™200, Alpha Innotech, San Leandrof, California) under UV light with quantitative analysis of the stained density of the bands.
Cell cycle analysis by flow cytometry
Fixation and stain
The cells were pipetted into ice-cold PBS, washed twice and the supernatant was removed. They were then fixed by adding −20°C 70% ethanol solution. The cells were stored at 4°C for 1 day and were washed with ice-cold PBS twice and stained with 1 mL propidium iodide evenly mixed (1% Triton X-100, 0.5 mg/mL RNase A, 4 μg/mL propidium iodide). The DNA was stained with propidium iodide (PI) after incubation for 30 min at room temperature. The cells were filtered out using a 40 μm nylon mesh.
Flow cytometry analysis
The contents of the DNA in the cells was measured by using flow cytometry (FACSCalibur, Becton-Dickinson, San Jose, CA, USA) and analyzed with fluorescence-activated cell sorting (FACS) in which a laser beam (488 nm) is focused on the cells which emit fluorescent light at varying frequencies depending on the quantity of the cells. The red fluorescent light (617 nm) received at a vertical (90°) position was recorded by CELLQuest software (Verity Software House, Inc., Maine, USA). Finally, the phases of the different cell cycles were classified and analyzed statistically by ModFit 3.0 software.
Measuring the human IFN-γ
Following the manufacturer’s instructions, a DuoSet Kit (R&D systems, Minneapolis, MN, USA) was used to dissolve the anti-human IFN-γ captured antibodies (4 μg/mL) in PBS to coat a 96-well plate, which was then incubated overnight. The plate was then washed three times with PBS with 0.05% Tween 20 in order to remove the unfixed antibodies. Blocking buffer (1% BSA and 0.05% NaN3) (300 μL) was added to the wells, and incubated for 1 h at room temperature, then washed again with PBS.
The IFN-γ was diluted by reagent diluent (20 mM Trizma base, 150 mM NaCl, pH 7.2 with 0.1% BSA and 0.05% Tween 20), then incubated at room temperature for 2 h and washed with PBS three times. Streptavidin-HRP was added to the wells and diluted with reagent diluent, then incubated for 20 min in the dark. The reagent was then washed from the wells and tetramethylbenzidine (TMB) (Zymed Laboratories, South San Francisco, CA, USA) added for 10 min. At the end of the reaction, stop solution (2 N H2SO4) was added for 5 min. We measured the amount of light (OD 450 nm) with an ELISA reader for the supernatant solution and converted it to the amount of stimulated IFN-γ.
In vivo tumor xenograft model
For the nude mice xenograft model, 7–8-week-old male immunodeficient nude mice (BALB/c nu/nu mice), 18–22 g in weight, were used. The animal use protocol listed below has been reviewed and approved by the Institutional Animal Care and Use Committee (IACUC) of Chung-Shan Medical University Experimental Animal Center. Mice were housed under pathogen-free conditions with a 12 h light/12 h dark schedule, and fed an autoclaved diet with access to standard rodent chow (Laboratory Rodent Diet 5001, LabDiet, St. Louis, MO). To establish A549 tumor xenografts, mice were injected subcutaneously with 1 × 107 cells mixed with 75 μL A549 plus 75 μL Matrigel (Collaborative Biomedical Products, Bedford, MA). Ten animals were then randomly divided into two groups consisting of five animals each. The first group of animals was fed via an oral gastric tube with a normal diet and served as controls. The animals in group 2 were fed via an oral gastric tube with TH-R solution at 0.1 mL/mice from day 7 after cell implantation, then twice a day from day 11 to day 30. The day of cell implantation was designated as day 0 and tumor growth and tumor appearance were assessed daily after cell injection. Tumor sizes were measured every three days, and tumor volume was calculated by the formula 0.5238 × L × W × H where L is the long diameter (mm), W is the short diameter (mm) and H is the height (mm) of the tumor (CitationTomayko & Reynolds, 1989). After treatment with TH-R solution for 30 days, the mice were sacrificed and the tumor growth curves were analyzed.
Statistics
The data are presented as mean ± standard deviation of triplicate experiments. The symbol * indicates P <0.05 when compared with untreated cells using the Student’s t-test. SPSS 10.0 software (Chicago, IL) was used to perform statistical analyses.
