Abstract
Context: Bombax malabaricum DC. (Bombacaceae) is a traditional Chinese herbal medicine used for the treatment of inflammatory conditions, diarrhea, fever, chronic inflammation, catarrhal affection, and as a diuretic. However, little information is available about its antioxidative activity.
Objective: Water, 50% ethanol, and 80% acetone extracts from flowers of B. malabaricum were investigated for their in vitro antioxidant activity in this article for the first time. Then the relationships between antioxidant activity measured by different methods and total phenolic content (TPC) and total flavonoid content (TFC) were established.
Materials and methods: The antioxidant activities of extracts from B. malabaricum flower were investigated including 1,1-diphenyl-2-picrylhydrazyl (DPPH) radical-scavenging activity, oxygen radical absorbance capacity (ORAC), reducing power, and inhibition on phosphatidylcholine liposome peroxidation.
Results: Results showed that all the extracts possessed remarkable antioxidant capacity compared with ascorbic or gallic acids. Total antioxidant activities evaluated by ORAC assay of different extracts ranged from 700.03 to 1482.46 μmol Trolox equivalents/g. The highest TPC of 130.38 mg gallic acid equivalents (GAE)/g was observed in 80% acetone extract, whereas the lowest TPC of 57.09 mg GAE/g was obtained in the water extract. Furthermore, TFC exhibited significant (P < 0.05) positive correlations with DPPH radical-scavenging activity, ORAC, and reducing power.
Discussion and conclusion: These findings demonstrate that the flowers of B. malabaricum have excellent antioxidant activities and thus might be a potential source of natural antioxidants.
Introduction
Numerous physiological and biochemical processes in the human body may produce harmful reactive free radicals such as superoxide anion radicals, hydroxyl radicals, and hydrogen peroxide (CitationHalliwell & Gutteridge, 1999; CitationFinkel & Holbrook, 2000). Various diseases including cancers (CitationAmes et al., 1995), heart disease (CitationDiaz et al., 1997), Parkinson’s disease, and neuronal degeneration such as Alzheimer’s (CitationChristen, 2000) are believed to be correlated with the oxidative damage induced by these free radicals. Therefore, natural plants or foods that have the ability to counteract the damaging effects of free radicals are now widely considered as an effective strategy to reduce oxidative damage and exert a beneficial effect on human health (CitationSun et al., 2009a). The traditional Chinese medicinal plants are good sources of natural antioxidants such as terpenoids, anthocyanins, flavonoids, and other phenolics compounds (CitationOu et al., 2003; CitationCai et al., 2004). The antioxidative components may help the human body to reduce oxidative damage.
Bombax malabaricum DC. (Bombacaceae) is a traditional Chinese herbal medicine. It mainly grows in tropical areas such as Southern China, India, and Northern Australia (CitationWu et al., 2008). This plant has been used widely in both Chinese and Indian traditional medicines for the treatment of inflammatory conditions, diarrhea, fever, chronic inflammation, catarrhal affection, and as diuretic (CitationSaleem et al., 1999; CitationShahat et al., 2003). In China, the edible flowers of B. malabaricum are used not only in medicinal treatment but also in healthy soup recipes. Previous phytochemical investigations of this plant have resulted in the isolation of naphthol and naphthoquinone derivatives, anthocyanins (CitationNiranjan & Gupta, 1973), flavonoids (CitationShahat et al., 2003), several sesquiterpenoids (CitationVijaya et al., 2003), and polysaccharide (CitationAgrawal et al., 1972). However, the bioactivities or pharmacological effects of the B. malabaricum flower remain to be elucidated, and information on the antioxidant activity of flower extract is limited.
Therefore, one objective of the present study was to determine the content of potential antioxidant phytochemicals (e.g., polyphenols) in this flower. The other objective was to compare different solvent extracts (water, 50% ethanol, and 80% acetone extracts) from B. malabaricum flower for their DPPH radical-scavenging activity, oxygen radical absorbance capacity (ORAC), reducing power, and inhibition of phosphatidylcholine liposome peroxidation. The last objective was to establish the relationships between antioxidant activity measured by different methods and total phenolic content (TPC) and total flavonoid content (TFC). Results from this preliminary study will provide a better understanding of the nutritional and health benefits of B. malabaricum flower.
Materials and methods
Reagents
DPPH• (1,1-diphenyl-2-picrylhydrazyl), Trolox (6-hydroxy-2,5,7,8-tetramethylchroman-2-carboxylic acid), fluorescein sodium salt, gallic acid, (+)-catechin, Folin–Ciocalteu reagent, and ascorbic acid standard were purchased from Sigma Chemical Co. (Shanghai, China). 2,2′-Azobis(2-methylpropionamidine)dihydrochloride (AAPH) was from Wako Pure Chemical Industries (Japan). All other chemicals and reagents were of analytical grade.
