Abstract
Context: Oxidative stress plays a key role in pathophysiology of many neurodegenerative disorders such as Alzheimer’s disease, Parkinson’s disease, Huntington’s disease, and so on. Although Matricaria recutita L. (Asteraceae), German chamomile, is traditionally used for central nervous system (CNS)-related diseases, its antistress properties have received little attention.
Objective: The present study evaluated the neuroprotective effect of German chamomile against aluminium fluoride (AlF4−)-induced oxidative stress in rats.
Materials and methods: The Sprague-Dawley rats of either sex (200–250 g) were selected and grouped as: group I received normal saline; group II received AlF4− (negative control); groups III, IV, and V received 100, 200, and 300 mg/kg, orally, German chamomile methanol extract (GCME) along with AlF4−; and group VI received quercetin (25 mg/kg, i.p.) + AlF4−, respectively. After 10 days treatment with GCME, oxidative stress was induced by administering AlF4− through drinking water for 7 days. Then, the protective antioxidant enzyme levels were measured and the histopathological studies were carried out.
Results: The GCME showed dose-dependent neuroprotective activity by significant decrease in lipid peroxidation (LPO) and increase in the superoxide dismutase (SOD), catalase (CAT), glutathione (GSH), and total thiol levels in extract-treated animals as compared with negative control group (P < 0.001). The histopathological studies also revealed the potent neuroprotective action of German chamomile against oxidative brain damage.
Conclusion: The present study for the first time shows potent neuroprotective activity of the methanol extract of German chamomile against AlF4−-induced oxidative stress in rats.
Introduction
Fluoride (F) is utilized in a number of industrial practices and is a ubiquitous ingredient of drinking water, foodstuffs, and dental products (CitationWHO, 2002). However, epidemiological studies have raised the possibility that prolonged exposure to aluminium (Al) in drinking water, perhaps as low as 0.1 ppm, may represent a risk factor for aging-related neurological impairments. In determining the effects of chronic exposure to Al in the drinking water, it is important to consider the factors that enhance or inhibit the bioavailability of Al and its effects on the nervous system (CitationVarner et al., 1998). One such factor is the nature of the Al complex and its speciation after ingestion. In earlier studies, it is hypothesized that when F and Al are both present in drinking water, they will form fluoroaluminium complexes (AlF3) in the stomach that, compared with their ionic forms, exhibit increased transport into bloodstream and likely across the blood–brain barrier (BBB) (CitationVarner et al., 1993). The fluoroaluminium complex has been shown to produce neuronal loss in the CA1 and CA4 areas of the hippocampus when given to animals as 0.5 ppm in drinking water (CitationVarner et al., 1998). The toxic effect may be related to a combination of effects, including impairment of energy-producing enzymes, impaired dephosphorylation of hyperphosphorylated tau-protein, increased neuronal iron concentration, elevated free radical and lipid peroxidation (LPO) levels, and impaired DNA repair, all of which are related to excitotoxicity (CitationBlaylock, 2004).
It is well-known that fluoride is highly active biologically, forming a strong hydrogen bond with the groups found in proteins and nucleic acids (CitationEmsley et al., 1981). Fluoride, as F− in bound form, is known to cross the BBB and accumulate in the brain of rats exposed to chronic high F levels. Some of the neurotoxic effects of F in rats include DNA damage, oxidative stress, decrease in the nicotinic acetylcholine receptors, and histopathological changes in brain cells of rats with decreased learning and memory ability (CitationChirumari & Reddy, 2007). Animal experiments reveal that fluoride increases the uptake of aluminium into the brain at 1 ppm in the drinking water (CitationVarner et al., 1998). Fluoride has been shown to inhibit certain antioxidant enzymes and molecules, such as superoxide dismutase (SOD), glutathione (GSH) reductase, GSH peroxidase, catalase (CAT), and GSH (CitationLi & Cao, 1994). This would not only increase free radical-provoked neuronal injury, but also enhance excitotoxicity, since reactive oxygen species (ROS) as well as nitrogen species and LPO products can trigger the excitotoxic process (CitationSiesjö & Bengtsson, 1989). A growing body of evidence suggests the use of natural antioxidants as therapeutic alternatives against oxidant-induced toxicity (CitationTunali-Akbay et al., 2007). Matricaria recutita L. (Asteraceae), commonly known as German chamomile, is one of the most widely used herbs in the world, especially in children (CitationNoonan et al., 2004). Chamomile is extensively used as a tea or tonic. It is traditionally used to treat anxiety, hysteria, nightmares, insomnia and sleep problems, convulsions, and even delirium tremens (CitationMarten, 1995). German chamomile has also shown antioxidant activity against CCl4-induced liver injury in rats (CitationGupta et al., 2006). The main chemical constituents of the German chamomile are terpenoids including α-bisobolol, chamazulene, sesquiterpenes, and flavonoids such as apigenin, luteolin, and quercetin (CitationNewall et al., 1996).