Results
TH-R in the cytotoxic effect in A549 cells
Morphological changes in the A549 lung cancer cell lines and WI-38 normal lung cell lines after treatment with TH-R for 72 h disclosed more significant apoptosis in A549 cells than in WI-38 cells (). Moreover, cell viability by an MTS assay determined that the cytotoxic effect of TH-R was greater in the A549 lung cancer cells than the WI-38 cells, after being treated with various concentrations of TH-R for 72 h. The survival rate of the A549 cells decreased as the concentration of TH-R increased, but the WI-38 cells were not affected ().
Figure 1. (A) Morphological changes of A549 and WI-38 after treatment with TH-R for 72 h; 24 h after plating, cells were exposed to various concentrations of TH-R (0, 2, 4, 8 mg/mL) for 72 h. (B) The cytotoxic effect of TH-R in A549 lung cancer and WI-38 cells for 72 h after treatment with various concentrations of TH-R using a cellular viability assay (MTS assay). (C) The cytotoxic effect of TH-R treatment for 8 h, 24 h and 48 h in A549 cells. The data are presented as mean ± SD of triplicate experiments. * indicates a P <0.05 with Student’s t-test, as compared with untreated cells. Columns, means of three determinations; bars, SD. (* means P <0.05). Each cell treatment concentration as repeated in three separate experiments.
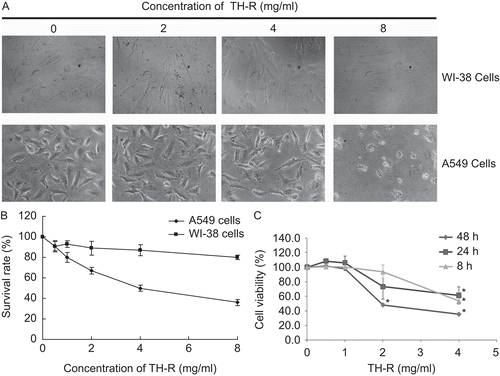
Cell cycle changes by flow cytometry in A549 cells
Cultured A549 cells (5 × 105 cells) were untreated or treated with 1, 2 or 4 mg/mL TH-R for 48 h. The numbers of cells in the G1, S, and G2/M cell cycle phases were assessed by flow cytometry. Cell cycle analysis indicated that in cells untreated and treated with 1, 2 or 4 mg/mL TH-R, the proportion of cells in the G0/G1 phase increased (from 68.01 to 80.15%) and sub-G1 phase (from 0 to 19.55%) and the ratio of cells in the S phase decreased (from 25.18 to 13.7%) (). This result demonstrated that the proliferation of A549 cells was suppressed significantly by TH-R, being associated with increased apoptosis and cell arrest at the G0/G1 to S phase transition.
Evaluation of the effect of TH-R on telomerase activity in A549 cells
A549 cells were treated with varying concentrations (0, 0.25, 0.5, 1, 2, and 4 mg/mL) of TH-R for 24 h. Telomerase activity was detected on a TRAP assay and the 36-base pair internal standard was used as a control (). The data are representative of three independent experiments. The results demonstrated that the telomerase activity in A549 cells was suppressed by TH-R under concentrations of 2 and 4 mg/mL.
Figure 3. Evaluation of the effect of TH-R on telomerase activity in A549 cells for 24 h. A549 cells were treated with varying concentrations (0, 0.25, 0.5, 1, 2, and 4 mg/mL) of TH-R for 24 h. Telomerase activity was detected on TRAP assays and the 36-base pair internal standard was used as a control. The data are representative of three independent experiments. NC (negative control, right lane): no telomerase extract was added.
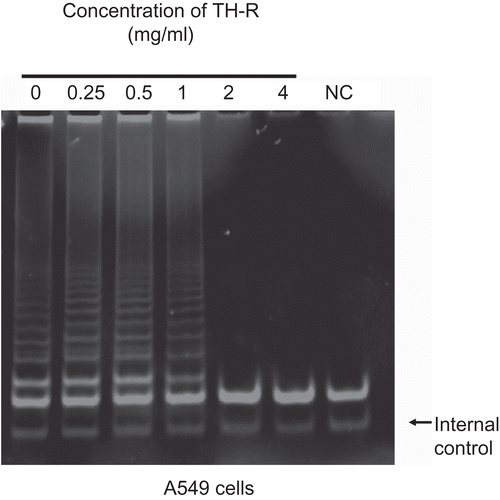
Effect of TH-R in stimulating peripheral lymphocytes to produce human interferon-γ (IFN-γ)
After being treated with various concentrations of TH-R (0, 0.5, 1, 2 and 4 mg/mL) for 24 and 48 h, there was no interferon-γ production of the human cytotoxic T lymphocyte response in vitro, but it could be stimulated by PHA (phytohemagglutinin) as a control ().
