Abstract
Context: Scutellaria baicalensis Georgi (Labiatae) (SbG), one of the fifty fundamental herbs of Chinese herbology, has been reported to have anti-asthmatic, antifungal, antioxidative, and anti-inflammatory activities.
Objective: This study was designed to determine the protective effects of the extract of SbG against the acrolein-induced oxidative stress in cultured human umbilical vein endothelial cells (HUVEC).
Materials and methods: The MTT reduction assay was employed to determine cell viability. The total cellular glutathione (GSH) level was detected using a colorimetric GSH assay kit. Cellular GSH production was conducted by detecting the mRNA expression levels of γ-glutamylcysteine ligase catalytic subunit and modifier subunit.
Results: Concentration-dependent cytotoxic effects of acrolein were observed while SbG could effectively protect the acrolein-induced oxidative damage. The protective mechanism was investigated, showing that the increased GSH content in the SbG-incubated HUVE cells was associated with the protective effects of SbG-treated cells. Further RT-PCR data confirmed the elevated mRNA expressions of GSH synthesis enzymes.
Discussion and conclusion: The current study strongly indicated that SbG could be a potential antioxidant against oxidative stress in treating cardiovascular diseases.
Introduction
Acrolein is a highly reactive unsaturated aldehydic product of lipid peroxidation that could implicate various degenerative pathogenesis, oxidative DNA damage and mutagenesis and is widely used as a positive control of reactive oxygen species (ROS) experiments (CitationJia et al., 2007; CitationLoPachin et al., 2009; CitationLuo & Shi, 2005; CitationPan et al., 2009; CitationRoy et al., 2009; CitationWang et al., 2009; CitationZitting & Heinonen, 1980). However, protection against acrolein toxicity in endothelial cells by using the aqueous extract of Scutellaria baicalensis Georgi (Labiatae) (SbG) has not been investigated, though SbG has been reported to have anti-asthmatic, anti-fungal, antioxidative, and anti-inflammatory activities (CitationHuang et al., 2006; CitationKoda et al., 1972; CitationWong & Tsang, 2009). Past studies of SbG showed the neuroprotective effects on ROS-induced cytotoxicity in neuronal HT-22 cells, neuroblastoma SH-SY5Y cells and PC12 cells (CitationChoi et al., 2002; CitationGao et al., 2001; CitationShang et al., 2006; CitationWang et al., 2009). SbG contains numerous flavone derivatives and the active components were studied (CitationDing et al., 2009; CitationJia et al., 2007; CitationYu et al., 2007). It is worthwhile to investigate whether the SbG also had protective effects on endothelial cells, which is of significant clinical meaning in treating endothelial cell related diseases.
Materials and methods
Materials
Human umbilical vein endothelial (HUVE) cells were purchased from BD Biosciences (San Jose, CA). The MTT kit was purchased from Invitrogen (Calscard, CA). Glutathione (GSH) and glutathione S-transferase (GST) kits were from BioVision (Mountain View, CA) and all the chemicals were purchased from Sigma (St. Louis, MO).
Cell culture and preparation of aqueous extract of SbG
The human umbilical vein endothelial cells were grown to confluence in CS-C medium (Sigma) supplemented with 5% fetal bovine serum (FBS) and 1% penicillin/streptomycin at 37 °C in a humidified atmosphere of 5% CO2. The culture media was changed every week. SbG was purchased from Hangzhou Chinese Pharmaceutical (Zhejiang, China) and extracted as reported (CitationWong & Tsang, 2009; CitationYoon et al., 2009). Briefly, the extractions were started with 50 g of material in 1000 mL of distilled water, boiled for 2 h, filtered through 0.22 µm syringe filter, and then lyophilized with a yield of 28.86% (w/w).
Acrolein exposure and SbG supplementation
When HUVE cells were confluent in the 96-well plate and 8-well cell culture chamber (Fisher scientific, USA), acrolein was dissolved in 1× PBS and loaded onto the cells for 24 h. The protective effects of SbG were studied with the acute toxicity experiment by pretreating the cells with SbG for 24 h.
