Abstract
Context: Citrus limon (L.) Burms (Rutaceae) has been shown in previous studies to have various biological functions (anti-inflammatory, antiallergic, antiviral, antimutagenic, and anticarcinogenic). However, traditional uses in folk medicine suggest that C. limon may have an effect on the central nervous system (CNS).
Objective: This study investigated the effects of C. limon essential oil (EO) on lipid peroxidation level, nitrite content, glutathione reduced (GSH) concentration, and antioxidant enzymes [superoxide dismutase (SOD), catalase, and glutathione peroxidase (GPx)] activities in mice hippocampus.
Materials and methods: Swiss mice were treated with the suspension of 0.5% Tween 80, in distilled water used as vehicle (i.p., control group) and with EO in three different doses (0.05, 0.1, or 0.15 g/kg, i.p., EO 50, EO 100, and EO 150 groups, respectively). After the treatments, all groups were observed for 24 h. The enzyme activities as well as the lipid peroxidation, nitrite, and GSH concentrations in mice hippocampus were measured using spectrophotometric methods and the results were compared with values obtained from control group.
Results: EO of C. limon treatment significantly reduced the lipid peroxidation level and nitrite content but increased the GSH levels and the SOD, catalase, and GPx activities in mice hippocampus.
Discussion and conclusion: Our findings strongly support the hypothesis that oxidative stress in hippocampus can occur during neurodegenerative diseases, proving that hippocampal damage induced by the oxidative process plays a crucial role in brain disorders, and also imply that a strong protective effect could be achieved using EO of C. limon as an antioxidant.
Introduction
Lemon [Citrus limon (L.) Burms (Rutaceae)] has many important natural chemical components, including citric acid, ascorbic acid, minerals, and flavonoids. Although its health-related properties have always been associated with its vitamin C content, it has recently been shown that flavonoids also play a role in this respect. Some authors suggest that flavonoids have different biological functions, including antioxidative, anti-inflammatory, antiallergic, antiviral, antiproliferative, antimutagenic, and anticarcinogenic activities (CitationElangovan et al., 1994; CitationBenavente-Garcia et al., 1997). Therefore, although the new Citrus cultivars have mainly been selected and developed for fresh consumption, the particular characteristics of their flavonoid contents have led to their use in pharmacological and food technology areas (CitationOrtuño et al., 1997; CitationDel Río et al., 1998).
Lemon fruit contains very important natural chemical components, including phenolic compounds (flavonoids) and other nutrients and non-nutrients (vitamins, minerals, dietary fiber, essential oils (EO), and carotenoids). The health-promoting effects and properties have been associated with the contents of vitamin C and flavonoids, due to their natural antioxidant characteristics (CitationTanaka et al., 1996; CitationRice-Evans et al., 1997). Overall, lemon fruits, rich in flavonoids, are a very important part of a balanced diet, particularly for their role in prevention of diseases (CitationMiyake et al., 1998), such as obesity, diabetes, blood lipid lowering, cardiovascular diseases, and certain types of cancer (CitationMonforte et al., 1995; CitationMiyake et al., 1997). Thus, C. limon EO may possess a modulatory role in the treatment of neurodegenerative diseases, since their phenolic compound can interrupt cellular oxidative processes in the central nervous system (CNS). The effects of EO of C. limon in the CNS have not yet been determined. Therefore, it would be important to conduct these studies in order to clarify its brain action mechanism.
Pharmacological studies with EO of C. limon can reveal its effects on lipid peroxidation level, nitrite content, glutathione reduced (GSH) concentration, and antioxidant enzymes [superoxide dismutase (SOD), catalase, and glutathione peroxidase (Gpx)] activities. Once those effects are established, they may justify the use of this EO in preclinical and clinical studies in the treatment of neurodegenerative diseases. Thus, the aim of this study was to investigate the effects of C. limon EO on lipid peroxidation level, nitrite content, GSH concentration, and antioxidant enzymes activities in mice hippocampus.