In our attempt to establish the neuroprotective activity of German chamomile, we previously reported the effectiveness of this plant in global cerebral ischemia/reperfusion-induced neuronal death, a widely accepted model to screen neuroprotective activity (CitationChandrashekhar et al., 2010). In the light of above background, the present study evaluated possible neuroprotective effect of German chamomile against aluminium fluoride (AlF4−)-induced oxidative stress in rats.
Materials and methods
Drugs and chemicals
Trichloroacetic acid (TCA), 2-thiobarbituric acid (TBA), 5,5′-dithiobis (2-nitrobenzoic acid) (DTNB), GSH, and (±)-epinephrine were purchased from Sigma-Aldrich Co., St. Louis, MO. AlF4− was purchased from Hi-Media, Mumbai, India. All other chemicals were of the highest purity.
Collection of plant material
In the present study, capitulas of German chamomile were collected from the National Botanical Research Institute (NBRI), Lucknow, India during the month of June. Herbarium was prepared and the specimen was identified and authenticated by Prof. S. Kappali, Botanist, Department of Botany, Basaveshwar Science College, Bagalkot, Karnataka, India and a voucher specimen (B.Sc./Bot./14/08) was deposited for future reference.
Preparation of plant extract
All capitulas were shade-dried at room temperature until they were free from moisture. Finally, the capitulas were subjected to pulverizer to get coarse powder and then passed through sieve #44 to get uniform powder. The sieved powder was stored in airtight high-density polyethylene container before extraction. The powdered capitulas (600 g) were subjected to successive extraction with petroleum ether (40–60°C) and subsequently with methanol (64–65.5°C). After the residue extraction, solvent was distilled off and excess solvent was completely removed by using a rotatory flash evaporator to get concentrate, then completely dried in freeze-drier and stored in airtight container under refrigeration. The obtained extract (64 g, yield 10.7% w/w) was used for neuroprotective activity.
Determination of LD50
The acute toxicity study was performed as per the method described by CitationLitchfield and Wilcoxon (1949), and LD50 was calculated accordingly. In brief, the German chamomile methanol extract (GCME) in the dose range of 10–2000 mg/kg was administered orally to different groups of mice (n = 10). The animals were examined at every 30 min up to a period of 3 h and then, occasionally for additional period of 4 h; finally, overnight mortality was recorded. All tests on rats were performed at three dose levels: 100, 200, and 300 mg/kg of body weight corresponding to 5, 10, and 15% of LD50 value (2000 mg/kg), respectively.
Experimental animals
The Sprague-Dawley rats of either sex (200–250 g) were obtained from the central animal house of H.S.K. College of Pharmacy and Research Centre, Bagalkot, India. The animals were housed at room temperature (25 ± 1°C) with 50–55% relative humidity and were provided standard laboratory feed (Amrut, Maharashtra, India) and water ad libitum. The study protocol was approved by Institutional Animal Ethics Committee (approval number-HSKCP/IAEC/Clear/2007-08/1). For the present study, animals were randomized into six groups of eight animals each and allowed to acclimatize for 1 week before the experiments.
Experimental design
The animals were divided into six groups of eight rats each as follows:
Group I: normal saline, 10 mL/kg, per os (p.o.)