Cell viability assay
MTT reduction assay was employed to determine cell viability. Briefly, the cells were plated into the 96-well plate. For the SbG protection groups, the appropriate wells in triplicates were loaded with SbG for 24 h. Then the cells were incubated with acrolein in DMEM supplemented with 0.5% FBS at 37 °C in a humidified atmosphere of 5% CO2 for 24 h, after which time the media were discarded, followed by addition to each well of 0.1 mL of fresh medium containing MTT (0.2 mg/mL). The plate was incubated for another 4 h at 37 °C. After solubilizing the formazon crystals by adding DMSO, optical densities were detected at 570 nm using a microplate spectrophotometer (Bio-Tek Instruments, USA).
Glutathione level measurement
The total cellular GSH level was detected by using a colorimetric GSH assay kit (BioVision). This kit makes use of a kinetic enzymatic recycling assay based on the oxidation of GSH by 5, 5′-dithiobis-2-nitrobenzoic acid (DTNB) and glutathione reductase to measure the total GSH content in cells. Addition of NADPH2 initiates the progressive reduction of DTNB by GSH, causing a color increase that is monitored at 405 nm. The rate of color change, typically monitored over a 5 min period, is proportional to the total GSH concentration. This assay is specific for GSH; other thiols do not cause interference in the assay. Cellular GSH content was calculated by using the standard GSH from the kit and expressed as nanomoles of GSH per mg of cellular protein.
GST activity measurement
Cellular GST activity was measured by using a GST fluorometric assay kit (BioVision). Briefly, appropriate samples were prepared in a total of 100 µL volume with GST sample buffer from the kit, including the negative control of 100 µL of GST sample buffer alone and a positive control of 2 µL of GST and 98 µL of sample buffer. All the samples were made in three dilutions and loaded into a 96-well plate in triplicates for each experiment. After adding GSH and substrate mix, the plate was read at Ex/Em 380/460 nm at 30 min time point to compare the fluorescence level of treated versus control samples.
RT-PCR of GCLC and GCLM
Molecular level of cellular GSH production was conducted by detecting the mRNA expression levels of γ-glutamylcysteine ligase catalytic subunit (GCLC) and modifier subunit (GCLM), since the synthesis of GSH requires two ATP-dependent enzymes, γ-glutamylcysteine ligase (GCL) and GSH synthase. GCL is the rate-limiting enzyme in the overall GSH synthesis process which is composed of a catalytic (GCLC) and modulatory (GCLM) subunit. GCLC has the catalytic activity while GCLM could enhance the catalytic activity. Total RNA was extracted using TRIzol reagent (Life Technologies, USA) following the manufacturer’s protocol. RNA was quantitated and 5 μg of the total RNA isolated was reverse transcribed to cDNA at 37°C for 1 h in a 30 μL reaction mix containing 200 U of MMLV Reverse Transcriptase (Promega) as per the manufacturer’s protocol. All the PCR amplifications were done in a 50 μL reaction mix containing 2.5 U Taq DNA Polymerase (Promega, USA) in 1× reaction buffer, 1.5 mM MgCl2, 150 μM dNTP mix, 5 to 25 pmol of forward and reverse primers (Genosys, Sigma) and 3 μL of template cDNA. The primers used in quantitative real-time PCR were GCLC (forward, 5′-GGCGATGAGGTGGAATAC-3′; reverse, 5′-AAAGGGTAGGATGGTTTGG-3′), GCLM (forward, 5′-ATCAAACTCTTCATCATCAAC-3′; reverse, 5′-GATTAACTCCATCTTCAATAGG-3′) and glyceraldehyde 3-phosphate dehydrogenase (GAPDH, forward, 5′-ACAGTCAGCCGCATCTTC-3′; reverse, 5′-GCCCAATACGACCAAATCC-3′). Real-time reactions were conducted on an Applied Biosystems 5700 using a hot start, followed by 40 cycles at 94°C for 15 s, 56°C for 30 s, 72°C for 30 s, and a final extension step at 72°C for 3 min. Analysis of the relative gene expression data was performed using the △Ct method. For each primer pair, a plot of the log cDNA dilution versus △Ct was generated to validate the quantitative PCR results. The mean quantities of GCLC and GCLM transcripts were normalized based on the mean quantity of the control gene (GAPDH).