Materials and methods
Plant materials
C. limon was identified and collected by Chistiane Mendes Feitosa in February 2010, at the city of Picos, state of Piaui, Brazil; their excicatae was deposited at the Graziella Barroso Herbarium of the Federal University of Piaui under the voucher number 26.453. The sample of C. limon EO leaves were prepared by Laboratory of Chemistry of Natural Products of that University (CitationMatos et al., 1996).
Plant extraction of C. limon EO
Leaves (0.3 kg) from “limoeiro” were collected on the same day and hour, from flowering plants. The same leaf samples collected were steam distilled for 1 h. Oils obtained from these leaves were analyzed (CitationMatos et al., 1996) by GC/MS using a GC-17 A/MS-QP505A (Shimadzu, Japan) instrument under the following conditions: column: dimethylpolysiloxane DB-1 fused-silica capillary column (30 m × 0.25 mm); carrier gas, helium (1 mL/min); injector temperature, 35–180°C at 4°C/min, then 180–250°C at 10°C/min; mass spectra electron impact, 70 eV. Individual components were identified by spectrometric analyses using two computer library MS searches, and Kovacs indices as a preselection aid. Visual mass spectra comparison data from the literature were used for confirmation.
Animals procedures
Male Swiss mice (25–30 g) were used. All animals were maintained at a controlled temperature (23 ± 1°C) and a 12-h dark/light cycle. The EO was emulsified with 0.5% Tween 80. Control animals were treated with a suspension of 0.5% Tween 80, in distilled water used as vehicle. All experiments were performed according to the Guide for the Care and Use of Laboratory Animals, from the US Department of Health and Human Services, Washington DC, 1985. The project was previously approved by the Animal’s Ethics Committee, of the Federal University of Piaui. All doses are expressed in milligrams per kilogram and were administered in a volume of 10 mL/kg injected intraperitoneally (i.p.). In a set of experiments, the treatment with EO [0.05 g/kg (EO 50), 0.1 g/kg (EO 100), or 0.15 g/kg (EO 150)] was delivered orally by gavage after suspension in polyoxyethylenesorbitan monooleate (Tween 80—0.5% v/v in saline; Synth, Brazil) in a constant volume of 10 mL/kg. Mice were treated for 30 consecutive days and 24 h after the drug administration, the animals were sacrificed by decapitation, and the hippocampus was dissected. After dissection, the area was used for determination of lipid peroxidation level, nitrite content, GSH concentration, and antioxidant enzymes. Tests were run between 8:00 a.m. and 6:00 p.m. in an illuminated room with controlled temperature and attenuated sound.
Determination of lipid peroxidation levels and nitrite content
For all of the experimental procedures, 10% (w/v) homogenates of the area of the brain investigated were prepared for all groups. Lipid peroxidation levels in the EO 50 (n = 7), EO 100 (n = 7), EO 150 (n = 7), and control (n = 10) groups were analyzed by method previously described by CitationDraper and Hadley (1990). The results above were expressed as nmol of malondialdehyde (MDA)/g wet tissue.
To determine nitrite contents of EO 50 (n = 7), EO 100 (n = 7), EO 150 (n = 7), and control (n = 10) groups, the 10% (w/v) was determined based on the Griess reaction (CitationGreen et al., 1981). The results above were expressed as nM.
Determination of SOD activity
The hippocampus was ultrasonically homogenized in 1 mL 0.05 M sodium phosphate buffer, pH 7.0. Protein concentration was measured by the method of CitationLowry et al. (1951). The 10% homogenates were centrifuged (800 g, 20 min), and the supernatants were used to assay SOD, catalase, and GPx activities. SOD activity in the EO 50 (n = 7), EO 100 (n = 7), EO 150 (n = 7), and control (n = 10) groups was assayed by using xanthine and xanthine oxidase to generate superoxide radicals (CitationFlohé & Otting, 1984). SOD activity in the samples was determined from this curve, and the results were expressed as U/mg of protein.
Determination of catalase activity
Catalase activity was measured in the EO 50 (n = 7), EO 100 (n = 7), EO 150 (n = 7), and control (n = 10) groups by the method that uses H2O2 to generate H2O and O2 (CitationChance & Maehly, 1955). Protein concentration was measured by the method of CitationLowry et al. (1951). Results are expressed as mmol/min/mg of protein (CitationMaehly & Chance, 1954).