Effect of TH-R in the migration of A549 cells
A549 cells were treated with various concentrations (0, 0.25, 0.5, 1, 2, and 4 mg/mL) of TH-R for 8 h and assessed with a Boyden chamber cell migration assay. The higher density in this cell scanning picture is representative of more cells that were passing through (). The results demonstrated that TH-R can suppress the migration of A549 cells.
Figure 5. Effect of TH-R on migration of A549 cells treated for 8 h. A549 cells were treated with various concentrations (0, 0.25, 0.5, 1, 2, and 4 mg/mL) of TH-R and were assessed with a cell migration assay. The higher density in this cell scanning picture is representative of more cells that passed through and adhered to the membrane. After about 8 h, the migrated A549 cells passed through the membrane. (B) They were quantified by counting the cells that migrated onto the membrane. Cells were fixed, stained, and counted as described in the text. The data represent mean ± SD.
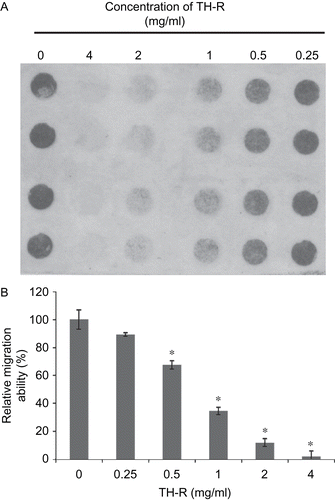
Columns, means of four determinations; bars, SD;*P <0.05). Each cell treatment concentration as repeated in three separate experiments.
Inhibitory effects of TH-R on tumor growth in vivo
Approximately 1 × 107 A549 cells were subcutaneously injected into each flank of the studied mice to initiate tumor growth. Once tumors started to grow, their sizes were measured every three days and the tumor volume was calculated. Seven days after cell implantation, the control group continued to receive sterilized PBS, whereas the experimental animals received TH-R (0.1 mL /mice at the concentration of 180 mg/mL) via gastric tube feeding on day 7, then day 11 and every other day until day 30. Mice from each group were sacrificed every thirty-three days with tumor resection (). Average tumor volumes were measured with different treatments of TH-R and the tumor growth curves were analyzed (). The results demonstrated that there was an initial inhibition of A549 cancer cell growth, but with no statistical difference after one month of therapy.
Figure 6. Inhibitory effects of TH-R on tumor growth in vivo. (A) Approximately 1 × 107 A549 cells were subcutaneously injected into each flank of the studied mice to initiate tumor growth. Once tumors started to grow, their sizes were measured every three days and the tumor volume was calculated. Seven days after cell implantation the control group continued to receive sterilized PBS, whereas the experimental animals received TH-R (0.1 mL/mouse under the concentration of 180 mg/mL) via gastric tube feeding on day 7, then day 11 and every other day until day 30. Mice from each group were sacrificed every thirty-three days with tumor resection. (B) Average tumor volumes were measured with different treatments of TH-R and the tumor growth curves were analyzed. *Illustrates that the treated were significantly different from controls.
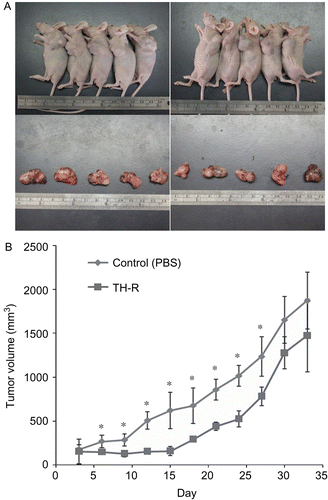
Discussion
It has been reported that extracts from Trichosanthes kirilowii, such as liganan, trichosanthin and chitinase, have positive effects against cancer through preventing or inhibiting the process of carcinogenesis (CitationMoon et al., 2008; CitationXu et al., 2008). A pharmacogenomic analysis of a cancer chemopreventive formulation needs to be considered, and the composition of the formulation should improve over time. Trichosanthins have proved to be effective in inhibiting cancer growth in vitro. Cucurbitacin D isolated from Trichosanthes kirilowii could be a valuable candidate for anti-hepatocarcinoma drug (CitationTakahashi et al., 2009).
