Abstract
Context: Phosphonoformate sodium (PFS) has been used as an anti-herpesvirus drug; nevertheless, studies of the use of PFS for treatment of pseudorabies herpesvirus (PrV) infection in the veterinary setting have not been widely reported.
Objective: The present study aimed to analyze the inhibitory effect of PFS on cell infection and apoptosis induced by PrV.
Materials and methods: The infectivity of PrV was determined by plaque assays when PFS was applied to the virus, to the virus-infected cells, and to the cells prior to infection. PCR amplifying DNA polymerase, gE, gG, and gD genes of PrV was performed. PrV-induced cell apoptosis was analyzed by immunofluorescence and flow cytometry.
Results: PFS inhibits cell infection by PrV. Addition of the drug decreased the number of apoptotic cells. Amplification of DNA polymerase and other viral structural genes detected in this study by PCR was reduced, because there were fewer viral DNA copies being made in the presence of the drug. The drug has an inhibitory effect on cell apoptosis induced by PrV.
Discussion and conclusion: PFS has inhibitory effects on cell infection by PrV, which may be used as an anti-PrV agent or combined with other anti-PrV agents. PrV-induced cell apoptotic cells and viral DNA copies decreased in the presence of the PFS.
Keywords::
Introduction
Pseudorabies herpesvirus (PrV) belongs to the subfamily Alphaherpesviridae in the family Herpesviridae and results in pseudorabies (PR), also called Aujeszky’s disease in pig (CitationDavison et al., 2009). PrV infection is often fatal and animals die from central nervous system disorders in susceptible species. PrV can infect most mammals and some avian species, although higher primates including humans are not susceptible to the virus (CitationKlupp et al., 2004). PR poses severe threat to pig industry in most countries in the world. Occurrence of PR has been reported in >20 provinces in China, leading to enormous economic loss (CitationZhao, 2009).
PrV genome is ~150 kb in length and consists of linear, double-stranded, and noncircular permuted DNA molecules. PrV is closely related to the prototypical herpes simplex virus (HSV). Like that of HSV, PrV envelope contains at least 10 different viral envelope glycoproteins, designated glycoprotein B (gB), gC, gD, gE, gH, gI, gK, gL, gM, and gN (CitationFavoreel et al., 2004). It is believed that gE (gI), gC (gIII), gI (gp63), and gG (gX) are not required for viral growth in tissue culture and are therefore designated nonessential (CitationMettenleiter et al., 1986; CitationRauh et al., 1991). In contrast, gB (gll), gD (gp50), gL, and gH (gL and gH are a complex) are thought to be essential for PrV replication (CitationCai et al., 1988; CitationRauh et al., 1991; CitationPeeters et al., 1992).
Vaccination is the main measure in protecting pigs from PrV infection; nevertheless, development of antivirals against PrV may be a complementary strategy in the future. At the same time, as a member of alpha herpesviruses, PrV is a good model virus for antiviral research due to its safety to human. Phosphonoformic acid and its derivatives own a broad spectrum of antiviral activities via noncompeting inhibition of viral DNA or RNA polymerase, interfering the propagation of viruses (CitationOberg, 1989; CitationKedes & Ganem, 1997). Phosphonoformate sodium (PFS), also called foscarnet, is one of the recommended chemical compounds against virus infection. In the current study, we investigated the antiviral effect of PFS on PrV infection in vitro via incubating the drug with viruses, cells, or virus-infected cells. The PCR amplification of DNA polymerase, gD, gE, and gG genes of PrV was comparatively analyzed after drug treatment. Moreover, the inhibitory effect of the drug on cell apoptosis induced by PrV was evaluated by immunofluorescence and flow cytometry. The data are useful for elucidating action mechanisms of PFS on herpesvirus infection.
