Abstract
Context: Daucus carota L. ssp. carota (Apiacea) is widely distributed throughout the world and has many uses in traditional medicine.
Objective: The present study investigates the chemopreventive effects of oil extract of D. carota umbels on 7,12-dimethyl benz(a)anthracene (DMBA)-induced skin cancer in mice.
Materials and methods: D. carota oil extract (DCOE) was prepared by extracting the dried umbels with 50:50 acetone:methanol. Skin papilloma were initiated by DMBA and promoted by 12-O-tetradecanoyl phorobol-13-acetate (TPA). The extract was administered to animals via gavage (0.02 mL of 100% oil), intraperitoneal (0.3 mL of 2% oil), and topical (0.2 mL of 5, 50, and 100% oil) routes for 20 weeks. Tumor appearance, incidence, yield, and volume were compared with those of a non-treated control group.
Results: Topical 100% treatment delayed tumor appearance, and inhibited tumor incidence and yield by 40 and 89%, respectively. Topical 50% treatment inhibited tumor incidence and yield by 30 and 83%, respectively, whereas the 5% treatment inhibited tumor yield by 36%. Tumor volume was decreased by 99, 91, and 70% following topical treatments with 100, 50, and 5% oil, respectively. Intraperitoneal treatment inhibited tumor yield by 43%, and decreased tumor volume by 85%, whereas gavage treatment showed minimal effects on both. Intraperitoneal and topical treatment decreased infiltration and hyperplasia with an increase in the level of hyperkeratosis.
Conclusion: These findings demonstrate that DCOE has remarkable antitumor activity against DMBA-induced skin cancer compared with non-treated animals paving the ground for further investigations.
Introduction
Wild carrot, Daucus carota L. ssp. carota (Apiacae), is a biannual spiny-fruited herb widely distributed throughout the world (CitationReed, 1976; CitationVan Wyk Wink, 2004). The plant is a part of the folk medicine in Lebanon where it is used to protect against gastric ulcer, diabetes, muscle and back pain, and enhance liver function and immune system. There is often confusion in the literature between the edible carrot, D. carota L. ssp. sativus, and the wild carrot, D. carota L. ssp. carota, which has an inedible whitish tough root. Extensive studies have been conducted on D. carota L. ssp. sativus, although little was done on D. carota L. ssp. carota. Wild carrot has been reported to possess antilithic, diuretic, and carminative properties, and has been traditionally used to treat urinary calculus, cystitis, gout, and lithuria (CitationBarnes, 1998; CitationThomas et al., 2001; CitationVan Wyk Wink, 2004). In European folk medicine, the volatile oil from wild carrot is used as a urinary antiseptic anti-inflammatory remedy for cystitis and prostatitis (CitationHoffman, 1991). It has been reported that rats fed on wild carrot exhibited protection against carbon tetrachloride-induced hepatotoxicity (CitationHanda, 1986). Recent work in our lab showed that the aqueous and methanolic extracts of the wild carrot umbels exhibited anti-inflammatory and antiulcer activities (CitationWehbe et al., 2009). Additionally, the aqueous extract was found to possess anticancer activity against human promyelocytic leukemia HL-60 cells (CitationDiab-Assaf et al., 2007).
The present study was conducted to evaluate the antitumor-promoting effects of D. carota oil extract (DCOE) on skin carcinogenesis established through the 7,12-dimethyl benz(a)anthracene (DMBA)-initiation-12-O-tetradecanoyl phorobol-13-acetate (TPA) promotion mouse skin carcinogenesis model (CitationIndra et al., 2007). The antitumor activity was evaluated using three different routes of administration: topical application, gavage, and intraperitoneal injections. Histological analysis of all papilloma biopsies was performed to assess three main skin morphological features: hyperplasia, hyperkeratosis, and dermal infiltration with inflammatory cells.