Statistical analysis
All data are expressed as mean ± SEM from three independent experiments. Data were analyzed with SPSS. Significant differences were examined using analysis of variance (ANOVA) and Student’s t-test was used to determine the difference between the two groups and the paired t-test for within-group differences. A value of P <0.05 was considered statistically significant.
Results
Effects of SbG on cell viability
shows the cell viability of the HUVE cells when they were treated with a series of SbG for 24 h. No significant cytotoxicity was observed when SbG was below 100 µg/mL, but the cell viability was significantly decreased when the SbG was over 200 µg/mL, and no dose-response relationship was observed. In the following cotreatment of SbG, 50 and 100µg/mL were employed for the subsequent experiments.
Figure 1. Effects of SbG on HUVE cell viability and cytotoxic effect of acrolein on HUVE cells. The cells were treated with SbG or acrolein for 24 h at appropriate concentrations. MTT was conducted for the cell viability assay. Data were presented as mean±SEM of results in three independent experiments. Each experiment was performed in triplicate. *P< 0.05, **P <0.001 versus control (black bar).
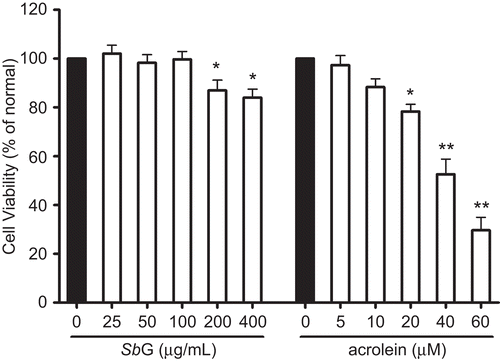
Effects of acrolein on cell viability
HUVE cell viability in the presence of acrolein is shown in . Acrolein below 10 µM for 24 h had no effect on the cell viability. However, significant decreased cell viability was observed when the concentration was over 20 µM and there was a concentration-dependent manner in the cytotoxicity when the cells were incubated with acrolein for 24 h ().
Protective effects of SbG on acrolein-induced cytotoxicity in HUVE cells
In order to elucidate the protective role of SbG against acrolein-induced cytotoxicity, HUVE cells were cultured in the 96-well plate at the density of 5 × 104 for each well. SbG at different concentrations were loaded into the appropriate wells and incubated for 24 h followed by another 24 h incubation with acrolein at the serial concentrations. The MTT cell viability results showed that SbG at 50 and 100 µg/mL could significantly improve the cell viability when treated with acrolein at 20 µM; Interestingly, SbG at 100 µg/mL had better protection than SbG at 50 µg/mL when acrolein concentrations were 40 and 60 µM, respectively (P <0.01 and P <0.05, respectively, ).
Figure 2. Protective effects of SbG pretreatment at 50 and 100 μg/ml showing the protection against acrolein-induced cytotoxicity in the HUVE cells. Cells were plated in the 96-well plate and SbG at different concentrations were loaded into the appropriate wells and incubated for 24 h. The media was discarded and the cells were continuously cultured in the presence or absence of acrolein for another 24 h. MTT was conducted for cell viability. Data were expressed as mean±SEM of the results of three independent experiments. *P < 0.05, **P < 0.01 vs acrolein alone groups.