Materials and methods
Cells and viruses
African green monkey kidney cells (Vero) were maintained in Dulbecco’s modified Eagle’s medium (DMEM) supplemented with 10% newborn bovine serum. The Kaplan strain of PrV was rescued by standard cell transfection procedure using Lipofectamine 2000 transfection reagent (Invitrogen, Carlsbad, CA). The full-length genomic DNA of PrV strain Kaplan was provided by Prof. Lynn Enquist, Princeton University, Princeton, NJ. The PrV was propagated in Vero cells and passaged approximately three times a week.
Cytotoxicity assays
Cytotoxic effect of PFS (Daan, China) on the viability and proliferation of Vero cells was determined by Trypan blue staining and 3-(4,5-dimethylthiazol-2-yl)-2,5-diphenyl tetrazolium bromide (MTT) assays, respectively, as previously described (CitationSui et al., 2010). In brief, the cells in six-well plates were treated with serially diluted PFS (stock concentration 3 g/mL in physiological salt solution) in DMEM at 37°C for 48 h. Then the cells were incubated with 0.5% w/v Trypan blue Mix diluted in phosphate-buffered saline (PBS) at room temperature for 5 min followed by complete washing with PBS. Cell viability was calculated using the formula: (number of unstained cells/total number of cells) × 100 = % viable cells. For MTT assays, the cells in 96-well culture plates were incubated with the drug serially diluted in DMEM (6 wells/each dilution) for 48 h. DMEM-treated cells were served as control. Absorbance of optical density (OD) at a wavelength of 490 nm was read using an ELISA microplate reader. Cell proliferation ability was calculated according to the equation: cell survival rate (%) = (experimental average OD value/control average OD value) × 100%.
Plaque assay
Plaque assays were used to evaluate the infectivity of viruses as previously described (CitationLi et al., 2009; CitationRen et al., 2011a, Citationb). In brief, cells in 24-well plates were infected with serially diluted viruses. The inoculums were replaced with 1% (w/v) methylcellulose in DMEM (1 mL/well) after virus absorption at 37°C for 1 h. Forty-eight hours later, the overlaid medium was removed and the cells were fixed with 3% formalin for 30 min followed by staining with 1% crystal violet (w/v) diluted in 5% ethanol in PBS (200 μL/well) for 20 min. The plaque number was counted and the virus titer in plaque-forming units (pfu) was calculated. All infection assays were performed in 24-well culture plates, unless otherwise indicated. Each assay was performed in triplicate.
Antiviral activity assays
To analyze the effect of PFS on virus per se, PrV (1 × 106 pfu/mL) was incubated with the drug serially diluted in medium at 37°C for 1 h. Then the viruses at a multiplicity of infection (MOI) of 2 were used to infect Vero cells at 37°C for 1 h. After PBS washing, the cells were overlaid with 1% methylcellulose in medium and cultured for 48 h and subjected to plaque assays as above. The protective effect of the drug on cells was analyzed by incubating the cells in 24-well plates with PFS serially diluted in medium at 37°C for 1 h. The cells were then infected with PrV at an MOI of 2 for 48 h and the infectivity of the virus was determined as above. To investigate the effect of PFS on virus-infected cells, the cells were infected with PrV at an MOI of 2 at 37°C for 1 h followed by complete washing with PBS. Then the cells were incubated with serially diluted drugs at 37°C for 48 h and subjected to plaque assays as above.
Viral DNA extraction and PCR
Total DNA was extracted from PrV-infected cells post-PFS treatment using a DNA extraction kit (FastGene, Shanghai, China). Five pairs of primers were designated to amplify genes encoding DNA polymerase, gD, gE, and gG of PrV, as well as a housekeeping gene, glyceraldehyde-3-phosphate dehydrogenase (GAPDH). The information regarding the primer sequence and PCR products is summarized in . The PCR system was composed of 2 µg DNA template (2 μL), 12.5 µL 2× GCbuffer, 4 µL dNTP (10 mM each), 0.5 µL of each primer, 0.25 μL rTaq polymerase (TaKaRa, Shiga, Japan), and 5.25 µL ddH2O. PCR parameters for amplifying the genes of PrV included 94°C for 1 min, 30 cycles of 94°C for 30 sec, 60°C for 30 sec, 72°C for 1 min, and a final extension at 72°C for 10 min. PCR amplification for GAPDH was performed as above, except that the annealing temperature was 57°C. These PCR products were electrophoresed on 1% agarose gel and photographed by using ultraviolet light transilluminator.