Materials and methods
Plant collection and oil extraction
D. carota L. ssp. carota were collected in June–July 2008 from Mount Lebanon region. The plant was identified according to the characteristics described in Handbook of Medicinal Herbs book (CitationVan Wyk Wink, 2004) and confirmed by Dr. A. Houri, a Lebanese plant expert at the Lebanese American University. The umbels were air-dried in the shade and then cut into small pieces. Aliquots of 200 g were extracted with 50:50 acetone:methanol (3 × 2 L, 72 h each) (CitationMockute Nivinskiene, 2004). The extract was then filtered and evaporated to dryness under reduced pressure. The residue was centrifuged and the oil was dried over anhydrous sodium sulfate. The final yield (3.47%) was stored in a closed amber bottle at 4°C until use.
GC-MS analysis
The composition of the DCOE was analyzed using GC-MS QP2010 (Shimadzu, Japan) with a fused silica capillary column (30 m × 0.25 mm) with a bonded stationary phase: DB-1. The carrier gas was helium with the split ratio of 1:30, and the flow rate of 1.71 mL/min was applied. The temperature program was 2.5 min at 50°C and then from 50° to 270°C at 10°C/min. The injector temperature was 250°C. Identification of the components was performed by comparing their mass spectra with literature (NIST and WILEY). Percentage composition was computed from GC peak areas.
Animals
BALB/c mice, caged in groups of five and maintained under a 12-h photoperiod (08:00–20:00) at an environmental temperature of 22°C ± 2°C, were used. All experimental protocols were approved by the Animal Ethical Committee of the Lebanese American University, which complies with the Guide for the Care and Use of Laboratory Animals (Committee for the Update of the Guide for the Care and Use of Laboratory Animals, 2010).
LD50 determination
The lethal dose (LD50) was determined using seven different groups (10 mice each). Each group was injected on a daily basis for a period of 2 weeks with one of the following doses 0.5, 1, 1.5, 2, 7.5, 10, and 20% corresponding to 50, 100, 150, 200, 750, 1000, and 2000 mg/kg body weight, respectively. All doses were volumetrically diluted with isotonic saline solution (0.9%) to a total volume of 0.3 mL. Postmortem examination was performed on those animals that died immediately after injections.
Papilloma induction
BALB/c mice papillomas were initiated by a single topical application of 190 nmol of DMBA in 0.2 mL acetone. The dorsal surface was shaved three times until the hair growth was efficiently reduced to assure that the mice were in a resting phase of their hair growth cycle at the time of tumor initiation (CitationKhavari, 2006). Three weeks after the initiation, papillomas were promoted twice weekly at Day 1 and Day 4 with a topical application of 8 nmol TPA in 0.2 mL acetone. TPA solution was delivered to the dorsal skin of individual mice twice per week for the duration of the experiment (20 weeks) (CitationIndra et al., 2007).
Treatment with DCOE
The oil extract was administered to seven different groups (10 mice each) via three different routes: intraperitoneal, gavage, and topical application. For the intraperitoneal treated group, the mice received 0.3 mL of 2% oil diluted with 0.9% NaCl (CitationFarhat et al., 2001). For the gavage treated group, 0.02 mL extract was forced through the mouse pharyngo-esophageal region (Konoshima Takasaki, Citation2002). As for the topical treated group, different oil concentrations were prepared (5, 50, and 100%). The oil was dissolved in acetone and applied topically to the dorsal skin of mice in volume of 0.2 mL (CitationGali-Muhtasib and Affara, 2000). Animals of negative control group received 0.2 mL acetone. TPA solutions were applied topically on the dorsal skin surface 20 min after applying the oil to ensure complete dryness of the skin prior to tumor promotion. Negative and positive control groups of the three different routes of treatment were produced.
Data collection
Tumor incidence (percentage of mice bearing tumors in each group) and tumor yield (number of tumors per mouse) were recorded on a daily basis. Tumor volume was recorded as well at Weeks 15, 17, and 20 (CitationZhaorigetu et al., 2003). Tumor volume was estimated in comparison with specific reference volume models provided by our labs.
