Abstract
Context: The main objective of the study was to investigate the biochemical mechanism of the antidiabetic activities of the dry extracts of Teucrium polium L. ssp. capitatum (L.) Arcangeli (Lamiaceae), from Republic of Macedonia, traditionally used to treat diabetes.
Materials and methods: Aerial parts of the plant were extracted in alcohol and freeze- or spray-dried, analyzed by high performance liquid chromatography (HPLC) and examined for insulinotropic effect in INS-1E cells in vitro. Their effect on blood glucose, lipids and carbohydrate-related enzymes was tested in normo- and streptozotocin hyperglycemic rats.
Results and discussion: HPLC analyses revealed several flavonoids: luteolin, apigenin, cirsiliol, diosmetin, cirsimaritin and cirsilineol as both free aglycons and glycosides. The extract and mixture of commercial flavonoids showed a distinct insulinotropic effect on INS-1E cells at 500 µg/ml. Intragastric (i.g.) administration of identical doses of the extract (125 mg/kg) in both normo- and hyperglycemic rats was more efficient in lowering the blood glucose than intraperitoneal injection (35% vs. 24% reduction) with highest effect (50% reduction) 8 h after administration. After 10 days of treatment, the magnitude of the effect was comparable to i.g. administration of 2.5 mg/kg of glibenclamide (38% reduction). No effect was seen on blood lipid profiles. In OGTT (oral glucose tolerance test), the extract lowered blood glucose levels by ~35%. The treatment reduced hepatic glycogen and tended to normalize the activity of gluconeogenic enzymes.
Conclusion: The results demonstrate that examined plant extracts contain flavonoids with insulinotropic and antihyperglycemic effects.
Introduction
The genus Teucrium L. (Lamiaceae) comprises more than 300 species, 49 of which grow in Europe. Teucrium species have been used as medicinal herbs for more than 2000 years for their diuretic, diaphoretic, antidiabetic, tonic, antipyretic, anti-inflammatory, anticonvulsive, antihypertensive and cholagogic properties (CitationAtta and Zaman, 1989; CitationBarrachina et al., 1995; CitationBello et al., 1995).
In the Macedonian folk medicine, three species of this genus are very commonly used (T. chamaedrys L., T. montanum L. and T. polium L.). Infusions of a dry flowering plant of Teucrium polium L. ssp. capitatum (L.) Arcangeli (Lamiaceae) is used for treatment of inflammatory affections (skin erythema, hemorrhoids), kidney disorders and as an antidiabetic and antilipidemic drug. Teucrium polium has been studied for its anti-inflammatory and spasmolytic (CitationCapasso et al., 1983; CitationSuleiman et al., 1988; CitationTariq et al., 1989), antinociceptive (CitationAbdollahi et al., 2003) and antipyretic and antibacterial activities (CitationAutore et al., 1984), for its anorexic effect (CitationGharaibeh et al., 1988), but mostly for its antilipidemic and antidiabetic properties (CitationGharaibeh et al., 1988; CitationRasekh et al., 2001; CitationVessal et al., 2003).
Intensive phytochemical work has been carried out on T. polium (Golden germander), and previously, diterpenoids, flavonoids, iridoids, sterols and terpenoids have been identified (CitationRizk et al., 1986; CitationVerykokidou-Vitsaropoulou & Vajias, 1986; CitationKamel & Sandra, 1994; CitationBarrachina et al., 1995; CitationBedir et al., 2010; CitationKawashty et al., 2010). Flavonoids have shown a wide range of pharmacological activities and have been reported to have antidiabetic properties in alloxan and streptozotocin (STZ)-induced diabetes mellitus in rats and rabbits (CitationHii & Howell, 1985; CitationNuraliev & Avezov, 1992; CitationAhmad et al., 2000; CitationVessal et al., 2003).
Diabetes mellitus is a metabolic disorder of multiple etiologies characterized by chronic hyperglycemia with disturbances of carbohydrate, fat and protein metabolism resulting from defects in insulin secretion, insulin action or both. Globally, the estimated incidence of diabetes and projection for year 2030, as given by the International Diabetes Federation, is 366 million (CitationWild et al., 2004).
To gain insight into the biochemical mechanism of action of the antidiabetogenic activities of Teucrium polium ssp. capitatum extracts, we determined its flavonoid compounds and evaluated its effect on blood glucose and blood lipid concentrations in normal and STZ diabetic rats and on insulin secretion in vitro in INS-1E cells.
Methods
Preparation of the extract
Aerial parts of wild, flowering plants were collected in July, 2008, at Belasica Mountain in the region of Strumica, Republic of Macedonia. The material was air-dried, packed in paper bags and kept in a dark and cool place until analysis. Plant identity was verified as Teucrium polium L. ssp. capitatum (L.) Arcangeli (Lamiaceae) by Liuba Evstatieva, and voucher specimens (no. 7/05) were deposited at the Herbarium at the Department of Pharmaceutical Botany, Institute of Pharmacognosy, Faculty of Pharmacy, Skopje, Macedonia.