Table 1. Primer information used in the study.
Cell apoptosis analysis
Two approaches were used to evaluate the effect of PFS on cell apoptosis as described previously (CitationLi et al., 2009; CitationSui et al., 2010). In brief, cells infected with PFS-treated viruses or PrV-infected cells treated with PFS were cultured at 37°C for 12 h. The MOI of PrV used to infect the cells was 2. Then, the apoptotic cells were analyzed using an Annexin V–FITC kit (Nanjing Keygen Biotech, Nanjing, China) as described previously (CitationLi et al., 2009). Moreover, flow cytometry was used to quantify the number of Annexin V and propidium iodide (PI)-stained cells among different experimental groups. In brief, the cells were treated with the drug as above and trypsinated. The viable cells determined with Trypan blue staining were adjusted to a final density of 4 × 105 cells/mL. Then the cells were incubated with FITC-conjugated anti-Annexin V antibody and PI for 15 min in the dark prior to flow cytometry analysis.
Statistical analysis
The data were analyzed using Student’s t-test, and were expressed as mean value ± standard error of the mean (SEM). The P-value of <0.05 was considered statistically significant.
Results
Maximum nontoxic concentration of PFS
The cell viability indicated by Trypan blue staining was observed under light microscopy. The results showed that the maximum nontoxic concentration of PFS was 480 μg/mL. No cell was stained at 480 μg/mL PFS and some cells were stained at 960 μg/mL PFS (). The nontoxic concentration of the drug was further confirmed by MTT assays ().
Figure 1. Impact of the drug on cell viability and proliferation. The cell viability was evaluated with Trypan blue staining. Mock-treated cells and cells treated with maximum nontoxic concentration or higher concentration of phosphonoformate sodium (PFS) were subjected to Trypan blue staining and a representative comparison is provided. (A) The cell proliferation was determined by MTT colorimetric assays. The cell survival rate under different concentrations of drugs is given and 0.85% NaCl (drug dilution solution) was used as control.(B)
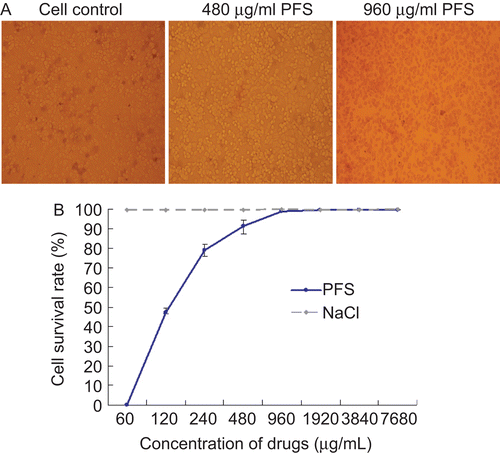
Inhibitory effect of PFS on cell infection by PrV
The incubation between the viruses and the drug led to a decreased infectivity of PrV in a dose-dependent manner. The cell infection by the drug-treated viruses was completely inhibited at the maximum nontoxic concentration (). To analyze the protective effect of PFS on virus-infected cells, the cells were infected with PrV prior to drug treatment. The results showed that PFS inhibited the infectivity of PrV efficiently (). In contrast, pretreatment of cells with the drug did not protect the cells from PrV infection.
Figure 2. Inhibition ability of phosphonoformate sodium (PFS) to cell infection by pseudorabies herpesvirus (PrV). Increased concentrations of PFS were either incubated with PrV for 1 h prior to cell infection or incubated with PrV-infected cells followed by plaque assays. The plaque inhibition rates calculated from drug-treated virus infection group and drug-treated cells pre-infected with viruses are shown in panels A and B, respectively.