Histological analysis
Biopsies of volume greater than 1 mm3 were excised from all the groups and preserved in 10% formaldehyde in phosphate-buffered saline (PBS). Later, all these samples were processed with routine histological procedures using periodic acid Schiff (PAS) stain for later light microscopy examination.
Statistical analysis
Values of the different tested parameters within each group are presented as mean ± SEM. Comparison of each group with the control was made by independent t-test. P-value <0.05 is considered to be significant.
Results
The most prominent components identified by GC-MS analysis of DCOE are shown in . The chromatographic analysis also revealed the presence of a number of unidentified and trace compounds with C15H26O (base peak 119) being a major one constituting 32.36%. LD50 assay showed that the lethal dose for the DCOE is slightly <4%; therefore, a 2% (200 mg/kg body weight) dose was used for the intraperitoneal treatment.
Table 1. Main components (≥1%) of Daucus carota oil.
Data presented in and show the percentage of tumor incidence and tumor yield, respectively, throughout the study period. and reveal the percentage inhibition of tumor yield and incidence at Weeks 15 and 20, respectively. The three topical applications of DCOE (5, 50, and 100%) exhibited dose-dependent chemopreventive effects. The tumor appearance in the 100% topical treated group was delayed by 4 weeks with 40% of the mice showing no sign of tumor at the end of the experiment. The tumor yield was inhibited by 89% and the tumor volume was very minimal to be excised for histological analysis. In case of 50% topical treated groups, the tumor appearance was delayed by 2 weeks, and the tumor incidence, yield, and volume were inhibited by 30, 82, and 92%, respectively. The 5% topical oil treatment showed similar profile but with weaker activity. Gavage treated group had no significant effects on tumor incidence and yield starting Week 6 untill Week 20.
Table 2. The effects of different concentrations of Daucus carota oil when administered by various means on the promotion of mouse skin papilloma (PAPS) at Week 15.
Table 3. The effects of different concentrations of Daucus carota oil when administered by various means on the promotion of mouse skin papilloma (PAPS) at Week 20.
Figure 1. Effect of Daucus carota oil extract administered by various means on (A) papilloma incidence and on (B) papilloma yield.
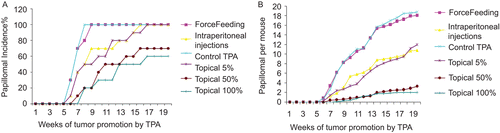
Tumor volume results were recorded on Weeks 15, 17, and 20. shows a significant decrease in the tumor volume in the three topical treated groups reduced with respect to the control, and the exact percent inhibition of tumor volume is clearly highlighted in . The gavage treated groups showed no significant decrease in the tumor volume ( and ), but the effectiveness of the intraperitoneal treated group continued to be significant with a remarkable decrease in the tumor volume after Week 15 ( and ).
Table 4. The inhibitory effects of different concentrations of Daucus carota oil administered by various means on the papilloma volume (mm3) at Weeks 15, 17, and 20.
Figure 2. Effect of Daucus carota oil extract on tumor volume (mm3). (A) Topical application; (B) gavage and intraperitoneal injections. Bars represent mean tumor volume ± SEM. *P < 0.05 significant compared with control.