Determination of GPx activity GSH concentration
GPx was measured in the EO 50 (n = 7), EO 100 (n = 7), EO 150 (n = 7), and control (n = 10) groups using the method described by CitationSinet et al. (1975) and the protein concentration was measured according to the method described by CitationLowry et al. (1951). Results are expressed as mU/mg of protein.
GSH concentration was measured in the EO 50 (n = 7), EO 100 (n = 7), EO 150 (n = 7), and control (n = 10) groups using the method described by CitationSedlak and Lindsay (1968). The hippocampus was homogenized in 0.02 M ethylenediaminetetraacetic acid (EDTA). Results are expressed as μg/g tissue wet weight.
Statistical analyses
Results of neurochemical determinations were compared using ANOVA and the Student-Newman-Keuls t-test as post hoc test, because these results show a parametric distribution. In all situations, statistical significance was at P ≤ 0.05. The statistical analyses were performed with the software GraphPad Prism, Version 3.00 for Windows, GraphPad Software (San Diego, CA).
Results
GCMS analysis showed a mixture of monoterpenes among which limonene (52.77%), geranyl acetate (9.92%), and trans-limonene oxide (7.13%) were the main compounds in C. limon EO ().
Table 1. Chemical composition and retention indices of the constituents of the Citrus limon essential oil.
Effects of C. limon EO on lipid peroxidation levels, nitrite concentrations, and GSH concentration in mice hippocampus are presented in . Lipid peroxidation was markedly decreased 12, 13, and 27% in EO 150 [P < 0.001], EO 100 [P < 0.001], and EO 50 [P < 0.001] groups, when compared with corresponding values for the control group, respectively (). No group that received injections of EO (0.1 g/kg) [P > 0.05] or (0.15 g/kg) [P > 0.05] showed alterations in lipid peroxidation level, when compared with the EO 50 group. However, the group treated with EO (0.15 mg/kg) showed decreases of 17 and 15% in lipid peroxidation level, when compared with the EO 50 [P < 0.001] and EO 100 [P < 0.001] groups, respectively.
Figure 1. Citrus limon essential oil effects on status of lipid peroxidation, nitrite content, and glutathione reduced (GSH) levels in mice hippocampus. Results are mean ± SEM for the number of animals used in experimental procedures. The differences in experimental groups were determined by analysis of variance (ANOVA). aP < 0.05 as compared with control animals (ANOVA and Student-Newman-Keuls t-test); bP < 0.05 as compared with EO 50 group (ANOVA and Student-Newman-Keuls t-test); and cP < 0.05 as compared with EO 100 group (ANOVA and Student-Newman-Keuls t-test).
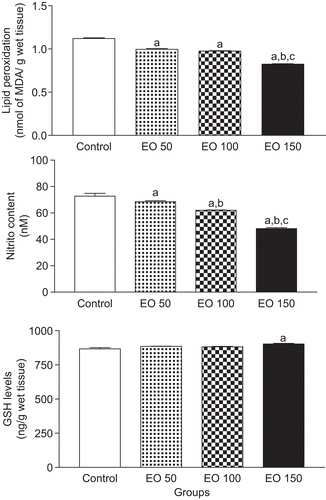
Post hoc comparison of means indicated significant decreases of 34, 30, and 23% in nitrite content of mice hippocampus pretreated with EO 150 [P < 0.001], when compared with the control, EO 50, and EO 100 groups, respectively (). In groups treated with EO 100 [P < 0.001] and EO 50 [P < 0.05], important decreases of 15 and 6% in nitrite content of mice hippocampus were observed, when compared with the control group, respectively. In addition, in EO 100 group [P < 0.01] it was observed a significant decrease of 10% in nitrite content of mice hippocampus, when compared with the EO 50 group.