Milled plant material was extracted with 100 ml 70% ethyl alcohol per gram of plant material by maceration and continuously stirring for 24 h. The extract was then filtered and the alcohol evaporated under low pressure. The aqueous residue was frozen at −80°C; extract T1 was spray-dried and extract T2 was freeze-dried using a Mini Spray Dryer B-290 (Büchi Labortechnik AG, Flawil, Switzerland) or a Christ Alpha 1–4 freeze-dryer (Bioblock Scientific, Illkirch, France), respectively.
Reagents and authentic samples
High-performance liquid chromatography (HPLC)-grade acetonitrile and phosphoric acid were from Merck (Darmstadt, Germany). Reference samples of luteolin, apigenin, diosmetin, cirsimaritin, cirsilineol, flavon, flavanon, apigenin-7-glycoside, luteolin-7-glycoside, rutin, kaempferol and quercetin were from Extrasinthese (Genay, France) and Merck.
HPLC analysis
A Varian HPLC system equipped with a ternary pump, model 9012, an ultraviolet (UV)-diode array detector, model 9065, a reverse phase C18 column 250 × 4 mm, 5 µm RP-18 LiChroCART column (Merck, Darmstadt, Germany), protected with a LiChroCART guard column (4 × 4 mm, RP-18, 5 µm particle size, Merck Darmstadt, Germany) and a 20 µl loop was used. The mobile phase (1.0 ml/min, 35°C) was H3PO4, pH 3.0 (A) and CH3CN (B) and the elution profile 0–5 min 30% B, 10–20 min 35% B, 25–30 min 45% B and 40–48 min 65% B. Dry extracts were redissolved in methanol (1 mg/ml) and filtered through 0.45 µm pore-size polyethersulfone filter (Millipore, Millex-HV 13 mm, Molsheim, France) before analysis. Spectral data from all peaks were accumulated in range 240–400 nm and displayed chromatograms were recorded at 348 nm. Identification of the flavonoids was done by comparing retention time and UV spectra of the components in the extracts with the reference flavonoid compounds. Results comply to the validation requirements of the International Conference for Harmonization guidelines (ICH Q2(R1), 2005).
Cell culture and insulin stimulation setup
INS-1E cells were cultured in 95% RPMI 1640 (Lonza), 5% heat-inactivated fetal calf serum (FCS, Invitrogen, Carlsbad, CA ), 1 mM sodium pyruvate, 50 µM 2-mercaptoethanol, 2 mM l-glutamine and 10 mM HEPES (4-(2-hydroxyethyl)-1-piperazineethanesulfonic acid) in saturated 5% CO2. Cultures were passaged weekly by trypsinization and were free of mycoplasma (Mycoalert, Lonza).
For experiments, INS-1E cells were seeded at 4 × 105 cells/ml in Falcon 12-well tissue culture plates. Following 24 h of culture, the medium was changed to RPMI 1640 ± 0.5% FCS for 24 h, then for 2 h in RPMI 1640, 3 mM glucose, 0.5% FCS. Stimulation was carried out in triplicates by incubation for 30 min in Ca5 buffer [125 mM NaCl, 5.9 mM KCl, 1.28 mM CaCl2, 1.2 mM MgCl2, 25 mM HEPES, 0.1% bovine serum albumin (BSA)] containing 3 mM glucose followed by incubation for 30 min in Ca5 buffer with 20 mM glucose containing extract T1 (5, 50, 250, 500 and 1000 µg/ml) or flavonoids (500 µg/ml flavon, flavanon, apigenin-7-glycoside, luteolin-7-glycoside, rutin, kaempferol or quercetin). Insulin secretion was measured by ELISA (Mercodia, Sweden).
Animals
Male Wistar rats (460–500 g) were housed in 20 ± 2°C, 70% relative humidity and 12 h dark/light cycle. They had free access to standard diet (20% protein, 30% carbohydrate, 9% lipid, 2.5% cellulose, 10% water) and water.
Diabetes was induced by a single intraperitoneal (i.p.) injection of 35 mg/kg body weight STZ (Sigma) freshly dissolved in 0.1 M cold sodium citrate buffer (pH 4.5). After 6 days, rats with fasting blood glucose levels above 15 mmol/l (measured in tail tip blood) were considered diabetic.