Plant material
The dried flowers of B. malabaricum were purchased from SanCai Medicine Corporation (Guangzhou, China). Authenticity of the plant species was validated by the specific morphological and anatomical features of the flowers as reported by CitationLi et al. (1978). The dried flowers of B. malabaricum were powdered with a steel blender passed through a 35-mm (42-mesh) sieve, and were kept at −20°C in the dark until use.
Preparation of extracts
Each 50 g powered sample of B. malabaricum flower was extracted with 500 mL of water, 50% ethanol (ethanol:water, 50:50 v/v), or 80% acetone (acetone:water, 80:20, v/v) individually according to the method of CitationKim et al. (2003) with some modifications. The samples were sonicated for 20 min in a bath sonicator (Kunsan KQ-250DE, Shanghai, China), followed by continuous shaking at 150 rpm for 24 h at 30°C. Then the extracts were centrifuged at 10,000 g for 10 min using an Eppendorf 5804R refrigerated bench top centrifuge (Eppendorf, Wesseling-Berzdorf, Germany). After centrifugation, the supernatant was removed and the remaining pellet was repeated by adding fresh solvents. The supernatant was then collected, evaporated under vacuum at 40°C to remove solvents, and finally dried by a vacuum freeze-drier (Christ Alpha 1-2 LD Freeze dryer, Germany). All the extracts were stored at −20°C until analysis. Before the assay, extracts were dissolved at required concentration.
Determination of TPC
TPC in extracts was determined by the Folin–Ciocalteu colorimetric method (CitationSingleton et al., 1999) with some modifications. In brief, 0.5 mL sample, which was prepared by dissolving 10 mg extracts in 10 mL methanol/water (5:5, v/v) solution, was thoroughly mixed with 2 mL ddH2O and 0.5 mL of Folin–Ciocalteu reagent. After 6 min, 5 mL of 70 g/L sodium carbonate (Na2CO3) and 4 mL deionized water were added to the mixture. The reaction mixture was incubated at room temperature for 90 min and then the absorbance was read at 760 nm. In addition, a sample containing only extract solution processed in the same manner to account for any background due to methanol. The results were compared with a calibration curve of the gallic acid standard, which with nine data points between 0 and 200 μg/mL. TPC was expressed as mg GAE/g sample.
Determination of the TFC
TFC was determined by an aluminium colorimetric assay (CitationEnayat & Banerjee, 2009). A standard solution of (+)-catechin at different concentrations (0–100 mg/L) was prepared by dissolving (+)-catechin in ddH2O. The extracts (35 μL) from a 1.0 mg/mL stock solution or standard (+)-catechin solutions (35 μL) were mixed thoroughly by pipetting with 140 μL ddH2O in a 96-well plate. After the addition of 10.5 μL of 5% sodium nitrite (NaNO2), the mixture was incubated for 5 min at 25°C followed by adding 10.5 μL of 10% aluminium chloride (AlCl3). 6 min later, 70 μL 1 M NaOH and ddH2O were added to make the final volume 350 μL. The absorbance was read at 490 nm in a Wallace 1420 multilabel counter VICTOR3 (Perkin Elmer, Singapore) using ddH2O as a blank. TFC was expressed as mg catechin equivalents (CE)/g sample.
Determination of anthocyanin content
Anthocyanin content (AC) of B. malabaricum flower extracts was measured using the pH differential method by CitationSun et al. (2009b). Results were expressed as mg cyanidin-3-glucoside equivalents (CGE)/100 g sample.
DPPH radical-scavenging activity
The DPPH radical-scavenging activity of all the extracts was estimated by the modified method according to CitationEnayat and Banerjee (2009). Ten milligrams of the powder for each extract was dissolved in 1 mL methanol. This original stock solution (10 mg/mL) was further serially diluted with methanol to give a range of concentrations between 0 and 450 μg/mL. These stock solutions (50 μL) were then added to 250 μL of 0.005% methanol DPPH solution in 96-well plates. The DPPH solution was prepared freshly every day. The decrease in absorbance was measured at 490 nm (CitationHwang et al., 2001) at an end point of 30 min after incubation at 25°C in the dark in a 1420 multilabel counter (Perkin Elmer). The normal purple color of DPPH turned into yellow when its single electron was paired with a hydrogen atom coming from a potential antioxidant. Methanol was used as the negative control, and ascorbic acid was used as the positive control. The percentage of DPPH free radical-quenching activity was determined from the formula:
where A0 refers to the absorbance value at 490 nm of the methanol solution of DPPH without extract, A1 is the absorbance of (DPPH + extract), and A2 is the absorbance of the extract only. DPPH radical-scavenging activity is expressed as the 50% inhibition concentration (IC50), that is, the concentration of extracts or pure compounds required for 50% scavenging of DPPH radicals in the specified time period. The lower the IC50 value, the higher the antioxidant activity. Each sample was repeated six times.