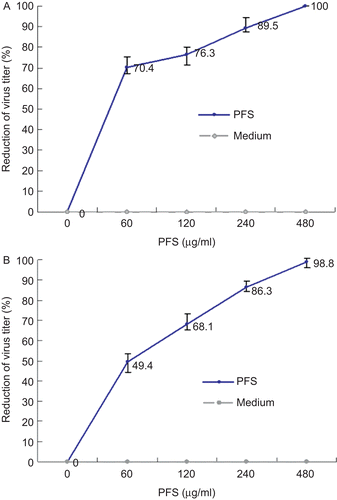
Genome load decreased with the increased concentrations of PFS
Total DNA was extracted and DNA polymerase gene, gD, gE, and gG genes of PrV, as well as the housekeeping gene GAPDH were amplified by PCR, after the virus-infected cells were treated with serially diluted PFS. The results showed that the density of the PCR product bands of these genes was decreased with the increased concentration of the drug gradually. The bands were hardly detectable at 480 μg/mL PFS. The density of the PCR bands of GAPDH was maintained at a relatively stable level (). PCR amplification of these genes from cells infected with drug-treated viruses gave rise to similar results (data not shown).
Figure 3. PCR amplification of pseudorabies herpesvirus (PrV) genes. The cells infected by drug-treated viruses or virus-infected cells treated with the drug were subjected to PCR. The effect of phosphonoformate sodium (PFS) on the amount of amplified DNA polymerase, gD, gE, and gG genes are shown. Housekeeping gene GAPDH is served as an internal reference.
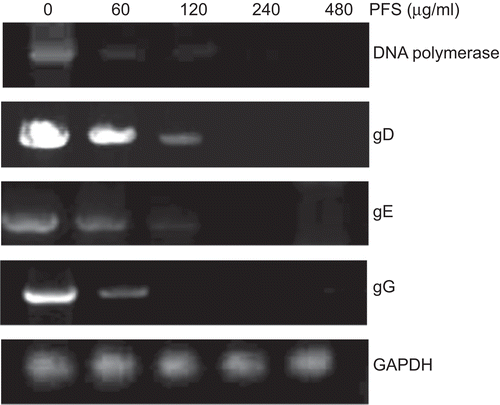
PFS decreased the number of apoptotic cells
Immunofluorescence results showed that addition of PFS to cells infected with drug-treated viruses or virus-infected cells treated with drug resulted in fewer apoptotic cells compared with virus infection control (). The number of apoptotic cells was quantified with flow cytometry. The results showed that there was ~6-fold decrease in the number of apoptotic cells at the maximum nontoxic concentration of drug treatment group compared with virus infection group ().
Figure 4. Effect of the drug on cell apoptosis. The cell apoptosis was analyzed after the cells were infected by drug-treated viruses or virus-infected cells were treated with phosphonoformate sodium (PFS). The multiplicity of infection (MOI) of pseudorabies herpesvirus (PrV) used to infect the cells was 2. (A) The green fluorescence signals are designated as the index of early apoptosis in immunofluorescence analysis. (B) To quantify the cell apoptosis, the cells were stained with Annexin V–FITC and PI, either after they were infected by maximum nontoxic concentration of PFS-treated viruses or virus-infected cells were treated with PFS at maximum nontoxic concentration. The cell apoptosis was analyzed by flow cytometry. Data are presented as dual parameter consisting of Annexin V versus PI (Q2 gate). The early apoptosis (Annexin V–FITC staining) and late apoptosis (PI staining) were reflected in Q4 and Q1 gates, respectively. The apoptosis rates of cells are indicated.