Group II: AlF4− (negative control)
Group III: 100 mg/kg GCME, p.o. + AlF4−
Group IV: 200 mg/kg GCME, p.o. + AlF4−
Group V: 300 mg/kg GCME, p.o. + AlF4−
Group VI: Quercetin 25 mg/kg, i.p. + AlF4− (reference standard).
Animals from each group were fed with drug or vehicle for 10 days prior to AlF4− treatment through their drinking water (7 days at a dose of 600 ppm).
Preparation of post-mitochondrial supernatant
Following decapitation, the brain was removed and washed in cooled 0.9% saline, kept on ice, and subsequently blotted on filter paper, then weighed and homogenized as 10% w/v in cold phosphate buffer (0.05 M, pH 7.4). The homogenates were centrifuged at 10,000 g for 10 min at 4°C (MPW-350R, Korea) and post-mitochondrial supernatant (PMS) was stored at −80°C freezer until assayed (CitationChandrashekhar et al., 2010).
Biochemical estimation
Lipid peroxidation
Thiobarbituric acid reactive substances (TBARS) in the homogenate were estimated by using standard protocol (CitationPrabhakar et al., 2006). In brief, 0.5 mL of 10% homogenate was incubated with 15% TCA, 0.375% TBA, and 5 N HCl at 95°C for 15 min; the mixture was cooled, centrifuged, and absorbance of the supernatant was measured at 512 nm against appropriate blank. The amount of LPO was determined by using ϵ = 1.56 × 105 per M/cm and expressed as TBARS nmol/mg of protein (CitationBraughler et al., 1987).
Superoxide dismutase
SOD activity was determined based on the ability of SOD to inhibit the autoxidation of epinephrine to adrenochrome at alkaline pH (CitationMisra & Fridovich, 1972). In brief, 25 μL of the supernatant obtained from the centrifuged brain homogenate was added to a mixture of 0.1 mM epinephrine in carbonate buffer (pH 10.2) in a total volume of 1 mL and the formation of adrenochrome was measured at 295 nm. The SOD activity (U/mg of protein) was calculated by using the standard plot.
Catalase
CAT activity was assayed by the method of CitationClaiborne (1985). In brief, the assay mixture consisted of 1.95 mL phosphate buffer (0.05 M, pH 7.0), 1 mL hydrogen peroxide (0.019 M), and 0.05 mL homogenate (10% w/v) in a total volume of 3 mL. Changes in absorbance were recorded at 240 nm. CAT activity was calculated in terms of nM H2O2 consumed/min/mg of protein.
Total thiols
This assay is based on the principle of formation of relatively stable yellow color by sulfhydryl groups with DTNB (CitationMoron et al., 1979). In brief, 0.2 mL of brain homogenate was mixed with phosphate buffer (pH 8), 40 µL of 10 mM DTNB, and 3.16 mL of methanol. This mixture was incubated for 10 min and the absorbance was measured at 412 nm against appropriate blanks. The total thiol content was calculated by using ϵ = 13.6 × 103 per M/cm (CitationSedlak & Lindsay, 1968).
Glutathione
GSH was estimated in various tissues by the method of CitationSedlak and Lindsay (1968). In brief, 5% tissue homogenate was prepared in 20 mM ethylenediaminetetraacetic acid (EDTA), pH 4.7, and 100 μL of the homogenate or pure GSH was added to 0.2 M Tris–EDTA buffer (1.0 mL, pH 8.2) and 20 mM EDTA (0.9 mL, pH 4.7) followed by 20 μL of Ellman’s reagent (10 mmol/L DTNB in methanol). After 30 min of incubation at room temperature, absorbance was read at 412 nm. Samples were centrifuged before the absorbance of the supernatants was measured (CitationKhynriam & Prasad, 2003).
Total protein
Protein concentration in all samples was determined by the method of CitationLowry et al. (1951).
Histopathology
The brains from normal and experimental groups were fixed with 10% formalin and embedded in paraffin wax and cut into longitudinal sections of 5 µm thickness. The sections were stained with hematoxylin and eosin dye for histopathological observation (CitationChandrashekhar et al., 2010).