ORAC assay
Antioxidant activities of extracts from B. malabaricum flower were evaluated by the ORAC assay according to the method of CitationHuang et al. (2002) with minor modifications. In brief, 20 μL of sample or Trolox standards and 200 μL of 95.6 nM fluorescein in 75 mM phosphate buffer (pH 7.4) were pipetted into a 96-well microplate. The mixture was preincubated for 20 min at 37°C, and then 20 μL of 119.4 mM AAPH was added rapidly using a multichannel pipette. The fluorescence (λexcitation = 485 nm; λemission = 535 nm) was recorded every 4.5 min for 35 cycles using a 1420 multilabel counter (Perkin Elmer). Standard solutions of Trolox were run in each assay. Data were exported to Excel (Microsoft) for further calculations. The relative area under the fluorescence decay curve (AUC) is calculated as AUC = (0.5 × f1/f1 + f2/f1 + f3/f1 + … + fi/f1 + … + f34/f1 + 0.5 × f35/f1) × CT.
where f1 is the first fluorescence reading, fi is the reading at the cycle i, and the CT is cycle time in minutes. Subtract the AUC from the blank to get the net AUC. The final ORAC value is calculated by linear regression between Trolox concentration (μM) and the net AUC. The linear regression is used in the range of 6.25–50 μM Trolox. The final ORAC values were expressed as μmol Trolox equivalents (TE) per gram sample. Each sample was repeated six times.
Reducing power
The reducing power of B. malabaricum flower extracts was assayed as described in CitationOktay et al. (2003). Various concentrations (1 mL) of extract (0–0.8 mg/mL) were mixed with 2.5 mL of phosphate buffer (200 mM, pH 6.6) and 2.5 mL of potassium ferrocyanide [K3Fe(CN)6] (1%, w/v). After incubation at 50°C for 20 min, 2.5 mL of trichloroacetic acid (TCA) (10%, w/v) was added to the mixture before centrifugation at 2790 g for 10 min. Then 2.5 mL of the supernatant was mixed with 2.5 mL ddH2O and 0.5 mL of FeCl3 (0.1%, w/v). The absorbance was measured 10 min later at 700 nm. Ascorbic acid was used as positive control.
Measurement of inhibitory effect on phosphatidylcholine liposome peroxidation
The inhibitory effect of the extracts from B. malabaricum flower on lipid peroxidation was determined according to the thiobarbituric acid (TBA) method (CitationDuh et al., 1999). In this method, malondialdehyde (MDA), a product of the oxidative degradation of polyunsaturated fatty acids, was reacted with TBA to form a diadduct, which can be detected spectrophotometrically at 532 nm. Liposomes were prepared from 300 mg soybean lecithin and 30 mL of 10 mmol/L phosphate buffer (pH 7.4), and sonicated using an ultrasonic cleaner until an opalescent suspension was obtained. The 0.25 mL of B. malabaricum flower extract (0–8 mg/mL) was mixed with 1 mL of sonicated solution (10 mg lecithin/mL) and 0.6 mL of sodium phosphate buffer, then 0.05 mL of 25 mM FeCl3 and 0.05 mL of 25 mM ascorbic acid were added to induce the phosphatidylcholine liposome oxidation. Reaction mixtures were incubated at 37°C for 1 h. At the end of the incubation period, the solution was mixed with TBA (0.4% in 0.2 M HCl) and BHT (0.2% in 95% ethanol) at a ratio of 1:2:0.3 (v/v/v), and then heated at 100°C for 20 min to develop the pink-colored MDA-(TBA)2 adduct. After rapid cooling of the solution, an equal volume of n-butanol was added to extract the MDA-(TBA)2 chromogen by shaking for 5 min. The mixture was centrifuged and the absorbance of the supernatant was recorded at 532 nm. The percentage of inhibitory effect of MDA formation was calculated according to Equation (1), where A0 is the absorbance of the control (without extract), A1 is the absorbance of the extract addition, and A2 is the absorbance without lecithin solution. Gallic acid was used as the positive control.
Statistical analysis
All data were reported as the means ± SD for at least three replications. Analysis of variance (ANOVA) and Pearson’s correlation coefficients were performed to compare the data using SAS 8.0 software package (SAS Institute, Cary, NC).
Results and discussion
Extraction yield
The extraction yields of extractable compounds using different solvents are summarized in . Water (WE), 50% ethanol (EE50), and 80% acetone (AE80) extracts prepared from 50 g B. malabaricum flower gave a yield of 249.6, 276.0, and 212.6 mg extractable compounds (EC) per gram of dried flower, respectively.
Table 1. Yields of extraction, TPCa, TFCb, and ACc in extracts from B. malabaricum flower.
Total phenolic content
shows that all extracts of B. malabaricum flower exhibited noticeable amounts of phenolic compounds. The TPC of different extracts were assayed by the Folin and Ciocalteu method using gallic acid as standard (y = 0.0045x − 0.00526, R2 = 0.9986). The highest TPC of 130.38 ± 0.41 mg GAE/g was obtained in AE80, whereas the lowest TPC of 57.09 ± 1.03 mg gallic acid equivalents (GAE)/g was achieved in WE. The TPC of B. malabaricum flower extracts was much higher than that reported of cranberry (140.9 μmol/g, equivalently 23.82 mg GAE/g) (CitationVinson et al., 2001), and comparable with that reported of black teas (80.5–134.9 mg GAE/g) (Shabnam et al., 2009).