For evaluation of hypoglycemic and hypolipidemic effects, normal rats, fasted for 12 h and randomly divided into five groups (n = 6 per group), were used. The rats received either 5 ml of water, extracts T1 or T2 intragastrically (i.g.) via a Sondi needle (i.g.), 0.5 ml (125 mg/kg/day) T2 i.p. or glibenclamide (GLB, 2.5 mg/kg, i.g.) as a positive control. Blood was drawn before and 4, 8 and 12 h after administration of the compounds and either analyzed immediately using an Exactech blood glucose monitor or centrifuged at 4000 rpm for 10 min at 4°C to obtain plasma, which was frozen until analysis.
For the oral glucose tolerance tests, normal rats were fasted for 16 h. Thirty minutes prior to administration of the glucose load (2.5 g/kg), the five groups of rats received the same treatments as above. Blood glucose was measured before the glucose administration and 2 h after the load.
For evaluation of the antihyperglycemic and antihyperlipidemic effects, STZ hyperglycemic rats, previously fasted for 12 h and randomly divided into four groups (n = 6 per group), were used. All rats received a single daily dose (5 ml) of extract T1, T2 (125 mg/kg) or GLB (2.5 mg/kg) i.g., over a period of 10 days. Blood was drawn every day, 8 h after administration. The research was approved by the Ethical Committee of the Faculty of Pharmacy, Skopje, R. Macedonia, and was conducted in accordance with the internationally accepted principles for laboratory animal use and care as found in the European Community guidelines (EEC Directive of 1986; 86/609/EEC).
Enzyme activities and substrate concentration
Liver glycogen content was determined by the anthrone method (CitationSeifter & Dayton, 1950). For enzyme activity measurements, liver tissue was homogenized with an ultrasonic homogenizer. Glucose 6-phosphatase (E.C. 3.1.3.9) was assayed in a substrate mixture that contained G-6-P (100 mM) and ethylene diamine tetra-acetic acid (EDTA, 2 mM), pH 6.5 (CitationHers, 1959). Substrate mixture for fructose 1,6-bisphosphatase (E.C. 3.1.3.11) was 5 mM fructose 1,6-bisphosphate, 2.5 mM MgSO4, 5 mM MnSO4, 30 mM cysteine and 20 mM serine (CitationFreedland & Harper, 1959). The activity of liver glycogen phosphorylase α (E.C. 2.4.1.1) was determined by the method of CitationStalmans and Hers (1975). The substrate mixture contained 50 mM glucose-1-phosphate, 1% glycogen, 2.5 mM EDTA, 0.15 M NaF and 0.5 mM caffeine (pH = 6.0). The enzyme activity was expressed as nmol inorganic phosphate/min/mg protein. The assays were measured spectrophotometrically and the amount of released inorganic phosphate was determined by CitationFiske and Subbarow (1925). The protein content in the tissue extracts was measured using BSA as a standard (CitationLowry et al., 1951). Finally, the concentration of glucose-6-phosphate and fructose-6-phosphate was determined in 1 M perchlorate homogenates, neutralized with 5 M K2CO3, by measuring the production of reduced nicotinamide adenosine dinucleotide phosphate at 340 nm (CitationLang and Michal, 1974).
Statistics
Results are presented as means ± SD. Statistical difference between the groups was evaluated by one way analysis of variance (ANOVA) analysis and Student’s t-test. Differences between time course experiments were tested using repeated measures ANOVA. A probability level of p < 0.050 was used as a significant difference and illustrated by the asterisk.
Results
HPLC analysis
The flavonoids luteolin, apigenin, cirsimaritin, cirsilineol and diosmetin were identified by HPLC analysis () by comparing retention time and UV spectra of the components in the extracts with the reference flavonoid compounds (). Cirsiliol was identified by isolation and identification by mass spectrometry and NMR (CitationStefova et al., 2007). There are no differences noticed in the flavonoid profile between T1 and T2 extracts. In addition, LC-DAD-ESI-MS analyses confirmed these findings (data not shown) and are in agreement with a previous report about flavonoid profile of T. polium (CitationHarborne et al., 1986).
Figure 1. HPLC chromatograms of Teucrium polium ssp. capitatum extract (chromatogram A) compared with chromatogram of flavonoids in the standard mixture (chromatogram B): 1.Eriodictiole; 2. Luteoline; 3. Naringenine; 4. Apigenine; 5. Chriseriole; 6. Diosmetine; 7. Acacetine; 8. Genquanine and in the standard mixture (chromatogram C): 9. Cirsimaritine; 10. Cirsilineol; 11. 5,4’-OH 6,7,8,3’-OCH3-flavone. Cirsiliol (Fx) was isolated and identified by mass spectrometry and NMR (CitationStefova et al., 2007).
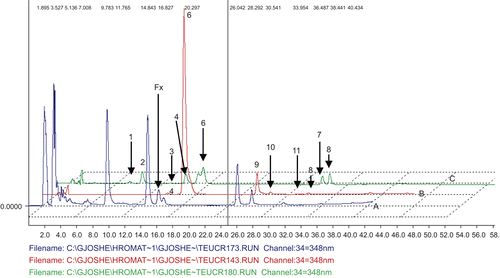
Table 1. Retention time (Rt) in minutes of the flavonoids in the standard mixtures “1” and “2” and in the extract.