Statistical analysis
All the data are presented as mean ± SEM. The significance of difference in means between negative control and treated animals for different parameters was determined by using one-way analysis of variance (ANOVA) followed by multiple comparisons Dunnett’s test. A value of P < 0.05 was considered statistically significant.
Results
Determination of LD50
The LD50 of GCME was found to be 2000 mg/kg, p.o. of body weight of rats.
Biochemical estimation
As shown in , brain LPO levels were significantly increased (P < 0.001) in terms of TBARS in AlF4−-treated animals (negative control) as compared with normal group. It is significantly reduced (P < 0.001) in the GCME-treated groups in dose-dependent manner as well as in quercetin-treated group (P < 0.001). The AlF4−-treated group showed a significantly reduced (P < 0.001) levels of SOD, CAT, GSH, and total thiols as compared with the normal group, whereas their levels were significantly increased (P < 0.001) in GCME-treated groups as well as in quercetin-treated group (P < 0.001).
Table 1. Effect of German chamomile methanol extract (GCME) against AlF4−-induced oxidative stress in rats.
Histopathology of rat brain
As shown in , histopathological studies revealed that there was a marked damage observed in the hippocampus region in AlF4−-treated animals. In contrast to the normal brain (), large intracellular space along with significantly increased leukocyte infiltration and marked cellular necrosis were observed in the AlF4−-treated group (). This damage was markedly reversed up to normal in the GCME-treated groups (–) and also in quercetin-treated group ().
Figure 1. Neuroprotective effect of German chamomile methanol extract against AlF4−-induced oxidative brain damage in rats. Photographs of brain sections from different treatment groups stained with hematoxylin and eosin (H&E), 10×. Plates: A: normal; B: AlF4−-treated group; C–E: 100 mg/kg + AlF4−; 200 mg/kg + AlF4− and 300 mg/kg + AlF4−, respectively; and F: quercetin 25 mg/kg + AlF4−. In plate B, AlF4− through the drinking water caused brain damage in rats. There was a marked increase in intracellular space, neutrophil infiltration, and neuronal necrosis observed in the hippocampus region in AlF4−-treated animals (B). There was a significant reversal of damage observed in German chamomile-treated groups (C–E) and also in quercetin-treated group (F). Scale bar: 100 µm.
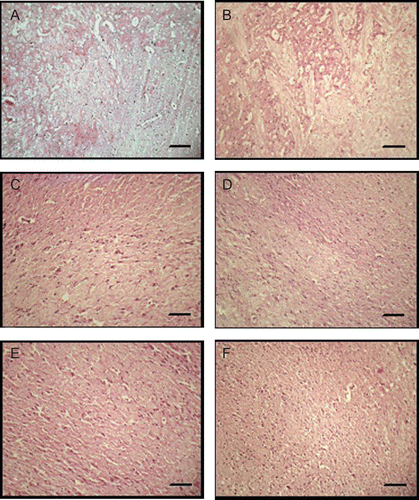
Discussion
In the present study, we observed the therapeutic potential of German chamomile for its neuroprotective action against AlF4−-induced oxidative stress in rat brain. In our studies, the brain damage was produced through administration of AlF4− in drinking water of rats. The severity of oxidative damage to the brain was estimated by measuring LPO, SOD, CAT, GSH, and total thiol levels. The histopathological studies were performed to substantiate these parameters. The results of the study revealed the neuroprotective effect of GCME against the AlF4−-induced oxidative stress as compared with negative control group.