Total flavonoid content
The TFC of different extracts from B. malabaricum flower was assayed by aluminum colorimetric assay using (+)-catechin as standard (y = 0.0031x + 0.00387, R2 = 0.9944). The data presented in indicated that the highest flavonoid content of 86.95 ± 12.75 mg catechin equivalents (CE)/g was obtained in AE80, whereas the lowest flavonoid content of 39.62 ± 2.68 mg CE/g was obtained in WE. In the present study, it was noted that AE80, which was abundant in phenolics, was also abundant in flavonoids (which contribute to the TPC). In addition, the ratio of total flavonoid compounds to the total phenolic compounds (TFC/TPC) ranged from 53.75 to 69.40% (), showing that flavonoids were the major phenolic components existed in the extracts.
Anthocyanin content
As shown in , no anthocyanin compounds were found in the AE80 and EE50. However, WE contained a rather low amount of anthocyanins of 71.33 ± 4.56 mg CGE/100 g.
Generally, there are some anthocyanins present in fresh flowers of B. malabaricum. CitationNiranjan and Gupta (1973) isolated two anthocyanidine glycosides, pelargonidin 5-β-d-glucopyranoside, and cyanidin-7-methylether-3-β-glucopyranoside from the methanol hydrochloric acid (1%) extract of fresh B. malabaricum petals. However, because B. malabaricum has the short flowering time, the flowers after dropping are usually collected and air-dried for storage in traditional Chinese medicinal method. Therefore, the low AC in the extracts of dried flowers from B. malabaricum might be ascribed to the thermal decomposition of anthocyanins during the air-dried treatment.
All these results of TPC, TFC, and AC mentioned earlier indicated that the extract yields and recovery of antioxidant compounds of the plant materials were strongly dependent on the nature of extracting solvent. It is clear that different solvents used for the extraction of plant antioxidant compounds have different abilities due to varied chemical characteristics and polarities of different antioxidant compounds. Polar solvents are frequently employed for the recovery of polyphenols from a plant matrix (CitationSultana et al., 2009). The most suitable of these solvents are aqueous mixtures containing ethanol, methanol, acetone, and ethyl acetate (CitationPeschel et al., 2006). Meanwhile, water is also a widely used solvent, which can extract the major components in vegetables and plants. In the present study, 80% acetone and 50% ethanol showed excellent antioxidant compounds extraction yields from B. malabaricum flower, which was in agreement with the reports that aqueous ethanol and aqueous acetone had good recovery of antioxidant compounds from plant materials (CitationZhao et al., 2006).
DPPH radical-scavenging activity
As shown in , DPPH radical-scavenging activity of nine different concentrations of extracts from B. malabaricum flower was investigated. With the increasing concentration of EE50 from 0 to 450 μg/mL, the scavenging rate of DPPH radical presented a dose-dependent manner (y = 0.1682x + 7.5149, R2 = 0.9738). Furthermore, DPPH radical-scavenging activity was also linearly correlated with the concentrations of AE80 and ascorbic acid in the range of 0 to 150 μg/mL and WE in the range of 0 to 350 μg/mL, respectively. The corresponding correlation coefficients were 0.9780, 0.9883, and 0.9942 for AE80, ascorbic acid, and WE, respectively. But the scavenging rate of DPPH radical presented a plateau after reached 86% in the AE80 or ascorbic acid additional test.
Figure 1. 1,1-Diphenyl-2-picrylhydrazyl (DPPH) radical-scavenging activity of the extracts from B. malabaricum flower. Ascorbic acid was used as positive control. Values are means ± SD (n = 6).

The results of DPPH radical-scavenging activity test were also expressed in the terms of the half-inhibition concentration (IC50) (). The IC50 value was obtained by interpolation from linear regression analysis of the data shown in . The highest DPPH radical-scavenging effect was obtained in the 80AE with the lowest IC50 of 85.17 ± 0.86 μg/mL, followed by EE50 (249.94 ± 1.34 μg/mL) and WE (554.67 ± 3.83 μg/mL), respectively. These results are consistent with the previous observation that B. malabaricum possessed significant radical-scavenging activity and could directly react with and quench stable DPPH radical (CitationShyur et al., 2005). Moreover, the antioxidative property of AE80 of B. malabaricum flower on scavenging DPPH radical was found to be superior to that of Bombax ceiba L. methanol extract (IC50 = 87 μg/mL) (CitationVieira et al., 2009). And AE80 was effective as a DPPH free radical scavenger as ascorbic acid (IC50 = 84.55 μg/mL) (P > 0.05). Therefore, all the three extracts showed potent DPPH radical-scavenging activity, indicating that B. malabaricum flower contained noticeable antioxidant compounds.