Extracts from the roots of Trichosanthes kirilowii induce apoptosis in leukemia cells and glioblastoma multiforme and prolong survival of tumor-bearing mice (CitationLi et al., 2007; CitationYin et al., 2008). The relationship between apoptosis and cancer has been emphasized with an increasing body of evidence, and the induction of tumor cell apoptosis is the basis of many cancer therapies (CitationLee et al., 2006). Our study evaluated the potential anticancer effect of TH-R with increased apoptosis and cell arrest at the G0/G1 phase of lung cancer cells, using cell cycle analysis. TH-R exhibited inhibition of cell growth in a dose- and time-dependent manner by arresting the A549 cells in the G0/G1 phase of the cell cycle and inducing apoptosis. In addition, we found that p53 is important in TH-R-induced apoptosis.
Telomerase, a ribonucleoprotein with reverse transcriptase activity, plays a pivotal role in maintaining telomere length and chromosomal stability in proliferating cells. In cells lacking telomerase activity, replication-associated telomere shortening limits the replicative lifespan. In contrast, high levels of telomerase activity are a hallmark of cancer (CitationWege & Brummendorf, 2007). Telomerase activation is considered to be a critical step in carcinogenesis and its activity correlates closely with human telomerase reverse transcriptase (hTERT) expression. In our study, telomerase activity was determined by TRAP assay. The telomerase activity in cancer cells was suppressed by TH-R treatment. It is a novel finding that TH-R is modified from Tian-hua-fen which can inhibit telomerase activity.
The current state of knowledge regarding IFN-γ calls for promoting host responses to tumors and providing protection against tumor development (CitationIkeda et al., 2002). IFN-γ and lymphocytes not only provide protection against tumor development, but also sculpt the immunogenic phenotype of tumors (CitationBlanck, 2002). In our study TH-R had no effect on stimulating peripheral lymphocytes to produce human IFN-γ, therefore there was no immunomodulatory anticancer effect. Similar to the previous results, trichosanthin could induce the expression of IL-4, one of the major T helper 2 (Th2) cytokines, and inhibit the expression of IFN-r, and important Th1 cytokine both in vivo and in vitro (CitationZhao et al., 2006).
Metastasis is a multistep process during cancer growth that involves attachment to the basement membrane, local proteolysis and migration into the surrounding tissues, lymph, or bloodstream. The ability of cancer cells to migrate and invade involves many gene phenotypes and signal pathways. Our results showed that TH-R in vitro can suppress the migration of A549 cells, and therefore can be a potentially valuable source for anticancer drug discovery.
Although the results of our animal model showed that there was only initial inhibition of cancer cell growth in the first month, a study on the potent antitumor effect of xenografts in vivo may be designed using oral feeding every day to assure the therapeutic effect of the drug. Serum biochemical analysis may be monitored in the future as a drug safety evaluation.
Combinations with traditional chemotherapy or target therapy in animal models of lung cancer should be further investigated to see if there are additive anticancer effects or the possibility of decreased side effects. In order to further investigate the molecular mechanisms inhibiting tumor growth, the possibly targeted gene may be evaluated by gene microarrays.
Conclusion
Based on the results of the anti-lung cancer effect in cell lines and in an animal model, further human lung cancer therapeutic experiments are important to demonstrate the actual responses and survival benefits in clinical practice in randomized controlled studies.
Acknowledgment
Our thanks to Malcolm Higgins (ATS senior editor) for revising the English.
Delcaration of interest
We would like to thank Binshing Pharmaceutical for supplying the Tianhua™ sample and a grant for this study.
References
- Bi L, Li H, Zhang Y (1998): [Effect of trichosanthin of cell cycle and apoptosis of murine melanoma cells]. Zhongguo Zhong Xi Yi Jie He Za Zhi 18: 35–37.
- Blanck G (2002): Components of the IFN-gamma signaling pathway in tumorigenesis. Arch Immunol Ther Exp (Warsz) 50: 151–158.
- Chan DS, Chu LO, Lee KM, Too PH, Ma KW, Sze KH, Zhu G, Shaw PC, Wong KB (2007): Interaction between trichosanthin, a ribosome-inactivating protein, and the ribosomal stalk protein P2 by chemical shift perturbation and mutagenesis analyses. Nucleic Acids Res 35: 1660–1672.
- Hanahan D, Weinberg RA (2000): The hallmarks of cancer. Cell 100: 57–70.
- Ikeda H, Old LJ, Schreiber RD (2002): The roles of IFN gamma in protection against tumor development and cancer immunoediting. Cytokine Growth Factor Rev 13: 95–109.