Oxygen is essential for aerobic life, but it is also precursor to the formation of harmful ROS (CitationAlbert & Yong-Mei, 1998). Free radicals in the living organism are generated both enzymatically and non-enzymatically, leading to the formation of ROS. Among all the ROS generated, OH− and OONO− are the most potent and can cause damage to proteins, lipids, and nucleic acids, resulting in the inactivation of some enzyme activities, disruption of ion homoeostasis, and modification of the genetic apparatus and apoptotic death (CitationHalliwell, 1991). A body of evidence suggests fluoride as the toxicant, as its exposure can occur in the workplace and in the environment (CitationChinoy, 2003; CitationSinha et al., 2007). Fluorosis caused by excess intake of fluoride is a slow, progressive degenerative disorder, known to affect predominantly the skeletal muscles, brain, and spinal cord. Recent studies have shown the accumulation of fluoride in the hippocampus of the brain, causing the degeneration of neurons and decreased aerobic metabolism (CitationSinha et al., 2007). It has been reported that during the functioning of the immune system, such as in phagocytosis, reactive oxygen and nitrogen species are generated. If they are left unchecked, they can affect the components of the immune system by inducing oxidative damage (CitationDevasagayam & Sainis, 2002). There is much evidence that superoxide free radicals and LPO plays an important role in fluorosis. Superoxide radicals, ·O2−, are highly reactive and may lead to chemical modification and impairment of proteins, lipids, carbohydrates, and nucleotides in living cells. This toxicity is not only due to an increase in superoxide radicals and lipid peroxides, but also accompanied by a decline in activities of some antioxidant enzymes, viz., SOD, GSH, and CAT, as well as alteration in the levels of reducing substances like GSH and ascorbic acid (CitationChinoy, 2003).
Fluoride has been shown to inhibit SOD, which would increase intracellular levels of the superoxide radicals (CitationVani & Reddy, 2000). Aluminium, another related neurotoxin, is known to produce a dramatic increase in brain free radical generation and LPO both directly and by increasing neuronal and glial iron levels (CitationMundy et al., 1997). Aluminofluoride complexes bind ionically to the terminal oxygen of GDP phosphate. Enzyme-bound GDP or ADP could therefore form a complex with AlF4− that imitates ATP or GTP in its effect on protein confirmation. This effect often causes a structural change that locks the site and prevents the dissociation of the triphosphate. These complexes influence the activity of a variety of phosphatases, phosphorylases, and kinases (CitationBigay et al., 1987).
Interestingly, recent studies report neuroprotective activities of various plant constituents. For instance, CitationShutenko et al. (1999) showed the antioxidant profile of flavonoids as strong basis for a neuroprotective activity in the brain. Further, it has been characterized that changes in brain nitric oxide levels in a model of global ischemia with reperfusion in the presence of quercetin attributed the observed changes to the free radical scavenger action of the flavonoids (CitationShutenko et al., 1999). In consistence with this, apigenin, an important flavonoid present in the German chamomile, showed a neuroprotective activity against acute transient focal cerebral ischemia in rats. Apigenin decreased the glutamate-induced [Ca2+]i increase by inhibiting AMPA, NMDA, and K+, and metabotropic glutamate receptor-induced [Ca2+]i increase in cultured rat hippocampal neuron (CitationLiu et al., 2008) and it may also inhibit the binding of AlF4− complexes to GDP and have membrane-stabilizing activity.
In our previous study, we reported potent neuroprotective action of German chamomile against global model of ischemia (CitationChandrashekhar et al., 2010). This caused us to further establish the effect of German chamomile in another model of cerebral damage.
In the light of above studies, we selected the AlF4−-induced oxidative stress model for evaluation of neuroprotective activity of German chamomile. Our results demonstrated an enhanced LPO level expressed in terms of TBARS and significantly reduced activity of SOD and CAT in the AlF4−-treated group confirming the brain damage due to oxidative stress. The level of LPO is a measure of membrane damage as well as alteration in structure and function of cellular membrane. Chamazulene, an active constituent from chamomile, affects the free radical processes and inhibits LPO in vitro in a concentration- and time-dependent manner (CitationRekka et al., 1996). In accordance with this, the LPO level was significantly decreased in the GCME-treated groups, which may be attributed to the effect of chamazulene. The SOD activity was brought to near-normal after treatment with German chamomile in AlF4−-treated experimental animals. The decreased CAT activity in AlF4−-treated animals may be due to link up to exhaustion of the enzyme because of oxidative stress. The CAT activity was restored to normal after treatment with German chamomile evidently shows the antioxidant properties of the German chamomile against free radicals. Similarly, significantly decreased levels of GSH and total thiols were observed in AlF4−-treated animals. Their levels were restored to near-normal in the German chamomile-treated animals.