Table 2. The 50% inhibition concentration (IC50) for 1,1-diphenyl-2-picrylhydrazyl (DPPH) radical-scavenging activity and lipid peroxidation inhibition, oxygen radical absorbance capacity (ORAC) value of extracts from B. malabaricum flowers.
ORAC value
Recently, the ORAC assay has found to be even a broader valuable and popular method than DPPH for measuring the antioxidant or peroxy radical-scavenging capacity of botanical samples and biological samples (CitationPrior et al., 2000). This assay measures the ability of antioxidant compounds to inhibit the decline of fluorescein disodium that is induced by a peroxyl radical generator, AAPH. In present study, the results of ORAC showed that different solvent extracts from B. malabaricum flower exhibited significantly (P < 0.05) different antioxidant activities under the experimental conditions ().
Similar to the DPPH radical-scavenging activity mentioned earlier (), the 80% acetone was found to be the most effective solvent among the three solvent mixtures. AE80 showed the highest antioxidant activity of 1482.46 ± 220.59 μmol Trolox/g extract, followed by EE50 (887.51 ± 4.46 μmol Trolox/g extract) and WE (700.03 ± 76.01 μmol Trolox/g extract), respectively, indicating that extraction solvent influenced significantly in peroxyl radical-scavenging evaluation. Moreover, although the average ORAC value of WE was somewhat lower than that of ascorbic acid, the difference between them was not statistically significant (P > 0.05). Since there are no published data on the ORAC assay of B. malabaricum flower from previous researches, comparisons were made with some well-known fruits, which have been proved to be great natural antioxidants (CitationWu et al., 2004). Since their results were expressed in μmol Trolox/g fruit, we recalculated our ORAC values into adequate units as well, in order to facilitate the comparison. ORAC values of WE, EE50, and AE80 (174.73 ± 18.97, 244.95 ± 1.23, 315.17 ± 46.90 μmol Trolox/g flower, respectively) were much higher than those of blackberry (53.48 μmol Trolox/g fruit) and blueberry (62.20 μmol Trolox/g fruit).
Positive correlation has been earlier found between ORAC and total phenolic and anthocyanins content in many Chinese herbs (CitationOu et al., 2003; CitationLiao et al., 2008). The strong antioxidant activity of B. malabaricum flower extracts on ORAC is also possibly due to the phenolic compounds and some other compounds. Previous chemical investigations on the flower of B. malabaricum have led to the isolation of flavonoids (CitationShahat et al., 2003), quercetin, kaempferol, glucoside of β-sitosterol, and β-sitosterol. The components mentioned have been proved to be potential natural antioxidants from plants.
Reducing power
In reducing power assay, potential antioxidants reduce the Fe3+/ferricyanide complex to its ferrous form which can then be monitored spectrophotometrically at 700 nm. Increased absorbance of the reaction mixture indicates increased reducing power (CitationZhao et al., 2006). shows the dose–response curves for the reducing power of the extracts from B. malabaricum flower. At a concentration of 0.80 mg/mL, the reducing power of AE80 was 1.298 ± 0.124, whereas at the same concentration, the values of EE50 and WE were only 0.584 ± 0.098 and 0.643 ± 0.039, respectively.
Figure 2. Reducing power of the extracts from B. malabaricum flower. Ascorbic acid was used as positive control. Values are means ± SD (n = 6).
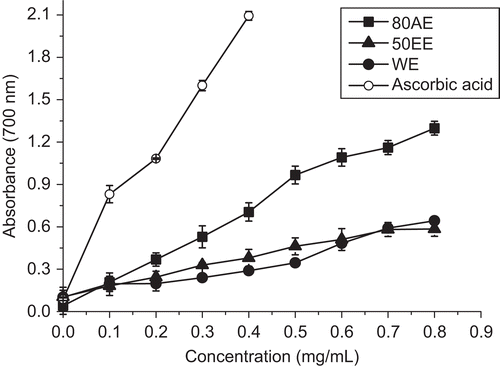
The results showed that AE80 had the highest reducing power among the extracts, followed by EE50 and WE, but the difference between the latter two was not statistically significant (P > 0.05). These observations were partly similar to the observations for DPPH radical-scavenging activities and ORAC, which suggested that 80% acetone was more efficient solvent than other solvent mixtures in reducing power evaluation of B. malabaricum flower.
Moreover, the reducing power values were positively correlated with the concentrations of the three extracts and ascorbic acid in the range of 0–0.8 mg/mL. The corresponding correlation coefficients were 0.9886, 0.9855, 0.9461, and 0.9773 for AE80, EE50, WE, and ascorbic acid, respectively. Although when compared with ascorbic acid, all the tested extracts showed significantly (P < 0.05) lower reducing power, it was evident that the extracts did have ability to reduce Fe(III) to Fe(II). The reducing activities might be due to the presence of flavonoids and polyphenols, which have been found to contribute to antioxidant activity by various reaction mechanisms (CitationZhao et al., 2006).