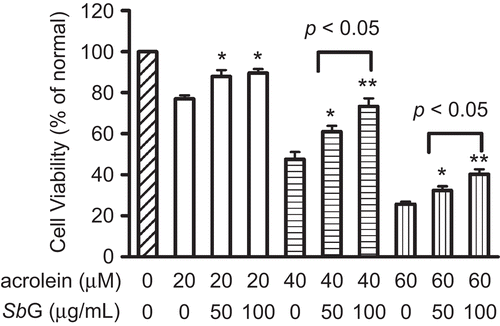
Effects of SbG on cellular GSH level in HUVE cells
When HUVE cells were incubated with SbG at 50 and 100 µg/mL for 24 h, we found that the cellular GSH contents were significantly increased. However, no statistically significant GSH increase was observed between the two concentrations of SbG treatment ().
Figure 3. Effects of SbG on cellular GSH levels and GST activity. HUVE cells were incubated with 50 and 100µg/ml SBG for 24 h. GSH contents were evaluated (*P < 0.05 vs the control). However, no significant GST activity changes were observed in the SbG treated when compared with the control group. Data were presented as mean ±SEM of the results of three independent experiments.
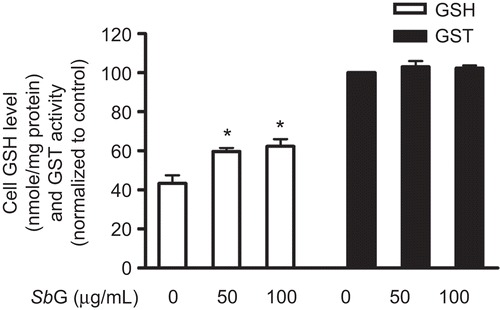
Effects of SbG on GST activity
GST assay was conducted according to the kit instruction (BioVision).The assay utilizes monochlorobimane (MCB), a dye that reacts with GSH. The free form of MCB is almost non-fluorescent, whereas the dye fluoresces blue (Ex/Em = 380/461 nm) when reacting with GSH. GST catalyzes the MCB-GSH reactions and the fluorescence levels are proportional to the amounts of GST presence in the reaction. The sample- and reagent-loaded assay plate was incubated for 2 h at 37°C with gentle rotation. Thirty minutes after loading the substrate mix, the plate was read in Applied Biosystem CytoFluor plate Reader at Ex/Em = 380/460 nm. Our results showed that the cellular GST activities were not significantly changed when comparing the GST activity of SbG pretreated groups (50 and 100 µg/mL for 24 h) with the control group ().
Effects of acrolein on GSH content in HUVE cells
The effects of acrolein on GSH reduction is shown in . HUVE cells were treated with different concentrations of acrolein for 24 h followed by GSH assay. The results showed that acrolein could significantly decrease the cellular GSH contents.
Figure 4. Protective effects of SbG on HUVE cells. The cells were treated with acrolein for 24 h at different concentrations. In the another set of the experiment, the cells were pretreated with 100 µg/mL SbG. Data were presented as mean±SEM of three independent experiments. *P <0.05, ** P < 0.01 versus the control, # P < 0.05, versus the acrolein alone group.
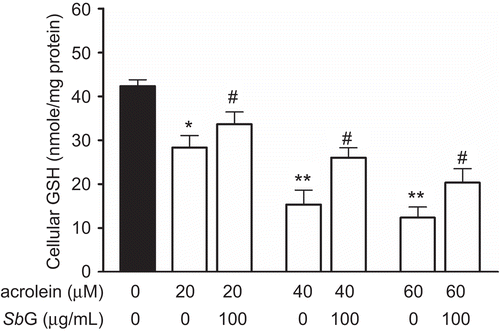
Protective effects of SbG on cellular GSH contents
Because GSH plays an important role in the cellular antioxidant defense (CitationPocernich et al., 2001), we thus investigated the cytoprotective effects of the SbG pretreated on acrolein-induced cytotoxicity in HUVE cells. Briefly, HUVE cells were treated with 50 and 100 µg/mL for 24 h followed by another 24 h of different concentrations of acrolein treatment. GSH assay was performed. As shown in , incubation of the cells with SbG for 24 h could significantly inhibit the GSH decrease.