Natural products with such properties constitute an ideal choice for maximum therapeutic effect with minimal risk of iatrogenic adverse effects. Consideration with our findings, significantly elevated LPO and depleted protective enzymes (SOD, GSH, CAT and total thiols) in AlF4−-treated groups are thus in correlation with already available reports on the role of oxidative stress in cell injury in general and cerebrovascular disease in particular. The observed results leading to neurodegeneration was confirmed by the histopathological differences between different treatment groups. On the basis of the previous studies, the apigenin and other flavonoids play a role in the probable mechanism by which the German chamomile has normalized the oxidative brain damage may be because of its ability to decrease the glutamate-induced increase in intracellular Ca2+ level, which requires further studies to elucidate the exact mechanism of action.
Conclusion
In conclusion, our results, for the first time, suggest that German chamomile has the potent neuroprotective action against the AlF4−-induced oxidative damage in brain. Further studies are required to find exact molecular mechanism for the neuroprotective action of this plant.
Acknowledgement
We are thankful to Dr. I.S. Muchchandi, Principal and Head, Department of Pharmacology, H.S.K. College of Pharmacy and Research Centre, Bagalkot, Karnataka, India, for providing necessary facilities during the course of this study.
Declaration of interest
The authors report no conflicts of interest. The authors alone are responsible for the content and writing of the paper.
References
- Albert YS, Yong-Mei C. (1998). Oxidative stress and neurodegenerative disorders. J Biomed Sci 5:401–414.
- Bigay J, Deterre P, Pfister C, Chabre M. (1987). Fluoride complexes of aluminium or beryllium act on G-proteins as reversibly bound analogues of the gamma phosphate of GTP. EMBO J 6:2907–2913.
- Blaylock RL. (2004). Excitotoxicity: A possible central mechanism in fluoride neurotoxicity. Fluoride 37:301–314.
- Braughler JM, Chase RL, Pregenzer JF. (1987). Oxidation of ferrous iron during peroxidation of lipid substrates. Biochim Biophys Acta 921:457–464.
- Chandrashekhar VM, Ranpariya VL, Ganapaty S, Parashar A, Muchandi AA. (2010). Neuroprotective activity of Matricaria recutita Linn against global model of ischemia in rats. J Ethnopharmacol 127:645–651.
- Chinoy NJ. (2003). Fluoride stress on antioxidant defense systems. Fluoride 36:138–141.
- Chirumari K, Reddy PK. (2007). Dose-dependant effects of fluoride on neurochemical milieu in the hippocampus and neocortex of rat brain. Fluoride 40:101–110.
- Claiborne A. (1985). Catalase activity. In: Greenwald RA, ed. CRC Handbook of Methods for Oxygen Radical Research. Boca Raton, FL: CRC Press, pp. 283–284.
- Devasagayam TP, Sainis KB. (2002). Immune system and antioxidants, especially those derived from Indian medicinal plants. Indian J Exp Biol 40:639–655.
- Emsley J, Jones DJ, Miller JM, Overill RE, Waddilove RA. (1981). An unexpectedly strong hydrogen bond: ab initio calculations and spectroscopic studies of amide–fluoride systems. J Am Chem Soc 103:24–28.
- Gupta AK, Chitme HR, Dass SK, Misra N. (2006). Antioxidant activity of Chamomile recutita capitula methanolic extracts against CCl4-induced liver injury in rats. J Pharmacol Toxicol 1:101–107.
- Halliwell B. (1991). Reactive oxygen species in living systems: source, biochemistry, and role in human disease. Am J Med 91:14S–22S.
- Khynriam D, Prasad SB. (2003). Changes in endogenous tissue glutathione level in relation to murine ascites tumor growth and the anticancer activity of cisplatin. Braz J Med Biol Res 36:53–63.
- Li J, Cao S. (1994). Recent studies on endemic fluorosis in China. Fluoride 27:125–128.