Inhibition on phosphatidylcholine liposome peroxidation
The inhibitory effect (%) of WE, EE50, and AE80 from B. malabaricum flower on lipid peroxidation compared with gallic acid are shown in . All the extracts demonstrated the ability to inhibit the formation of MDA in a concentration-dependent manner. Among the levels used in the experiment, 8.0 mg/mL WE showed the strongest inhibitory effect on phosphatidylcholine liposome peroxidation with a scavenging rate of 81.13%, higher than that of gallic acid (80.36%) at the same concentration. The inhibitory effects of EE50 and AE80 increased with increasing concentration before reaching 67.26 and 73.83%, respectively, at a concentration of 7.0, 6.0 mg/mL and then remained constant or declined slightly. Nevertheless, the extracts from B. malabaricum flower did retard oxidation of unsaturated fatty acids thus further ascertaining its antioxidative activity. These results are in agreement with CitationVieira et al. (2009) who also reported a high inhibitory effect on soybean liposome peroxidation induced by ascorbyl radicals in a methanol extract of B. ceiba.
Figure 3. The inhibitory effect on phosphatidylcholine liposome peroxidation of the extracts from B. malabaricum flower. Gallic acid was used as positive control. Values are means ± SD (n = 3).
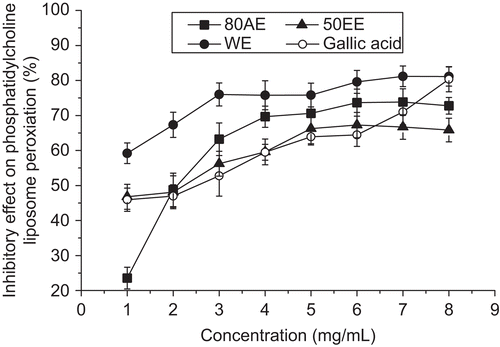
As shown in , it was interesting to find that water appeared to be the most appropriate solvent for extracting antioxidants with a high inhibitory effect on liposome peroxidation. We showed higher inhibitory effect on lipid peroxidation than EE50 and AE80, but its DPPH radical-scavenging activity (), ORAC value (), and reducing power () were weaker than those of the other solvent extracts. This result reflected that there was a relatively low correlation between TPC and inhibitory effect on phosphatidylcholine liposome peroxidation. This may be due to the different types of antioxidants that were assayed by the four methods, where the inhibitory effect on liposome peroxidation only gives an indication of the antioxidative levels of lipophilic compounds (CitationChew et al., 2008); in contrast, the three others give indications of the antioxidant activities of both lipophilic and hydrophilic compounds (CitationMariod et al., 2009).
Relationship between antioxidant activity and phenolic content
From the above results of TPC, DPPH radical-scavenging activity, ORAC, reducing power assay, and inhibitory test on liposome peroxidation, there were good correlations between TPC and antioxidant activities (ranging from 0.6118 to 0.9991, P < 0.05). These findings suggested that TPC was a good predictor of in vitro antioxidant activity. Furthermore, a well positive correlation (0.8856, P < 0.05) between TPC and TFC suggested that flavonoids were the major phenolic components existed in the extracts. The TFC and DPPH radical-scavenging activity, ORAC, and reducing power also showed positive and significant correlation (ranging from 0.8965 to 0.9782). This result is consistent with the previous research reported by CitationPrior et al. (1998) that the major phytochemicals responsible for the antioxidant capacity most likely could be the flavonoid compounds, which are known as secondary natural product metabolites.
Although all those above methods could be used to evaluate the antioxidant activity of B. malabaricum flower, the evaluation results were different. The main reason is that different methods involve distinct reaction mechanisms. On the basis of the chemical reactions involved, major antioxidant capacity assays can be roughly divided into two categories: single electron transfer (ET) reaction-based assays and hydrogen atom transfer (HAT) reaction-based assays (CitationHuang et al., 2005). For the ET-based methods, such as DPPH radical-scavenging activity and ferric reducing/antioxidant power (FRAP), antioxidants are oxidized by oxidants (also the probe) such as DPPH or Fe(III). As a result, a single electron is transferred from the antioxidant molecule to the oxidant (CitationOu et al., 2002). HAT assays, such as ORAC and inhibition of induced low-density lipoprotein peroxidation, generally utilize a thermal radical generator to produce peroxyl radicals that can oxidize the probe. When the test antioxidant is added to these assays, it competes with the probe for the peroxyl radicals, thus inhibiting probe oxidation, thereby allowing determination of the free radical-scavenging activity of the test antioxidant (CitationAlamed et al., 2009).
Due to the different reaction mechanisms of these chemically distinct methods and complexity of the composition of biological antioxidants, it is problematic to use only one or two methods to evaluate antioxidant capacity of them. Therefore, many authors have stressed the need to perform more than one type of antioxidant activity measurement to evaluate the antioxidative property of potential natural antioxidants (CitationKatalinic et al., 2006).