RT-PCR for GCLC and GCLM
Since GSH synthesis requires two ATP-dependent enzymes of GCL and GSH synthase while GCL is the rate-limiting enzyme including GCLC and GCLM (CitationInoue et al., 2003; CitationLu, 2009; CitationSekhar et al., 2003), we used a sensitive real-time PCR assay to detect their mRNA changes. As shown in , the mRNA levels for both GCLC and GCLM were significantly increased after 24 h incubation of 100 µg/mL SbG, in which GCLC had 2.8-fold elevation. Interestingly, pretreatment of SbG followed by another 24 h incubation of 40 µM acrolein significantly reduced the GCLC mRNA level (P <0.05), while no statistical difference was found for the GCLM mRNA levels after the 24 h acrolein treatment (P >0.05).
Figure 5. After 24 h pretreatment of 100 µg/mL SbG, mRNA levels of both GCLC and GCLM were elevated. Acrolein (40 µM) treatment inhibited the SbG induced GCLC mRNA up-regulation levels. Data were mean±SEM of three independent experiments. *P <0.05, ** P < 0.01 versus the control; # P < 0.05, versus the SBG alone group.
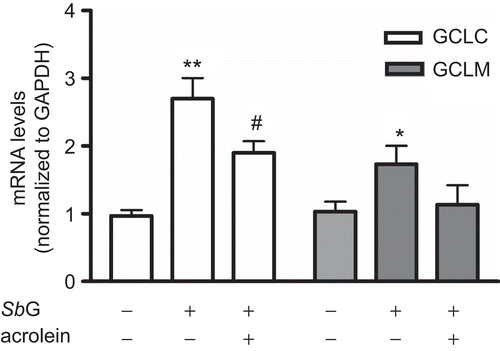
Discussion
Protection against oxidative stress in cardiovascular disorders by using phytotherapy and western medicines has been extensively studied (CitationBanerjee et al., 2003; CitationChen et al., 2009; CitationCosta et al., 2007; CitationCsiszar et al., 2008; CitationHe et al., 2008a; Citation2008b; CitationKobayashi et al., 2002; CitationLakomkin et al., 2005; CitationLiao et al., 2009; CitationNemoto et al., 2007; CitationSaada et al., 2009; CitationVaage et al., 1997; CitationVenardos et al., 2007; CitationYang et al., 1995). HUVE cells have been extensively employed to study cardiovascular diseases (CitationBreymann et al., 2006; CitationChen et al., 2009; CitationErdbrugger et al., 1989; CitationMorikawa et al., 2002). The HUVEC model we used had duplicatable results when they were exposed to the cytotoxic acrolein, which is a major byproduct of oxidative stress and lipid peroxidation. GSH and GST have been suggested to play a crucial role in the detoxification of acrolein (CitationJaeschke et al., 1987; CitationMitchell & Petersen, 1989; CitationMonteil et al., 1999). Although acrolein toxicity has been recently investigated in endothelial cells, herbal extract protection and their molecular mechanism have not been investigated (CitationMisonou et al., 2005; CitationPark & Taniguchi, 2008; CitationPatel & Block, 1993; CitationWu et al., 2006). The current study was aimed to elucidate the protective effects of SbG and explore the possible mechanism. Our data showed that SbG-incubated HUVE cells had higher levels of cellular GSH and the elevated mRNA expressions of GCLC and GCLM, which were the catalytic and modulatory subunits of the GSH synthesis process. Surprisingly, SbG did not affect GST activity when the cells were incubated with SbG for 24 h. The protective effect of SbG was significant when the cells were pretreated with SbG for 24 h, followed by exposing the cells to the cytotoxic acrolein incubation for another 24 h. The protection also showed even at the 50 µg/mL concentration. The present data suggest that SbG could be a potential antioxidant against oxidative stress in cardiovascular diseases.
Declaration of interest
This work was supported by the National Natural Science Foundation of China (30400094). The authors report no conflicts of interest. The authors alone are responsible for the content and writing of the paper.
References
- Banerjee SK, Mukherjee PK, Maulik SK. (2003). Garlic as an antioxidant: The good, the bad and the ugly. Phytother Res, 17, 97–106.