- Litchfield JT Jr, Wilcoxon F. (1949). A simplified method of evaluating dose–effect experiments. J Pharmacol Exp Ther 96:99–113.
- Liu C, Tu FX, Chen X. (2008). [Neuroprotective effects of apigenin on acute transient focal cerebral ischemia–reperfusion injury in rats]. Zhong Yao Cai 31:870–873.
- Lowry OH, Rosebrough NJ, Farr AL, Randall RJ. (1951). Protein measurement with the Folin phenol reagent. J Biol Chem 193:265–275.
- Marten D. (1995). Chamomile: The herb and the remedy. J Chir Acad Homeopath 6:15–18.
- Misra HP, Fridovich I. (1972). The role of superoxide anion in the autoxidation of epinephrine and a simple assay for superoxide dismutase. J Biol Chem 247:3170–3175.
- Moron A, De Pierre JW, Mannervick B. (1979). Levels of glutathione, glutathione reductase, glutathione-S-transferase activities in rat liver. Biochim Biophys Acta 582:67–68.
- Mundy WR, Freudenrich TM, Kodavanti PR. (1997). Aluminum potentiates glutamate-induced calcium accumulation and iron-induced oxygen free radical formation in primary neuronal cultures. Mol Chem Neuropathol 32:41–57.
- Newall CA, Anderson LA, Phillipson JD. (1996). Herbal Medicines: A Guide for Health-Care Professionals. London: Pharmaceutical Press.
- Noonan K, Arensman RM, Hoover JD. (2004). Herbal medication use in the pediatric surgical patient. J Pediatr Surg 39:500–503.
- Prabhakar KR, Veerapur VP, Parihar KV, Priyadarsini KI, Rao BS, Unnikrishnan MK. (2006). Evaluation and optimization of radioprotective activity of Coronopus didymus Linn. in gamma-irradiated mice. Int J Radiat Biol 82:525–536.
- Rekka EA, Kourounakis AP, Kourounakis PN. (1996). Investigation of the effect of chamazulene on lipid peroxidation and free radical processes. Res Commun Mol Pathol Pharmacol 92:361–364.
- Sedlak J, Lindsay RH. (1968). Estimation of total, protein-bound, and nonprotein sulfhydryl groups in tissue with Ellman’s reagent. Anal Biochem 25:192–205.
- Shutenko Z, Henry Y, Pinard E, Seylaz J, Potier P, Berthet F, Girard P, Sercombe R. (1999). Influence of the antioxidant quercetin in vivo on the level of nitric oxide determined by electron paramagnetic resonance in rat brain during global ischemia and reperfusion. Biochem Pharmacol 57:199–208.
- Siesjö BK, Bengtsson F. (1989). Calcium fluxes, calcium antagonists, and calcium-related pathology in brain ischemia, hypoglycemia, and spreading depression: A unifying hypothesis. J Cereb Blood Flow Metab 9:127–140.
- Sinha M, Manna P, Parames CS. (2007). Aqueous extract of the bark of Terminalia arjuna plays a protective role against sodium-fluoride-induced hepatic and renal oxidative stress. J Nat Med 61:251–260.
- Tunali-Akbay T, Sener G, Salvarli H, Sehirli O, Yarat A. (2007). Protective effects of Ginkgo biloba extract against mercury(II)-induced cardiovascular oxidative damage in rats. Phytother Res 21:26–31.
- Vani LM, Reddy KP. (2000). Effects of fluoride accumulation on some enzymes of brain and gastrocnemius muscle of mice. Fluoride 33:17–26.
- Varner JA, Huie CW, Horvath W, Jensen KF, Isaacson RL. (1993). Chronic AlF3 administration: II. Selected histological observations. Neurosci Res Commun 13:99–104.
- Varner JA, Jensen KF, Horvath W, Isaacson RL. (1998). Chronic administration of aluminum-fluoride or sodium-fluoride to rats in drinking water: Alterations in neuronal and cerebrovascular integrity. Brain Res 784:284–298.
- World Health Organization (WHO). (2002). Environmental Health Criteria. Geneva, Switzerland: Fluoride IPS, pp. 227.