Cell culture
In vitro investigations of the insulinotropic effect of Teucrium polium extract revealed a significant increase in insulin secretion by INS-1E insulinoma cells compared with the secretion in medium containing 20 mmol/l glucose alone. There is subsequent increment of the insulinotropic effect in the presence of 5–500 µg/ml T1 extract, whereas medium containing 1000 µg/ml T1 extract was less efficient in stimulating insulin secretion than 500 µg/ml. Statistical analysis showed that there is significant difference between the highest insulinotropic effect (500 µg/ml T1 extract) and the lesser concentrations of medium containing extract. At the most efficient concentration, the insulin secretion increased by a factor of 2.6 (). Further, all flavonoids increased the insulin secretion, although to a variable degree (). The statistical analysis between group of glycoside flavonoids (apigenin-7-glycoside, luteolin-7-glycoside and rutin) exhibited significantly higher potency than the group of aglycones (flavon, flavanon, kaempferol, quercetin).
Figure 2. Insulin secretion from INS-1E cells stimulated with extract T1. Cells were exposed to the extract for 30 min in the range 5 - 1000 μg/mL and in the presence of 20 mM glucose (G). (n=6 for each stimulatory condition. * denotes P<0.05 compared to 20 mM G).
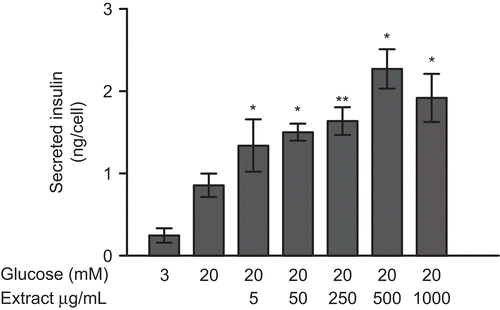
Figure 3. Insulin secretion in INS-1E cells stimulated for 30 min with pure flavonoids (500 μg/mL) in the presence of 20 mM glucose (G). The results have been normalized to secretion per nmole of the flavonoids. The following flavonoids were used: apigenin-7-glycoside, luteolin-7-glycoside, kaempferol, quercetin, rutin, flavon and flavanon. (n=6 for each stimulatory condition. * denotes P<0.05 compared to 20 mM G).
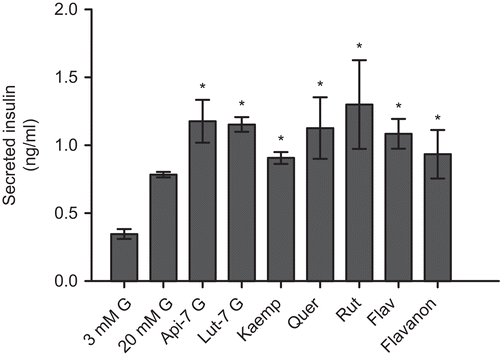
Animal experiments
As shown in , T1 and T2 extracts exhibited an acute, hypoglycemic effect in normal rats after a single dose compared with water (p < 0.001 in all cases). The average blood glucose lowering effect (12 h period), was 24% when injecting T1 i.p. and 35% by i.g. administration. These effects are comparable with the average effect exhibited from i.g. administration of the antidiabetic drug glibenclamide in a single dose of 2.5 mg/kg (38%). The largest hypoglycemic effect (50%) was seen 8 h after a single i.g. administration of 125 mg/kg extract T1, which also compares with glibenclamide (57%). Twelve hours after administration, extract T1 exceeded the efficiency of glibenclamide (38% vs. 30%, p < 0.05).
Figure 4. The effect of a single dose of Teucrium polium ssp. capitatum extract on glucose in normoglycemic rats. Rats were fasted for 12 h and divided into random groups (n=6 per group) and received H2O (•), extract T2 (▪ 125 mg/kg i.g.) or T1 (▴ 125 mg/kg i.g., Δ 125 mg/kg i.p.) or glibenclamide (▪ 2.5 mg/kg GLB i.g.).
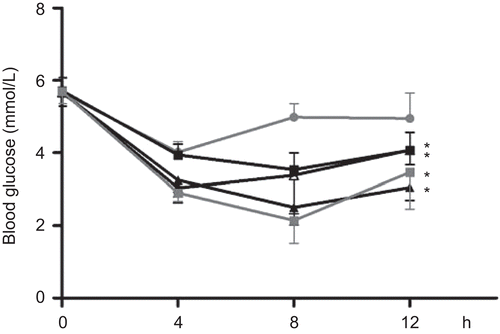
To investigate whether the Teucrium polium ssp. capitatum extract could also reduce postprandial hyperglycemia in normal rats, an oral glucose tolerance test was performed 30 min after administration of a single dose of either extract T1 (125 mg/kg i.p. or i.g.) or T2 (125 mg/kg i.g.). Two hours later (), blood glucose levels were significantly lower in rats receiving extracts T1 i.g. (35%, p < 0.05) but not T2, compared to the animals that received water i.g.