GSH concentration was not markedly changed in EO 100 and EO 50 groups, when compared with corresponding values for the control group [P > 0.05] (). However, the group treated with EO (0.15 g/kg) showed an increase of 4% in GSH concentration in mice hippocampus, when compared with the control group [P < 0.01].
shows the effects of C. limon EO on SOD, catalase, and GPx activities in mice hippocampus. SOD activities in mice hippocampus was not markedly altered in EO 50 group [P > 0.05], when compared with the control group (). Post hoc comparison of means indicated significant increases of 40, 46, and 28% in SOD activities [P < 0.0001] of hippocampus of mice pretreated with EO 150 as compared with the control, EO 50, and EO 100 groups, respectively (). In addition, post hoc comparison of means indicated significant (9 and 14%) increases in hippocampal SOD activity of mice pretreated with EO 100 [P < 0.0001] when compared with control and EO 50 groups, respectively.
Figure 2. Citrus limon essential oil effects on superoxide dismutase, catalase, and glutathione peroxidase activities in mice hippocampus. Results are mean ± SEM for the number of animals used in experimental procedures. The differences in experimental groups were determined by analysis of variance (ANOVA). aP < 0.05 as compared with control animals (ANOVA and Student-Newman-Keuls t-test); bP < 0.05 as compared with EO 50 group (ANOVA and Student-Newman-Keuls t-test); and cP < 0.05 as compared with EO 100 group (ANOVA and Student-Newman-Keuls t-test).
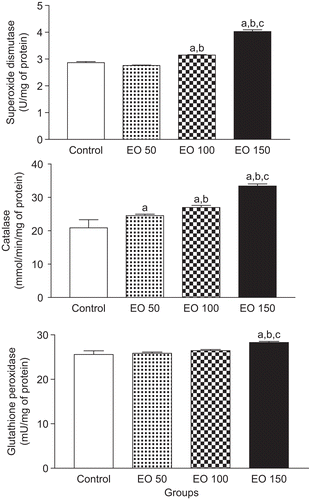
Catalase activities in mice hippocampus were markedly increased (18 and 29%) in EO 50 and EO 100 groups [P < 0.01], when compared with the control group, respectively (). Post hoc comparison of means indicated significant increases of 59%, 36%, and 24% in hippocampus of mice pretreated with EO 150 in catalase activities [P < 0.001], when compared with control, EO 50, and EO 100 groups, respectively ().
GPx activities in mice hippocampus were not markedly changed in EO 50 and EO 100 groups [P > 0.05], when compared with the control group (). Post hoc comparison of means indicated significant increases of 10, 9, and 7% in hippocampus of mice pretreated with EO 150 in GPx activities [P < 0.001], when compared with control, EO 50, and EO 100 groups, respectively ().
Discussion
Most of the studies with EO were carried out in organs and systems other than the CNS (CitationViana et al., 2000; CitationGottlieb et al., 2006). In the present work, lipid peroxidation level, nitrite content, GSH concentration, and antioxidant enzymes were determined in mice hippocampus, after the EO of C. limon administration. We have examined whether the treatment with EO (0.15 g/kg) decreases the lipid peroxidation level in mice hippocampus. Reactive oxygen species (ROS) generation is currently observed in brain (CitationCastagne et al., 1999). In the absence of an efficient defense mechanism, these ROS cause peroxidation of membrane polyunsaturated fatty acids (CitationHalliwell & Gutteridge, 1999). Brain is particularly susceptible to peroxidation due to the simultaneous presence of high levels of polyunsaturated fatty acids and iron (CitationHalliwell & Gutteridge, 1989), which is the target of free radical damage. We have recorded the reduction in lipid peroxidation level in mice hippocampus, after 24 h of EO treatment. This is reflected by decrease in TBARS level that may be related to scavenge of free radicals by EO of C. limon.
It has been demonstrated that neurodegenerative diseases produced changes in nitric oxide metabolism and interacts with glutamatergic receptors to produce part of its stimulatory action on the CNS (CitationDymond & Crandall, 1976; CitationXavier et al., 2007; CitationFreitas, 2009). The fall in nitrite content, after pretreatment with EO of C. limon, is most readily explained as a consequence of inhibiting formation of radicals, scavenges ROS, and lipid peroxidation products (CitationXavier et al., 2007). Increased free radicals and elevated nitrite levels observed in neurodegenerative disease may cause lipid peroxidation (CitationFreitas et al., 2005; CitationFerreira et al., 2009).