In this study, all the extracts of B. malabaricum flower presented antioxidant activities in the four assays including DPPH radical-scavenging test, ORAC, reducing power assay, and inhibition on phosphatidylcholine liposome peroxidation. These results revealed that the extracts of B. malabaricum flower could not only react as electron or proton donors but also serve as free radical inhibitors or scavengers to terminate the radical chain reaction. On the other hand, it should be pointed out that variations among different extracts prepared with different solvents in each antioxidant activity evaluation are noted in the study. In the other word, extraction solvent mixtures greatly affected the antioxidant activities of different extracts including the DPPH radical-scavenging activity, ORAC, reducing power, and inhibitory effect on liposome peroxidation.
Conclusion
In summary, this study brings attention to the potential of B. malabaricum flower to act as a natural antioxidant source. All extracts of B. malabaricum flower presented good antioxidant activities in aforementioned antioxidant capacity assays regardless they were based on HAT or ET reaction mechanism. However, further research is needed to isolate and identify antioxidative components in the extracts and their effects on antioxidant status in animal models, finally developing applications in food and pharmaceutical industries.
Acknowledgement
The work was supported by the Natural Science Fund of Guandong province, China (No. 9151064101000070).
Declaration of interest
The Authors report no conflicts of interest. The Authors are alone responsible for the content and writing of the paper.
References
- Agrawal GD, Rizvi SA, Gupta PC, Tewari JD. (1972). Study of a polysaccharide from the stamens of Bombax malabaricum flowers. Planta Med 21:293–303.
- Alamed J, Chaiyasit W, McClements DJ, Decker EA. (2009). Relationships between free radical scavenging and antioxidant activity in foods. J Agric Food Chem 57:2969–2976.
- Ames BN, Gold LS, Willett WC. (1995). The causes and prevention of cancer. Proc Natl Acad Sci USA 92:5258–5265.
- Cai Y, Luo Q, Sun M, Corke H. (2004). Antioxidant activity and phenolic compounds of 112 traditional Chinese medicinal plants associated with anticancer. Life Sci 74:2157–2184.
- Chew YL, Lim YY, Omar M, Khoo KS. (2008). Antioxidant activity of three edible seaweeds from two areas in South East Asia. LWT-Food Sci Technol 41:1067–1072.
- Christen Y. (2000). Oxidative stress and Alzheimer disease. Am J Clin Nutr 71:621S–629S.
- Diaz MN, Frei B, Vita JA, Keaney JF Jr. (1997). Antioxidants and atherosclerotic heart disease. N Engl J Med 337:408–416.
- Duh PD, Du PC, Yen GC. (1999). Action of methanolic extract of mung bean hulls as inhibitors of lipid peroxidation and non-lipid oxidative damage. Food Chem Toxicol 37:1055–1061.
- Enayat S, Banerjee S. (2009). Comparative antioxidant activity of extracts from leaves, bark and catkins of Salix aegyptiaca sp. Food Chem 116:23–28.
- Finkel T, Holbrook NJ. (2000). Oxidants, oxidative stress and the biology of ageing. Nature 408:239–247.
- Halliwell B, Gutteridge JMC. (1999). Free Radicals in Biology and Medicine, 3rd ed. Oxford: Oxford University Press.
- Huang D, Ou B, Hampsch-Woodill M, Flanagan JA, Prior RL. (2002). High-throughput assay of oxygen radical absorbance capacity (ORAC) using a multichannel liquid handling system coupled with a microplate fluorescence reader in 96-well format. J Agric Food Chem 50:4437–4444.
- Huang D, Ou B, Prior RL. (2005). The chemistry behind antioxidant capacity assays. J Agric Food Chem 53:1841–1856.
- Hwang BY, Kim HS, Lee JH, Hong YS, Ro JS, Lee KS, Lee JJ. (2001). Antioxidant benzoylated flavan-3-ol glycoside from Celastrus orbiculatus. J Nat Prod 64:82–84.
- Katalinic V, Milos M, Kulisic T, Jukic M. (2006). Screening of 70 medicinal plant extracts for antioxidant capacity and total phenols. Food Chem 94:550–557.
- Kim DO, Jeong SW, Lee CY. (2003). Antioxidant capacity of phenolic phytochemicals from various cultivars of plums. Food Chem 81:321–326.
- Li HL, Liu TS, Huang TC, Koyama T, Devol CE. (1978). Flora of Taiwan, Vol. 5. Taipei, China: Epoch Publishing Co. Ltd.
- Liao H, Banbury LK, Leach DN. (2008). Antioxidant activity of 45 Chinese herbs and the relationship with their TCM characteristics. Evid Based Complement Alternat Med 5:429–434.
- Mariod AA, Ibrahim RM, Ismail M, Ismail N. (2009). Antioxidant activity and phenolic content of phenolic rich fractions obtained from black cumin (Nigella sativa) seedcake. Food Chem 118:120–127.