Figure 5. The effect of Teucrium polium ssp. capitatum extract on blood glucose concentration during oral glucose tolerance test. Rats were fasted for 12 h and divided into random groups (n=6 per group) and received H2O (•), extract T2 (▪ i.g.) or T1 (▴i.g., Δ i.p.) extract T1 i.p. 30 min prior to administering a glucose load i.g. Blood glucose concentration was measured before the administration of extracts and two hours after the glucose load. (* denotes P<0.05 compared to the animals that received water).
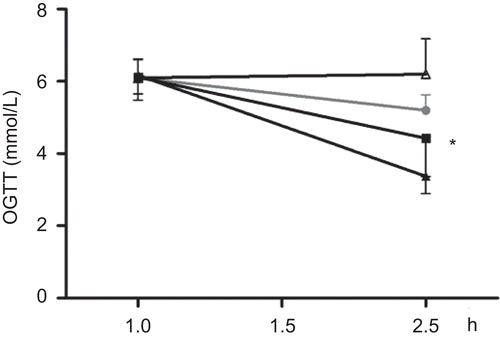
The antihyperglycemic activity of the extract was further investigated by measuring serum glucose concentration in STZ-diabetic rats that had been treated for 10 days with extract (). Significant reductions in blood glucose concentrations were detected after the first administration of either extract at day 1 of the treatment (p = 0.004 and p < 0.001 for T1 and T2, respectively). The largest antihyperglycemic effect, normalizing blood glucose concentrations to ~5 mmol/l, was measured 8 h after administration of extracts, and the effect persisted until next day administration. Again, the effects of the two extracts were comparable (by time and glucose concentrations) with the effect exhibited by glibenclamide (p < 0.001). Serum concentrations of total cholesterol, triglycerides, low-density lipoprotein and high-density lipoprotein were measured along with serum glucose and did not show any significant changes during the experiment (results not shown).
Figure 6. The effect of Teucrium polium ssp. capitatum extract on blood glucose concentration in STZ-diabetic rats after ten days of treatment. Serum glucose concentrations were measured on the last day after administering H2O (•), extract T1 (▴125 mg/kg i.g.), extract T2 (▪ 125 mg/kg i.g.) or glibenclamide (▪ GLB 2.5 mg/kg i.g).
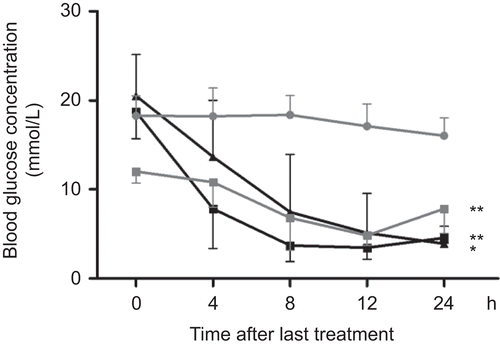
Rat liver analyses
To clarify whether prolonged treatment with Teucrium polium ssp. capitatum extract induced permanent changes in the liver glucose metabolism, we analyzed liver tissue from 10-day-treated animals for metabolites and enzyme activities related to glycogen disposal and retrieval as well as for glycolysis and gluconeogenesis. The analyses () showed that animals with experimental diabetes had significantly reduced hepatic glycogen. Neither Teucrium polium ssp. capitatum extracts nor glibenclamide treatment could recover the diabetes animals from this condition; in fact they all lowered hepatic glycogen further than placebo treatment with water. The same was found for phosphorylase α-activity () and for glucose-6-phosphate () and fructose-6-phosphate content (). Liver glucose 6-phosphatase () and fructose 1,6-bisphosphatase () activities were increased in diabetic animals and reduced, although not normalized, by treatment with both extracts and glibenclamide.
Figure 7. Liver enzyme activities and metabolite concentrations in STZ-diabetic rats treated with Teucrium polium ssp. capitatum extract. The parameters were measured in liver homogenates of the STZ-diabetic rats after 10 days of treatment with either H2O, extract T1 (125 mg/kg i.g.), extract T2 (125 mg/kg i.g.) or glibenclamide (GLB 2,5 mg/kg i.g.) and compared to healthy, untreated animals (C) and diabetic animals, placebo-treated with water (H2O). The panels depict A: Liver glycogen content, B: Glycogen phosphorylase a activity, C: Glucose-6-phosphate concentration, D: Glucose-6-phosphatase activity, E: Fructose-1,6-bisphospshatase activity and F: Fructose-6-phosphate concentration, respectively. It is of note that in all cases placebo treatment with water altered enzyme and metabolite levels (P<0.05). * denotes P<0.05 compared to diabetic animals receiving H2O.