SOD, catalase, and GPx activities can protect against neuronal damage involved in physiopathology of neurodegenerative diseases. It has been demonstrated that pretreatment with EO of C. limon (0.15 g/kg) produces an increase in antioxidant enzymatic activities in mouse hippocampus. The increase in antioxidant enzymes activities, after treatment with EO of C. limon, is most readily explained as a necessary consequence of inhibiting ROS formation and can induce the GSH (CitationMichiels et al., 1994; CitationKudin et al., 2004).
Results of animal studies demonstrate that EO of medicinal plants can reduce damage to neurons caused by free radicals that are produced by inflammatory process in neurodegenerative diseases. A growing body of evidence has suggested that ROS generation may underlie the neurotoxic effects of neurodegenerative diseases (CitationBarros et al., 2007; CitationLiang et al., 2007; CitationOliveira et al., 2007; CitationMilitão et al., 2010). Several plant-derived products that exhibit direct antioxidant activity are also being shown to have anticonvulsant activity (CitationMcCord, 1995; CitationHsieh et al., 1999). Recently, our group detected antioxidant properties from lipoic acid, α-tocopherol, and ascorbic acid in pilocarpine-induced seizures in rats (CitationBarros et al., 2007; CitationXavier et al., 2007; CitationSantos et al., 2008; CitationMilitão et al., 2010). Using these data, the present study also investigated whether the EO of C. limon antioxidant actions would be involved in oxidative stress in mice hippocampus. For that, the activities of antioxidant enzymes, as well as the levels of nitrite, GSH, and lipid peroxidation were measured in brain hippocampus of mice.
Neurodegenerative diseases have been found to trigger the activation of membrane phospholipases, proteases, and nucleases, which cause degradation of membrane phospholipids, proteolysis of cytoskeletone proteins, and protein phosphorylation (CitationOno et al., 2000; CitationFreitas et al., 2003; CitationAkbas et al., 2005; CitationObay et al., 2008). In fact, in accordance to those studies, a recent work has shown that neurodegenerative diseases lead to an increase in lipid peroxidation and nitrite levels (CitationFreitas, 2009; CitationSantos et al., 2010). Treatment of animals with (0.05, 0.1, and 0.15 g/kg) of EO of C. limon significantly prevented elevations in lipid peroxidation and nitrite. It is also known that neurodegenerative diseases followed by an increase in lipid peroxidation and nitrite in brain tissue might be diminished by substances with antioxidant properties (CitationWalz et al., 2000; CitationBarros et al., 2007; CitationOliveira et al., 2007). Thus, our results suggest that, at least in part, the EO of C. limon bioprotective activities are also probably related to its antioxidant effects against lipid peroxidation in mice hippocampus.
ROS, such as superoxide, hydroxyl radical, and hydrogen peroxide (H2O2), are normally produced in the brain. The conversion of H2O2 to H2O can be made by catalase and GPx, which involves the GSH, a cofactor of this enzyme. When produced in excess, ROS can also cause enzyme inactivation (CitationSharma et al., 2007). In fact, the current study showed that catalase activity in the EO 150 group was significantly higher than in the control group, demonstrating an alteration in neuroprotective brain defenses. EO treatment with lower doses (0.05 and 0.1 g/kg) preserved catalase activity at normal levels. These findings suggest that EO of C. limon could help the brain cells to counteract the neurodegenerative-induced ROS overproduction and the oxidative damage.
GSH is an essential tripeptide and endogenous antioxidant found in all animal cells. It reacts with the free radicals and can protect the brain from singlet oxygen, hydroxide radicals, and superoxide radical damage (CitationOliveira et al., 2007). Our results showed that the treatment of mice with EO of C. limon resulted in decreased levels of GSH in hippocampus. Interestingly, EO treatment with lower doses (0.05 and 0.1 g/kg) did not produce changes in GSH content, but the highest dose (EO 150) promoted increased GSH levels, suggesting that EO of C. limon could be possibly inducing an increase in GSH levels in mice hippocampus. In a similar way, in previous studies conducted by our group (CitationFreitas et al., 2005; CitationXavier et al., 2007; CitationSantos et al., 2010), we have found that antioxidant compounds themselves were able to induce an increased GSH level in mice hippocampus.