- Breymann C, Schmidt D, Hoerstrup SP. (2006). Umbilical cord cells as a source of cardiovascular tissue engineering. Stem Cell Rev, 2, 87–92.
- Chen Y, Ma D, Huang QL, Zheng W, Zhang J, Shen Y, Li J, Dong W, Lu M, Wang J, Hua ZC. (2009). Expression, purification and characterization of C-FADD. Cell Mol Immunol, 6, 295–301.
- Choi J, Conrad CC, Malakowsky CA, Talent JM, Yuan CS, Gracy RW. (2002). Flavones from Scutellaria baicalensis Georgi attenuate apoptosis and protein oxidation in neuronal cell lines. Biochim Biophys Acta, 1571, 201–210.
- Costa VM, Silva R, Ferreira LM, Branco PS, Carvalho F, Bastos ML, Carvalho RA, Carvalho M, Remiao F. (2007). Oxidation process of adrenaline in freshly isolated rat cardiomyocytes: Formation of adrenochrome, quinoproteins, and GSH adduct. Chem Res Toxicol, 20, 1183–1191.
- Csiszar A, Labinskyy N, Perez V, Recchia FA, Podlutsky A, Mukhopadhyay P, Losonczy G, Pacher P, Austad SN, Bartke A, Ungvari Z. (2008). Endothelial function and vascular oxidative stress in long-lived GH/IGF-deficient Ames dwarf mice. Am J Physiol Heart Circ Physiol, 295, H1882–1894.
- Ding XF, Gao HN, Guo XH, Wang ZZ, Yang LH, Guan Y, Shi JS. (2009). [Analysis of the 4th generation Scutellaria baicalensis Georgi with space mutagenesis via FTIR spectroscopy]. Guang Pu Xue Yu Guang Pu Fen Xi, 29, 1286–1288.
- Erdbrugger W, Bauch HJ, Karbowski B, Kehrel B, Hauss WH. (1989). Alterations in prostaglandin metabolism of cultured human umbilical vein endothelial cells affected by arteriosclerotic risk factors during pregnancy. Prog Clin Biol Res, 301, 389–394.
- Gao Z, Huang K, Xu H. (2001). Protective effects of flavonoids in the roots of Scutellaria baicalensis Georgi against hydrogen peroxide-induced oxidative stress in HS-SY5Y cells. Pharmacol Res, 43, 173–178.
- He H, Liu Q, Shi M, Zeng X, Yang J, Wu L, Li L. (2008a). Cardioprotective effects of hydroxysafflor yellow A on diabetic cardiac insufficiency attributed to up-regulation of the expression of intracellular calcium handling proteins of sarcoplasmic reticulum in rats. Phytother Res, 22, 1107–1114.
- He H, Shi M, Yang J, Zeng X, Qiao H, Wu L, Li L. (2008b). The correlation between angiogenesis and abnormal expression of SERCA2a, phospholamban and the endothelin pathway in heart failure, and improvement by puerarin. Phytother Res, 22, 948–956.
- Huang WH, Lee AR, Yang CH. (2006). Antioxidative and anti-inflammatory activities of polyhydroxyflavonoids of Scutellaria baicalensis GEORGI. Biosci Biotechnol Biochem, 70, 2371–2380.
- Inoue Y, Tomisawa M, Yamazaki H, Abe Y, Suemizu H, Tsukamoto H, Tomii Y, Kawamura M, Kijima H, Hatanaka H, Ueyama Y, Nakamura M, Kobayashi K. (2003). The modifier subunit of glutamate cysteine ligase (GCLM) is a molecular target for amelioration of cisplatin resistance in lung cancer. Int J Oncol, 23, 1333–1339.
- Jaeschke H, Kleinwaechter C, Wendel A. (1987). The role of acrolein in allyl alcohol-induced lipid peroxidation and liver cell damage in mice. Biochem Pharmacol, 36, 51–57.