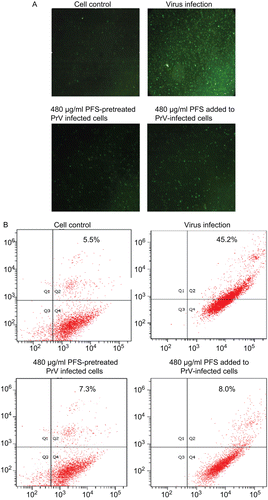
Discussion
PrV, a member of alpha herpesviruses, has been used as a model virus in herpesvirus biology (CitationNaesens & De Clercq, 2001). At the same time, the broad infection spectrum of PrV to most mammals and avian underlines the importance on development of antivirals as a complementary approach to vaccination. In China, some researchers are trying to investigate the effect of combined traditional Chinese medicine (TCM) on PrV infection (CitationRen et al., 2011a, Citationb). Nevertheless, the components of TCM are often complex and their antiviral mechanisms are poorly understood. In the study, we aimed at evaluating the anti-PrV activity of PFS, a chemical compound.
Antiviral assays showed that the drug inhibited the cell infection in a dose-dependent manner, after PrV or PrV-infected cells were treated with PFS. In contrast, the pretreatment of cell with the drug did not show inhibitory effect on PrV infection. Based on the finding, we propagated PFS-treated PrV in susceptible cells (Vero or swine testis cells) continuously (up to 15 passages) and no cell infection was observed (data not shown). The data indicate that PFS can inactivate the virus via unidentified routes. To confirm the conclusion, several important structural genes of PrV were amplified with PCR post-drug treatment. The results showed that the amplification of the genes was negatively related to the concentration of the drug. In other words, the higher the concentration of drug we applied, the weaker PCR bands of the viral structural genes we had. In particular, the synchronized decrease of the PCR bands of PrV structural and nonstructural gene confirms that PFS acts on the virus rather than cells. The DNA polymerase is the early product of virus replication, regulating the assembly and replication of herpesviruses and has been identified as a potential drug target (CitationMcGeoch, 1987; CitationOberg, 1989).
In our study, we confirmed that the DNA polymerase was inhibited by PFS. Since DNA polymerase is not present in virions and expressed only after infection, the effect of the drug on PrV per se needs to be investigated in the future. PFS has interfered with the viral genome load and PCR amplification of these viral genes; however, the GAPDH gene was not affected by the drug treatment. The finding implies that amplification of the genes is reduced, because there are fewer viral DNA copies being made in the presence of the drug. It is known that the DNA polymerase regulates these essential (gD) and nonessential (gE and gG) structural genes of viral replication. The effect of the compound on de novo synthesis of PrV determined by real-time PCR and the impact of PFS on other structural genes of PrV is under investigation in order to clarify related action mechanisms of PFS.
Recently, we confirmed that PrV can induce cell apoptosis (CitationSui et al., 2010). In the current study, we found that PFS inhibited cell infection by PrV, resulting in a decreased number of apoptotic cells. At 12 h post-infection, most infected cells were at a stage of late apoptosis. However, both immunofluorescence and flow cytometry analyses indicate that the inhibitory effect of the drug on cell apoptosis is significant. It has been reported that PFS is able to up-regulate the expression of apoptotic peptidase activating factor 1, also known as APAF1, resulting in cell apoptosis (CitationHofmann et al., 1997). Therefore, it is possible that on one hand, PFS inhibits cell apoptosis via antiviral routines; on the other hand, it fails to inhibit apoptosis of cell per se. It is reported that PFS can inhibit reverse transcriptase of HIV and is also effective as a therapeutic agent for cytomegalovirus and HSV (CitationJacobson et al., 1988; CitationSafrin et al., 1990; CitationDrew, 1992). In this study, we show for the first time that PFS inhibits cell infection by PrV efficiently. The anti-PrV and anti-apoptotic effects of PFS are achieved via inactivation of virions prior to infection and inhibition of viral replication. Inhibitory effect of PFS on PrV infection in vivo is under investigation.
Acknowledgement
We acknowledge funding supported by Program for New Century Excellent Talents in Heilongjiang Provincial University (1155-NCET-005).
Declaration of interest
The authors have no conflict of interest.