Nevertheless, whether EO of C. limon increases GSH levels, preventing depletion or inducing its synthesis, requires further investigation. Thus, our results are in agreement with previous studies that indicate increased oxidative stress during brain disorders (CitationOliveira et al., 2007; CitationFerreira et al., 2009), but they contrast with assays that show increased oxidative stress and decreased antioxidant defense during anticonvulsant therapy (CitationOno et al., 2000; CitationVinson et al., 2001; CitationDe Sousa et al., 2007).
Conclusions
In a previous study conducted by our group (data not shown), we have reported pharmacological activity from EO of C. limon at doses of 0.05 (32.41 mM/kg), 0.1 (64.82 mM/kg), and 0.15 g/kg (97.23 mM/kg). In the present study, the highest dose of EO of C. limon was needed for effective bioprotective actions against oxidative stress. The acute oral toxicity of EO of C. limon was already evaluated in mice. The results obtained and the LD50 (~1.732 ± 0.1 g/kg) value represent a relative low toxicity from EO of C. limon, which was particularly related to their possible CNS depressor properties. In spite of the knowledge that EO constitution of monoterpenes are often considered to have low toxicity (CitationTanaka et al., 1996; CitationDe Sousa et al., 2007), we assume that additional studies are recommended to further ascertain the safety of EO of C. limon use.
Acknowledgement
We would like to thank the National Council of Technological and Scientific Development (CNPq/Brazil) [grant number 503354/2009-0] and the Research Supporting Foundation of State of Piaui (FAPEPI/Brazil) for the financial support.
Declaration of interest
The authors report no declarations of interest.
References
- Akbas SH, Yegin A, Ozben T. (2005). Effect of pentylenetetrazol-induced epileptic seizure on the antioxidant enzyme activities, glutathione and lipid peroxidation levels in rat erythrocytes and liver tissues. Clin Biochem 38:1009–1014.
- Barros DO, Xavier SM, Barbosa CO, Silva RF, Freitas RL, Maia FD, Oliveira AA, Freitas RM, Takahashi RN. (2007). Effects of the vitamin E in catalase activities in hippocampus after status epilepticus induced by pilocarpine in Wistar rats. Neurosci Lett 416:227–230.
- Benavente-Garcia O, Castillo J, Marin FR, Ortuno A, Del Rio JA. (1997). Uses and properties of citrus flavonoids. J Agric Food Chem 45:4505–4515.
- Castagne V, Gautschi M, Lefevre K, Posada A, Clarke PG. (1999). Relationships between neuronal death and the cellular redox status. Focus on the developing nervous system. Prog Neurobiol 59:397–423.
- Chance B, Maehly AC. (1955). Assay of catalases and peroxidases. Methods Enzymol 2:764–775.
- De Sousa DP, Quintans JL, Almeida RN. (2007). Evaluation of the anticonvulsant activity of α-terpineol. Pharm Biol 45:69–70.
- Del Río JA, Arcas MC, Benavente-García O, Ortuño A. (1998). Citrus polymethoxylated flavones can confer resistance against Phytophthora citrophthora, Penicillium digitatum, and Geotrichum species. J Agric Food Chem 46:4423–4428.
- Draper HH, Hadley M. (1990). Malondialdehyde determination as index of lipid peroxidation. Meth Enzymol 186:421–431.
- Dymond AM, Crandall PH. (1976). Oxygen availability and blood flow in the temporal lobes during spontaneous epileptic seizures in man. Brain Res 102:191–196.
- Elangovan V, Sekar N, Govindasamy S. (1994). Chemopreventive potential of dietary bioflavonoids against 20-methylcholanthrene-induced tumorigenesis. Cancer Lett 87:107–113.
- Ferreira PM, Militão GC, Freitas RM. (2009). Lipoic acid effects on lipid peroxidation level, superoxide dismutase activity and monoamines concentration in rat hippocampus. Neurosci Lett 464:131–134.