- Jia L, Liu Z, Sun L, Miller SS, Ames BN, Cotman CW, Liu J. (2007). Acrolein, a toxicant in cigarette smoke, causes oxidative damage and mitochondrial dysfunction in RPE cells: Protection by (R)-alpha-lipoic acid. Invest Ophthalmol Vis Sci, 48, 339–348.
- Kobayashi S, Inoue N, Azumi H, Seno T, Hirata K, Kawashima S, Hayashi Y, Itoh H, Yokozaki H, Yokoyama M. (2002). Expressional changes of the vascular antioxidant system in atherosclerotic coronary arteries. J Atheroscler Thromb, 9, 184–190.
- Koda A, Nagai H, Watanabe S, Kiat LH, Katayama S. (1972). [Combined effect of Scutellaria baicalensis Georgi and anti-asthmatic drugs]. Arerugi, 21, 346–359.
- Lakomkin VL, Konovalova GG, Kalenikova EI, Zabbarova IV, Kaminnyi AI, Tikhaze AK, Lankin VZ, Ruuge EK, Kapelko VI. (2005). Changes in antioxidant status of myocardium during oxidative stress under the influence of coenzyme Q10. Biochemistry (Mosc), 70, 79–84.
- Liao Z, Yin D, Wang W, Zeng G, Liu D, Chen H, Huang Q, He M. (2009). Cardioprotective effect of sasanquasaponin preconditioning via bradykinin-NO pathway in isolated rat heart. Phytother Res, 23, 1146–1153.
- LoPachin RM, Gavin T, Petersen DR, Barber DS. (2009). Molecular mechanisms of 4-hydroxy-2-nonenal and acrolein toxicity: Nucleophilic targets and adduct formation. Chem Res Toxicol, 22, 1499–1508.
- Lu SC. (2009). Regulation of glutathione synthesis. Mol Aspects Med, 30, 42–59.
- Luo J, Shi R. (2005). Acrolein induces oxidative stress in brain mitochondria. Neurochem Int, 46, 243–252.
- Misonou Y, Takahashi M, Park YS, Asahi M, Miyamoto Y, Sakiyama H, Cheng X, Taniguchi N. (2005). Acrolein induces Hsp72 via both PKCdelta/JNK and calcium signaling pathways in human umbilical vein endothelial cells. Free Radic Res, 39, 507–512.
- Mitchell DY, Petersen DR. (1989). Metabolism of the glutathione-acrolein adduct, S-(2-aldehydo-ethyl)glutathione, by rat liver alcohol and aldehyde dehydrogenase. J Pharmacol Exp Ther, 251, 193–198.
- Monteil C, Le Prieur E, Buisson S, Morin JP, Guerbet M, Jouany JM. (1999). Acrolein toxicity: Comparative in vitro study with lung slices and pneumocytes type II cell line from rats. Toxicology, 133, 129–138.
- Morikawa S, Takabe W, Mataki C, Kanke T, Itoh T, Wada Y, Izumi A, Saito Y, Hamakubo T, Kodama T. (2002). The effect of statins on mRNA levels of genes related to inflammation, coagulation, and vascular constriction in HUVEC. Human umbilical vein endothelial cells. J Atheroscler Thromb, 9, 178–183.
- Nemoto M, Nishimura R, Sasaki T, Hiki Y, Miyashita Y, Nishioka M, Fujimoto K, Sakuma T, Ohashi T, Fukuda K, Eto Y, Tajima N. (2007). Genetic association of glutathione peroxidase-1 with coronary artery calcification in type 2 diabetes: A case control study with multi-slice computed tomography. Cardiovasc Diabetol, 6, 23–29.
- Pan J, Keffer J, Emami A, Ma X, Lan R, Goldman R, Chung FL. (2009). Acrolein-derived DNA adduct formation in human colon cancer cells: Its role in apoptosis induction by docosahexaenoic acid. Chem Res Toxicol, 22, 798–806.
- Park YS, Taniguchi N. (2008). Acrolein induces inflammatory response underlying endothelial dysfunction: A risk factor for atherosclerosis. Ann N Y Acad Sci, 1126, 185–189.