References
- Cai WH, Gu B, Person S. (1988). Role of glycoprotein B of herpes simplex virus type 1 in viral entry and cell fusion. J Virol 62:2596–2604.
- Davison AJ, Eberle R, Ehlers B, Hayward GS, McGeoch DJ, Minson AC, Pellett PE, Roizman B, Studdert MJ, Thiry E. (2009). The order Herpesvirales. Arch Virol 154:171–177.
- Drew WL. (1992). Cytomegalovirus infection in patients with AIDS. Clin Infect Dis 14:608–615.
- Favoreel HW, Mettenleiter TC, Nauwynck HJ. (2004). Copatching and lipid raft association of different viral glycoproteins expressed on the surfaces of pseudorabies virus-infected cells. J Virol 78:5279–5287.
- Hofmann K, Bucher P, Tschopp J. (1997). The CARD domain: A new apoptotic signalling motif. Trends Biochem Sci 22:155–156.
- Jacobson MA, Crowe S, Levy J, Aweeka F, Gambertoglio J, McManus N, Mills J. (1988). Effect of foscarnet therapy on infection with human immunodeficiency virus in patients with AIDS. J Infect Dis 158:862–865.
- Kedes DH, Ganem D. (1997). Sensitivity of Kaposi’s sarcoma-associated herpesvirus replication to antiviral drugs. Implications for potential therapy. J Clin Invest 99:2082–2086.
- Klupp BG, Hengartner CJ, Mettenleiter TC, Enquist LW. (2004). Complete, annotated sequence of the pseudorabies virus genome. J Virol 78:424–440.
- Li J, Yin J, Sui X, Li G, Ren X. (2009). Comparative analysis of the effect of glycyrrhizin diammonium and lithium chloride on infectious bronchitis virus infection in vitro. Avian Pathol 38:215–221.
- McGeoch DJ. (1987). The genome of herpes simplex virus: Structure, replication and evolution. J Cell Sci Suppl 7:67–94.
- Mettenleiter TC, Lukàcs N, Thiel HJ, Schreurs C, Rziha HJ. (1986). Location of the structural gene of pseudorabies virus glycoprotein complex gII. Virology 152:66–75.
- Naesens L, De Clercq E. (2001). Recent developments in herpesvirus therapy. Herpes 8:12–16.
- Oberg B. (1989). Antiviral effects of phosphonoformate (PFA, foscarnet sodium). Pharmacol Ther 40:213–285.
- Peeters B, de Wind N, Broer R, Gielkens A, Moormann R. (1992). Glycoprotein H of pseudorabies virus is essential for entry and cell-to-cell spread of the virus. J Virol 66:3888–3892.
- Rauh I, Weiland F, Fehler F, Keil GM, Mettenleiter TC. (1991). Pseudorabies virus mutants lacking the essential glycoprotein gII can be complemented by glycoprotein gI of bovine herpesvirus 1. J Virol 65:621–631.
- Ren X, Sui X, Yin J. (2011a). The effect of Houttuynia cordata injection on pseudorabies herpesvirus (PrV) infection in vitro. Pharm Biol 49:161–166.
- Ren X, Yin J, Li G, Herrler G. (2011b). Cholesterol dependence of pseudorabies herpesvirus entry. Curr Microbiol 62:261–266.
- Safrin S, Assaykeen T, Follansbee S, Mills J. (1990). Foscarnet therapy for acyclovir-resistant mucocutaneous herpes simplex virus infection in 26 AIDS patients: preliminary data. J Infect Dis 161:1078–1084.
- Sui X, Yin J, Ren X. (2010). Antiviral effect of diammonium glycyrrhizinate and lithium chloride on cell infection by pseudorabies herpesvirus. Antiviral Res 85:346–353.
- Zhao X. (2009). Diagnosis and therapy on mixed infection of swine fever, pseudorabies, and Eperythrozoon suis. Shanghai J Animal Husbandry Vet Med 3:98–99 (in Chinese).