- Flohé L, Otting F. (1984). Superoxide dismutase assays. Meth Enzymol 105:93–104.
- Freitas RM. (2009). The evaluation of effects of lipoic acid on the lipid peroxidation, nitrite formation and antioxidant enzymes in the hippocampus of rats after pilocarpine-induced seizures. Neurosci Lett 455:140–144.
- Freitas RM, Bezerra Felipe CF, Nascimento VS, Oliveira AA, Viana GS, Fonteles MM. (2003). Pilocarpine-induced seizures in adult rats: monoamine content and muscarinic and dopaminergic receptor changes in the striatum. Comp Biochem Physiol C Toxicol Pharmacol 136:103–108.
- Freitas RM, Vasconcelos SM, Souza FC, Viana GS, Fonteles MM. (2005). Oxidative stress in the hippocampus after pilocarpine-induced status epilepticus in Wistar rats. FEBS J 272:1307–1312.
- Gottlieb M, Leal-Campanario R, Campos-Esparza MR, Sánchez-Gómez MV, Alberdi E, Arranz A, Delgado-García JM, Gruart A, Matute C. (2006). Neuroprotection by two polyphenols following excitotoxicity and experimental ischemia. Neurobiol Dis 23:374–386.
- Green LC, Tannenbaum SR, Goldman P. (1981). Nitrate synthesis in the germfree and conventional rat. Science 212:56–58.
- Halliwell B, Gutteridge JMC. (1999). Free Radical in Biology and Medicine, 3rd edition. London: Oxford Science Publications, pp. 935–938.
- Halliwell B, Gutteridge JMC. (1989). Lipid peroxidation: A radical chain reaction. Free Radical in Biology and Medicine, 2nd edition. Oxford: Clarendon Press, pp. 188–276.
- Hsieh CL, Tang NY, Chiang SY, Hsieh CT, Lin JG. (1999). Anticonvulsive and free radical scavenging actions of two herbs, Uncaria rhynchophylla (MIQ) Jack and Gastrodia elata Bl., in kainic acid-treated rats. Life Sci 65:2071–2082.
- Kudin AP, Bimpong-Buta NY, Vielhaber S, Elger CE, Kunz WS. (2004). Characterization of superoxide-producing sites in isolated brain mitochondria. J Biol Chem 279:4127–4135.
- Liang LP, Beaudoin ME, Fritz MJ, Fulton R, Patel M. (2007). Kainate-induced seizures, oxidative stress and neuronal loss in aging rats. Neuroscience 147:1114–1118.
- Lowry OH, Rosebrough NJ, Farr AL, Randall RJ. (1951). Protein measurement with the Folin phenol reagent. J Biol Chem 193:265–275.
- Maehly AC, Chance B. (1954). The assay of catalases and peroxidases. Methods Biochem Anal 1:357–424.
- Matos FJA, Machado MIL, Craveiro AA, Alencar JW. (1996). Essential oil composition of two chemotypes of Lippia alba grown in northeast Brazil. J Essent Oil Res 8:695–698.
- McCord JM. (1995). Superoxide radical: controversies, contradictions, and paradoxes. Proc Soc Exp Biol Med 209:112–117.
- Michiels C, Raes M, Toussaint O, Remacle J. (1994). Importance of Se-glutathione peroxidase, catalase, and Cu/Zn-SOD for cell survival against oxidative stress. Free Radic Biol Med 17:235–248.
- Militão GC, Ferreira PM, de Freitas RM. (2010). Effects of lipoic acid on oxidative stress in rat striatum after pilocarpine-induced seizures. Neurochem Int 56:16–20.
- Miyake Y, Yamamoto K, Morimitsu Y, Osawa T. (1998). Characteristics of antioxidative flavonoid glycosides in lemon fruit. Food Sci Technol Int 4:48–53.
- Miyake Y, Yamamoto K, Morimitsu Y, Osawa T. (1997). Isolation of C-glucosylflavone from lemon peel and antioxidative activity of flavonoid compounds in lemon fruit. J Agric Food Chem 45:4619–4623.