- Niranjan GS, Gupta PC. (1973). Anthocyanins from the flowers of Bombax malabaricum. Planta Med 24:196–199.
- Oktay M, Gülcin I, Küfrevioglu Ö,. (2003). Determination of in vitro antioxidant activity of fennel (Foeniculum vulgare) seed extracts. LWT-Food Sci Technol 36:263–271.
- Ou B, Huang D, Hampsch-Woodill M, Flanagan JA. (2003). When east meets west: the relationship between yin–yang and antioxidation–oxidation. FASEB J 17:127–129.
- Ou B, Huang D, Hampsch-Woodill M, Flanagan JA, Deemer EK. (2002). Analysis of antioxidant activities of common vegetables employing oxygen radical absorbance capacity (ORAC) and ferric reducing antioxidant power (FRAP) assays: a comparative study. J Agric Food Chem 50:3122–3128.
- Peschel W, Sanchez-Rabaneda F, Dn WPA, Gartzia I, Jimenez D, Lamuela-Raventos RM, Buxaderas S, Condina C. (2006). An industrial approach in the search of natural antioxidants from vegetable and fruit wastes. Food Chem 97:137–150.
- Prior RL, Cao GH, Martin A, Sofic E, McEwen J, O’Brien C, Lischner N, Ehlenfeldt M, Kalt W, Krewer G, Mainland CM. (1998). Antioxidant capacity as influenced by total phenolic and anthocyanin content, maturity, and variety of Vaccinium species. J Agric Food Chem 46:2686–2693.
- Prior RL, Cao G, Prior RL, Cao G. (2000). Analysis of botanicals and dietary supplements for antioxidant capacity: A review. J AOAC Int 83:950–956.
- Saleem R, Ahmad M, Hussain SA, Qazi AM, Ahmad SI, Qazi MH, Ali M, Faizi S, Akhtar S, Husnain SN. (1999). Hypotensive, hypoglycaemic and toxicological studies on the flavonol C-glycoside shamimin from Bombax ceiba. Planta Med 65:331–334.
- Shahat AA, Hassan RA, Nazif NM, Van Miert S, Pieters L, Hammuda FM, Vlietinck AJ. (2003). Isolation of mangiferin from Bombax malabaricum and structure revision of shamimin. Planta Med 69:1068–1070.
- Shyur LF, Tsung JH., Chen JH, Chiu CY, Lo CP. (2005). Antioxidant properties of extracts from medicinal plants popularly used in Taiwan. Int J Appl Sci Eng 3:195–202.
- Singleton VL, Orthofer R, Lamuela-Raventos RM. (1999). Analysis of total phenols and other oxidation substrates and antioxidants by means of Folin–Ciocalteu reagent. Method Enzymol 299:152–178.
- Sultana B, Anwar F, Ashraf M. (2009). Effect of extraction solvent/technique on the antioxidant activity of selected medicinal plant extracts. Molecules 14:2167–2180.
- Sun YP, Chou CC, Yu RC. (2009a). Antioxidant activity of lactic-fermented Chinese cabbage. Food Chem 115:912–917.
- Sun J, Yao J, Huang S, Long X, Wang J, Garcia-Garcia E. (2009b). Antioxidant activity of polyphenol and anthocyanin extracts from fruits of Kadsura coccinea (Lem.) AC. Smith. Food Chem 117:276–281.
- Vieira TO, Said A, Aboutabl E, Azzam M, Creczynski-Pasa TB. (2009). Antioxidant activity of methanolic extract of Bombax ceiba. Redox Rep 14:41–46.
- Vijaya BRM, Kesava RM, Gunasekar D, Marthanda MM, Caux C, Bodo B. (2003). A new sesquiterpene lactone from Bombax malabaricum. Chem Pharm Bull 51:458–459.
- Vinson JA, Su X, Zubik L, Bose P. (2001). Phenol antioxidant quantity and quality in foods: Fruits. J Agric Food Chem 49:5315–5321.
- Wang H, Zeng HP, Zeng Z. (2004). Study on chemical constituents in ethyl acetate fraction of alcoholic extract from the flower of Bombax malabaricum DC. Chem Ind Forest Prod 24:89–91.
- Wu X, Beecher GR, Holden JM, Haytowitz DB, Gebhardt SE, Prior RL. (2004). Lipophilic and hydrophilic antioxidant capacities of common foods in the United States. J Agric Food Chem 52:4026–4037.
- Wu J, Zhang XH, Zhang SW, Xuan LJ. (2008). Three novel compounds from the flowers of Bombax malabaricum. Helv Chm Acta 91:136–143.
- Zhao H, Dong J, Lu J, Chen J, Li Y, Shan L, Lin Y, Fan W, Gu G. (2006). Effects of extraction solvent mixtures on antioxidant activity evaluation and their extraction capacity and selectivity for free phenolic compounds in barley (Hordeum vulgare L.). J Agric Food Chem 54:7277–7286.