- Lee HJ, Lee EO, Rhee YH, Ahn KS, Li GX, Jiang C, Lu J, Kim SH (2006): An oriental herbal cocktail, ka-mi-kae-kyuk-tang, exerts anti-cancer activities by targeting angiogenesis, apoptosis and metastasis. Carcinogenesis 27: 2455–2463.
- Li J, Xia X, Ke Y, Nie H, Smith MA, Zhu X (2007): Trichosanthin induced apoptosis in HL-60 cells via mitochondrial and endoplasmic reticulum stress signaling pathways. Biochim Biophys Acta 1770: 1169–1180.
- Li ZH, Nie BM, Chen H, Chen SY, He P, Lu Y, Guo XK, Liu JX (2004): In vitro anti-coxsackievirus B(3) effect of ethyl acetate extract of Tian-hua-fen. World J Gastroenterol 10: 2263–2266.
- Liao CH, Hsiao YM, Sheu GT, Chang JT, Wang PH, Wu MF, Shieh GJ, Hsu CP, Ko JL (2007): Nuclear translocation of telomerase reverse transcriptase and calcium signaling in repression of telomerase activity in human lung cancer cells by fungal immunomodulatory protein from Ganoderma tsugae. Biochem Pharmacol 74: 1541–1554.
- Maraganore JM, Joseph M, Bailey MC (1987): Purification and characterization of trichosanthin. Homology to the ricin A chain and implications as to mechanism of abortifacient activity. J Biol Chem 262: 11628–11633.
- Moon SS, Rahman AA, Kim JY, Kee SH (2008): Hanultarin, a cytotoxic lignan as an inhibitor of actin cytoskeleton polymerization from the seeds of Trichosanthes kirilowii. Bioorg Med Chem 16: 7264–7269.
- Sathesh Kumar S, Ravi Kumar B, Krishna Mohan G (2009): Hepatoprotective effect of Trichosanthes cucumerina var cucumerina L. on carbon tetrachloride induced liver damage in rats. J Ethnopharmacol 123: 347–350.
- Takahashi N, Yoshida Y, Sugiura T, Matsuno K, Fujino A, Yamashita U (2009): Cucurbitacin D isolated from Trichosanthes kirilowii induces apoptosis in human hepatocellular carcinoma cells in vitro. Int Immunopharmacol 9: 508–513.
- Tomayko MM, Reynolds CP (1989): Determination of subcutaneous tumor size in athymic (nude) mice. Cancer Chemother Pharmacol 24: 148–154.
- Wang YY, Ouyang DY, Zheng YT (2007): [Mechanism of trichosanthin against human leukemia/lymphoma cells in vitro]. Zhongguo Shi Yan Xue Ye Xue Za Zhi 15: 729–732.
- Wege H, Brummendorf TH (2007): Telomerase activation in liver regeneration and hepatocarcinogenesis: Dr. Jekyll or Mr. Hyde? Curr Stem Cell Res Ther 2: 31–38.
- Wu TC, Lin P, Hsu CP, Huang YJ, Chen CY, Chung WC, Lee H, Ko JL (2003): Loss of telomerase activity may be a potential favorable prognostic marker in lung carcinomas. Lung Cancer 41: 163–169.
- Xu L, Wang Y, Wang L, Gao Y, An C (2008): TYchi, a novel chitinase with RNA N-glycosidase and antitumor activities. Front Biosci 13: 3127–3135.
- Yin D, Wakimoto N, Xing H, Lu D, Huynh T, Wang X, Black KL, Koeffler HP (2008): Cucurbitacin B markedly inhibits growth and rapidly affects the cytoskeleton in glioblastoma multiforme. Int J Cancer 123: 1364–1375.
- Zhang C, Gong Y, Ma H, An C, Chen D, Chen ZL (2001): Reactive oxygen species involved in trichosanthin-induced apoptosis of human choriocarcinoma cells. Biochem J 355: 653–661.
- Zhang K, Xu J, Huang X, Wu L, Wen C, Hu Y, Su Y, Chen Y, Zhang Z (2007): Trichosanthin down-regulated p210Bcr-Abl and enhanced imatinib-induced growth arrest in chronic myelogenous leukemia cell line K562. Cancer Chemother Pharmacol 60: 581–587.
- Zhao S, Wang Y, Wei H (2006): Trichosanthin induced Th2 polarization status. Cell Mol Immunol 3: 297–301.