- Patel JM, Block ER. (1993). Acrolein-induced injury to cultured pulmonary artery endothelial cells. Toxicol Appl Pharmacol, 122, 46–53.
- Pocernich CB, Cardin AL, Racine CL, Lauderback CM, Butterfield DA. (2001). Glutathione elevation and its protective role in acrolein-induced protein damage in synaptosomal membranes: Relevance to brain lipid peroxidation in neurodegenerative disease. Neurochem Int, 39, 141–149.
- Roy J, Pallepati P, Bettaieb A, Tanel A, Averill-Bates DA. (2009). Acrolein induces a cellular stress response and triggers mitochondrial apoptosis in A549 cells. Chem Biol Interact, 181, 154–167.
- Saada HN, Said UZ, Meky NH, Abd El Azime AS. (2009). Grape seed extract Vitis vinifera protects against radiation-induced oxidative damage and metabolic disorders in rats. Phytother Res, 23, 434–438.
- Sekhar KR, Crooks PA, Sonar VN, Friedman DB, Chan JY, Meredith MJ, Starnes JH, Kelton KR, Summar SR, Sasi S, Freeman ML. (2003). NADPH oxidase activity is essential for Keap1/Nrf2-mediated induction of GCLC in response to 2-indol-3-yl-methylenequinuclidin-3-ols. Cancer Res, 63, 5636–5645.
- Shang YZ, Qin BW, Cheng JJ, Miao H. (2006). Prevention of oxidative injury by flavonoids from stems and leaves of Scutellaria baicalensis Georgi in PC12 cells. Phytother Res, 20, 53–57.
- Vaage J, Antonelli M, Bufi M, Irtun O, DeBlasi RA, Corbucci GG, Gasparetto A, Semb AG. (1997). Exogenous reactive oxygen species deplete the isolated rat heart of antioxidants. Free Radic Biol Med, 22, 85–92.
- Venardos KM, Perkins A, Headrick J, Kaye DM. (2007). Myocardial ischemia-reperfusion injury, antioxidant enzyme systems, and selenium: A review. Curr Med Chem, 14, 1539–1549.
- Wang HT, Zhang S, Hu Y, Tang MS. (2009). Mutagenicity and sequence specificity of acrolein-DNA adducts. Chem Res Toxicol, 22, 511–517.
- Wong KS, Tsang WK. (2009). In vitro antifungal activity of the aqueous extract of Scutellaria baicalensis Georgi root against Candida albicans. Int J Antimicrob Agents, 34, 284–285.
- Wu CC, Hsieh CW, Lai PH, Lin JB, Liu YC, Wung BS. (2006). Upregulation of endothelial heme oxygenase-1 expression through the activation of the JNK pathway by sublethal concentrations of acrolein. Toxicol Appl Pharmacol, 214, 244–252.
- Yang CS, Tsai PJ, Chou ST, Niu YL, Lai JS, Kuo JS. (1995). The roles of reactive oxygen species and endogenous opioid peptides in ischemia-induced arrhythmia of isolated rat hearts. Free Radic Biol Med, 18, 593–598.
- Yoon SB, Lee YJ, Park SK, Kim HC, Bae H, Kim HM, Ko SG, Choi HY, Oh MS, Park W. (2009). Anti-inflammatory effects of Scutellaria baicalensis water extract on LPS-activated RAW 264.7 macrophages. J Ethnopharmacol, 125, 286–290.
- Yu K, Gong Y, Lin Z, Cheng Y. (2007). Quantitative analysis and chromatographic fingerprinting for the quality evaluation of Scutellaria baicalensis Georgi using capillary electrophoresis. J Pharm Biomed Anal, 43, 540–548.
- Zitting A, Heinonen T. (1980). Decrease of reduced glutathione in isolated rat hepatocytes caused by acrolein, acrylonitrile, and the thermal degradation products of styrene copolymers. Toxicology, 17, 333–341.