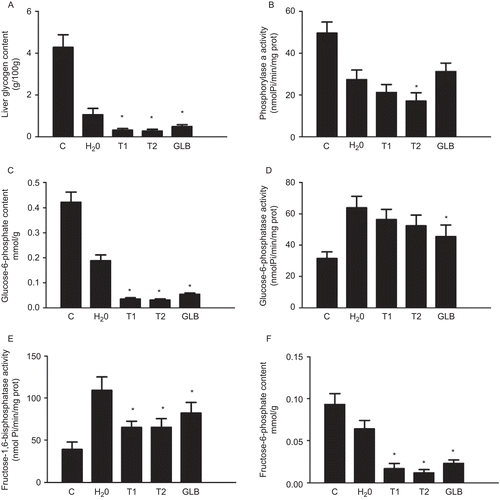
Discussion
The most important result in this study is the observation that extracts of Teucrium polium ssp. capitatum were able to lower the blood glucose concentration in diabetic and normoglycemic animals at a similar magnitude to glibenclamide. Our results suggest that both stimulation of insulin secretion from the pancreatic β-cells and activity changes in carbohydrate-related liver enzymes could contribute to the effect.
Other studies have previously found that Teucrium extracts show hypoglycemic effects in animals (CitationGharaibeh et al., 1988; CitationRasekh et al., 2001; CitationEsmaeili & Yazdanparast, 2004). Most frequently applied doses are 50–150 mg/kg, and in this context, the dose of 125 mg/kg is preferred in this study also for testing different kinds of administration.
An interesting observation in this study was that the effect of i.g. was more pronounced than i.p. administration. This is in agreement with CitationRasekh et al. (2001) who noticed the same phenomenon in normal and STZ hyperglycemic rats. It is assumed that release of intestinal hormones could play a role (incretin effect).
Previous experiments support a role for increased insulin release in the effect of the Teucrium extract in vivo. First, a 160% increase in blood insulin levels is observed in STZ rats, and in vitro rat islets of Langerhans show a 135% increase in insulin secretion (CitationEsmaeili & Yazdanparast, 2004). Also, in a parallel study, insulin release was enhanced by 44–77% in islets exposed to either epicatehin (0.8 mmol/l) or quercetin (0.01–0.1 mmol/l), whereas naringenin (0.1 mmol/l) and chrysin (0.08 mmol/l) inhibited insulin release by 40–60% (CitationHii and Howell, 1985).
We now extend these observations to a β-cell line model, the INS-1E, in which we observed that Teucrium extracts increased the insulin secretion by several hundred percent beyond the maximal glucose stimulus. It is known that some C-glycosyl flavones, isolated from plants, exhibit significant hypoglycemic effects (CitationSezik et al., 2005). Therefore, the presence of the identifiable flavonoids in the extracts and their ability to potentiate insulin secretion during hyperglycemia suggest that these flavonoids are responsible for the insulinotropic effect of the extract. The aglycon luteoline has a potent effect, whereas isoorientin and orientin, C-glycosides of luteoline, do not stimulate the insulin secretion (CitationVessal et al., 2003).
We also examined if the extract could prevent hyperlipidemia, an important risk factor in diabetes patients for developing cardiovascular complications. Several flavonoids were previously examined and confirmed as regulatory agents of hyperlipidemia (CitationChoi et al., 1991) and it has been found that aqueous Teucrium polium extracts reduced total cholesterol and serum triglyceride by 29–46% in an animal model of hyperlipidemia (CitationRasekh et al., 2001). However, we did not observe such effects following Teucrium polium administration in mice.
Since diabetes mellitus impairs the normal capacity of the liver to synthesize glycogen (CitationWhitton & Hems, 1975; CitationVats et al., 2003) and alters enzymes of glucose metabolism markedly, we also measured the activity of key gluconeogenic and glycogenolytic enzymes, some intermediary glucose metabolism substrates and liver glycogen content. As expected (CitationFerrannini et al., 1990), our results showed that hepatic glycogen content was reduced significantly in diabetic animals compared to nondiabetic controls. Neither treatment with extracts nor glibenclamide restored liver glycogen. We also found decreased glycogen phosphorylase α-activity, which is in agreement with a proportional relationship between glycogen content and glycogen phosphorylase activity (CitationSeglen, 1974), and decreased concentrations of glucose-6-phosphate and fructose-6-phosphate in diabetic animals. The latter could contribute to the reduced liver glycogen content, since glucose-6-phosphate is a stimulator of glycogen synthetase (CitationVillar-Palasí and Guinovart, 1997).