- Monforte MT, Trovato A, Kirjavainen S, Forestieri AM, Galati EM, Lo Curto RB. (1995). Biological effects of hesperidin, a citrus flavonoid (note II): Hypolipidemic activity on experimental hypercholesterolemia in rat. Farmaco 50:595–599.
- Obay BD, Tasdemir E, Tümer C, Bilgin HM, Atmaca M. (2008). Dose dependent effects of ghrelin on pentylenetetrazole-induced oxidative stress in a rat seizure model. Peptides 29:448–455.
- Oliveira AA, Almeida JP, Freitas RM, Nascimento VS, Aguiar LM, Júnior HV, Fonseca FN, Viana GS, Sousa FC, Fonteles MM. (2007). Effects of levetiracetam in lipid peroxidation level, nitrite–nitrate formation and antioxidant enzymatic activity in mice brain after pilocarpine-induced seizures. Cell Mol Neurobiol 27:395–406.
- Ono H, Sakamoto A, Sakura N. (2000). Plasma total glutathione concentrations in epileptic patients taking anticonvulsants. Clin Chim Acta 298:135–143.
- Ortuño A, Reynaldo I, Fuster MD, Botía J, Puig DG, Sabater F, Lidón AG, Porras I, Del Río JA. (1997). Citrus cultivars with high flavonoid contents in the fruits. Sci Hortic 68:231–236.
- Rice-Evans C, Miller N, Paganga G. (1997). Antioxidant properties of phenolic compounds. Trends Plant Sci 2:152–159.
- Santos IM, Tomé Ada R, Feitosa CM, de Souza GF, Feng D, de Freitas RM, Jordán J. (2010). Lipoic acid blocks seizures induced by pilocarpine via increases in delta-aminolevulinic dehydratase and Na+, K+-ATPase activity in rat brain. Pharmacol Biochem Behav 95:88–91.
- Santos LF, Freitas RL, Xavier SM, Saldanha GB, Freitas RM. (2008). Neuroprotective actions of vitamin C related to decreased lipid peroxidation and increased catalase activity in adult rats after pilocarpine-induced seizures. Pharmacol Biochem Behav 89:1–5.
- Sedlak J, Lindsay RH. (1968). Estimation of total, protein-bound, and nonprotein sulfhydryl groups in tissue with Ellman’s reagent. Anal Biochem 25:192–205.
- Sharma AB, Sun J, Howard LL, Williams AG Jr, Mallet RT. (2007). Oxidative stress reversibly inactivates myocardial enzymes during cardiac arrest. Am J Physiol Heart Circ Physiol 292:H198–H206.
- Sinet PM, Michelson AM, Bazin A, Lejeune J, Jerome H. (1975). Increase in glutathione peroxidase activity in erythrocytes from trisomy 21 subjects. Biochem Biophys Res Commun 67:910–915.
- Tanaka T, Makita H, Kawabata K, Mori H. (1996). Chemoprotection of 4-NOO-induced oral carcinogenesis by dietary flavonoids diosmin and hesperidin. Rec Adv Gastroenterol Carcinogen 1:1167–1170.
- Viana GS, do Vale TG, Silva CM, Matos FJ. (2000). Anticonvulsant activity of essential oils and active principles from chemotypes of Lippia alba (Mill.) N.E. Brown. Biol Pharm Bull 23:1314–1317.
- Vinson JA, Proch J, Bose P. (2001). Determination of quantity and quality of polyphenol antioxidants in foods and beverages. Meth Enzymol 335:103–114.
- Walz R, Dal-Pizzol F, Klamt F, Vianna MMR, Schröder N, Quevedo J, Benfato MS, Moreira JCF. (2000). Lipid peroxidation in hippocampus early and late after status epilepticus induced by pilocarpine or kainic acid in Wistar rats. Neurosci Lett 291:179–182.
- Xavier SM, Barbosa CO, Barros DO, Silva RF, Oliveira AA, Freitas RM. (2007). Vitamin C antioxidant effects in hippocampus of adult Wistar rats after seizures and status epilepticus induced by pilocarpine. Neurosci Lett 420:76–79.