Increased hepatic glucose production in diabetes mellitus is associated with impaired suppression of the gluconeogenic enzyme fructose 1,6-bisphosphatase. The activity of enzyme was increased accordingly compared to control animals, as has also previously been described (CitationFerrannini et al., 1990; CitationPari and Satheesh, 2006).
Since a major problem in diabetes is elevated hepatic glucose release, Teucrium treatment could be beneficial in the treatment of the disease, since it reduces hepatic glycogen, glycogenolysis (phosphorylase a) and substrates for glucose release (glucose- and fructose-6-phosphate). Likewise, reductions in glucose 6-phosphatase and fructose 1,6-biphosphatase activity and in gluconeogenesis could improve diabetes regulation.
Conclusions
The study demonstrated that extracts of the aerial parts of Teucrium polium ssp. capitatum is comparable in effect on hyperglycemia in rats to glibenclamide and suggests that the effect is attributable to a combined action of increased insulin release from the pancreas and changes in liver metabolites and enzyme activities. Studies in humans would be needed to clarify the potential benefit of the extract for treating diabetes patients.
Acknowledgements
We thank Dr. P. Maechler, Geneva, Switzerland, for sharing the INS-1E cells with us.
Declaration of interest
The authors report no declarations of interest.
References
- Abdollahi M, Karimpour H, Monsef-Esfehani HR. (2003). Antinociceptive effects of Teucrium polium L total extract and essential oil in mouse writhing test. Pharmacol Res, 48, 31–35.
- Ahmad M, Akhtar MS, Malik T, Gilani AH. (2000). Hypoglycaemic action of the flavonoid fraction of Cuminum nigrum seeds. Phytother Res, 14, 103–106.
- Atta UR, Zaman K. (1989). Medicinal plants with hypoglycemic activity. J Ethnopharmacol, 26, 1–55.
- Autore G, Capasso F, De Fusco R, Fasulo MP, Lembo M, Mascolo N, Menghini A. (1984). Antipyretic and antibacterial actions of Teucrium polium (L.). Pharmacol Res Commun, 16, 21–29.
- Barrachina MD, Bello R, Martinezcuesta MA, Esplugues J, Primo-Yúfera E. (1995). Antiinflammatory activity and effects on isolated smooth muscle of extracts from different Teucrium species. Phytother Res, 9, 368–371.
- Bedir ETD, Calis I, Zerbe O, Sticher O. (2010). Neo-clerodane diterpenoides from Teucrium polium. Phytochemistry, 51, 921–925.
- Bello R, Barrachina D, Martinez-Cuesta MA, Esplugues J, Primo-Yùfera E. (1995). Evaluation of the acute toxicity, analgesic and CNS activities of different species of Teucrium genus. Phytother Res, 9, 277–280.
- Capasso F, Cerri R, Morrica P, Senatore F. (1983). Chemical composition and anti-inflammatory activity of an alcoholic extract of Teucrium polium L. Boll Soc Ital Biol Sper, 59, 1639–1643.
- Choi JS, Yokozawa T, Oura H. (1991). Improvement of hyperglycemia and hyperlipemia in streptozotocin-diabetic rats by a methanolic extract of Prunus davidiana stems and its main component, prunin. Planta Med, 57, 208–211.
- Esmaeili MA, Yazdanparast R. (2004). Hypoglycaemic effect of Teucrium polium: Studies with rat pancreatic islets. J Ethnopharmacol, 95, 27–30.
- Ferrannini E, Lanfranchi A, Rohner-Jeanrenaud F, Manfredini G, Van de Werve G. (1990). Influence of long-term diabetes on liver glycogen metabolism in the rat. Metab Clin Exp, 39, 1082–1088.
- Fiske CA, Subbarow Y. (1925). The colorimetric determination of phosphorus. J Biol Chem, 66, 357–400.
- Freedland RA, Harper AE. (1959). Metabolic adaptations in higher animals. V. The study of metabolic pathways by means of metabolic adaptations. J Biol Chem, 234, 1350–1354.
- Gharaibeh MN, Elayan HH, Salhab AS. (1988). Hypoglycemic effects of Teucrium polium. J Ethnopharmacol, 24, 93–99.
- Grover JK, Vats V, Rathi SS. (2000). Anti-hyperglycemic effect of Eugenia jambolana and Tinospora cordifolia in experimental diabetes and their effects on key metabolic enzymes involved in carbohydrate metabolism. J Ethnopharmacol, 73, 461–470.
- Gupta D, Raju J, Baquer NZ. (1999). Modulation of some gluconeogenic enzyme activities in diabetic rat liver and kidney: Effect of antidiabetic compounds. Indian J Exp Biol, 37, 196–199.
- Harborne JB, Tomas-Barberan FA, Christine AG, Williams M. (1986). A chemotaxonomic study of flavonoids from European Teucrium species. Phytochemistry, 25, 2811–2816.
- Hers HG. (1959). Glycogen storage disease. In: Levine R, Luft R, eds. Advances in Metabolic Disorders. New York, London: Academic press 1964, 36–85.
- Hii CS, Howell SL. (1985). Effects of flavonoids on insulin secretion and 45Ca2+ handling in rat islets of Langerhans. J Endocrinol, 107, 1–8.
- ICH harmonised tripartite guideline Q2(R1). Validation of analytical procedures: Text and methodology; November 2005, Available at: http://www.ich.org/LOB/media/MEDIA417.pdf.
- Kamel A, Sandra P. (1994). Gas chromatography-mass spectrometry analysis of the volatile oils of two Teucrium polium varieties. Biochem Sys Ecol, 22, 529–532.
- Kawashty SA, Gamal El-Din EM, Saleh NAM. (2010). The flavonoid chemosystematics of two Teucrium species from Southern Sinai, Egypt. Biochem Sys Ecol, 27, 657–660.
- Lang G, Michal G. (1974). Methods of Enzymatic Analysis. In: Bermeyer HU, Ed. Verlag Chemie, 1283–1287.
- Lowry OH, Rosebrough NJ, Farr AL, Randall RJ. (1951). Protein measurement with the Folin phenol reagent. J Biol Chem, 193, 265–275.
- Nuraliev IuN, Avezov GA. (1992). The efficacy of quercetin in alloxan diabetes. Eksp Klin Farmakol, 55, 42–44.
- Pari L, Satheesh MA. (2006). Effect of pterostilbene on hepatic key enzymes of glucose metabolism in streptozotocin- and nicotinamide-induced diabetic rats. Life Sci, 79, 641–645.
- Rasekh HR, Khoshnood-Mansourkhani MJ, Kamalinejad M. (2001). Hypolipidemic effects of Teucrium polium in rats. Fitoterapia, 72, 937–939.
- Rizk AM, Hammouda FM, Rimpler H, Kamel A. (1986). Iridoids and flavonoids of Teucrium polium herb. Planta Med, 2, 87–88.
- Seglen PO. (1974). Control of glycogen metabolism in isolated rat liver cells by glucose, insulin and glucagon. Acta Endocrinol Suppl (Copenh), 191, 153–158.
- Seifter S, Dayton S. (1950). The estimation of glycogen with the anthrone reagent. Arch Biochem, 25, 191–200.
- Sezik E, Aslan M, Yesilada E, Ito S. (2005). Hypoglycaemic activity of Gentiana olivieri and isolation of the active constituent through bioassay-directed fractionation techniques. Life Sci, 76, 1223–1238.
- Stalmans W, Hers HG. (1975). The stimulation of liver phosphorylase b by AMP, fluoride and sulfate. A technical note on the specific determination of the a and b forms of liver glycogen phosphorylase. Eur J Biochem, 54, 341–350.
- Stefova M, Stafilov T, Kulevanova S, Stefkov G, Bankova VS. (2007). QSRR of flavones—evaluation of substituent contribution to RP HPLC retention. J Liq Chrom Rel. Technol, 30, 1035–1049.
- Suleiman MS, Abdul-Ghani AS, Al-Khalil S, Amin R. (1988). Effect of Teucrium polium boiled leaf extract on intestinal motility and blood pressure. J Ethnopharmacol, 22, 111–116.
- Tariq M, Ageel AM, al-Yahya MA, Mossa JS, al-Said MS. (1989). Anti-inflammatory activity of Teucrium polium. Int J Tissue React, 11, 185–188.
- Vats V, Yadav SP, Grover JK. (2003). Effect of T. foenumgraecum on glycogen content of tissues and the key enzymes of carbohydrate metabolism. J Ethnopharmacol, 85, 237–242.
- Verykokidou-Vitsaropoulou E, Vajias C. (1986). Methylated flavones from Teucrium polium. Planta Med, 401–402.
- Vessal M, Hemmati M, Vasei M. (2003). Antidiabetic effects of quercetin in streptozocin-induced diabetic rats. Comp Biochem Physiol C Toxicol Pharmacol, 135C, 357–364.
- Villar-Palasí C, Guinovart JJ. (1997). The role of glucose 6-phosphate in the control of glycogen synthase. Faseb J, 11, 544–558.
- Whitton PD, Hems DA. (1975). Glycogen synthesis in the perfused liver of streptozotocin-diabetic rats. Biochem J, 150, 153–165.
- Wild S, Roglic G, Green A, Sicree R, King H. (2004). Global prevalence of diabetes: estimates for the year 2000 and projections for 2030. Diabetes Care, 27, 1047–1053.