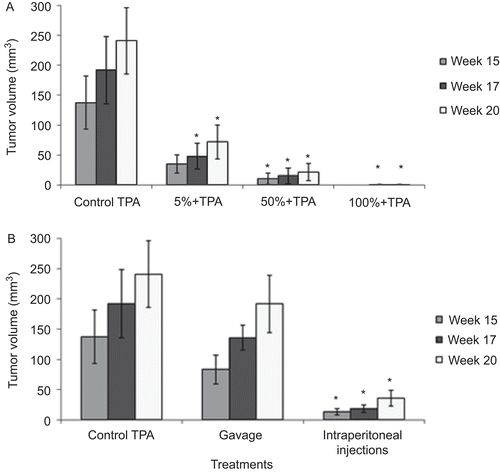
All slides representing the experimental groups were subjected to histological analysis and presented in . The control group showed extreme modifications in the skin morphological features showing a huge increase in the level of hyperkeratosis () and hyperplasia () with high levels of dermal infiltration and collagen core bundles (). Also, around 30% of the biopsies isolated from this group showed total tissue degradation (). For the gavage treated group, the level of hyperplasia and hyperkeratosis ( and ) were similar to those detected in the control ( and ) with a slight decrease in the level of dermal infiltration and collagen core bundles (). Unlike the control group, no tissue degradation was detected in the gavage treated group (). The intraperitoneal treated group showed remarkable decrease in the level of hyperplasia () and dermal infiltration (). Still a high degree of hyperkeratosis is detected (). Tissue necrosis was also obvious in 30% of the tumor biopsies (). In the 5% topical treated group, a decrease in the level of hyperplasia () and dermal infiltration () was detected. Still the level of hyperkeratosis was high similar to that indicated in the intraperitoneal groups (). In the case of 50% topical treated group, the slides indicated skin morphology mostly close to normal with minimal levels of hyperplasia and hyperkeratosis with moderate levels of infiltration ( and ). In addition, some papilloma biopsies (about 30%) showed samples of tissue necrosis (). For the 100% topically treated group, none of the tumor biopsies had a volume greater than 1 mm3 and consequently none could be excised for histological analysis.
Figure 3. Histological analysis of skin tumors induced by 7,12-dimethylbenz(a)anthracene (DMBA)/12-O-tetradecanoyl phorobol-13-acetate (TPA) treatment in mice removed after 20 weeks. Periodic acid Schiff (PAS) staining revealed the differences concerning several morphological features between the control (A–C), gavage treated groups (D–F), intraperitoneal treated groups (G–I), 5% treated groups (J–L), and the 50% topical treated groups (M–O). Black brackets points to the intensity of hyperplasia, black arrows point to hyperkeratosis, and black arrow heads indicate the levels of infiltration and the density of collagen core bundles. 200× magnification.
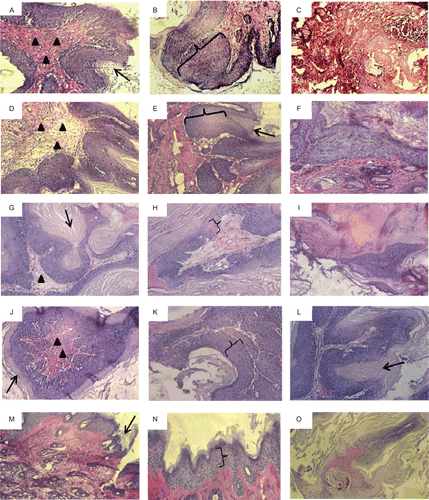
Discussion
Skin carcinogenesis is a multistage process involving initiation, promotion, and progression. Recently, chemoprevention strategy received a great deal of attention in the fight of different types of carcinogenesis. Chemopreventive products may interfere by arresting, reversing, or inhibiting the carcinogenesis process (CitationSporn Suh, 2000). It is now well-accepted that herbal medicine and plant-based diets have great potential in reducing the risk of various types of carcinogenesis. D. carota umbels are among folk medicine used in Lebanon for the treatment of cancer. Therefore, the present study was conducted to evaluate its chemopreventive activity against DMBA–TPA-induced skin carcinogenesis in mice.
The intraperitoneal treatment of DCOE showed antitumor effects as reflected in the histological analysis and the significant inhibition of papilloma yield (43%) and volume (85–90%), thus suggesting its chemopreventive activity. On the contrary, DCOE administration by gavage showed no significant effects on the appearance, incidence, and volume of papillomas. The lack of effect may be attributed to the bioinactivation or poor gastrointestinal absorption of the active oil components during their passage through the gastrointestinal tract. Topical applications of DCOE exhibited dose-dependent chemopreventive effects. Histological analysis revealed that the samples morphology is almost similar to normal skin with preserved sebaceous glands, which indicates the absence of any tissue degradation. Results showed the presence of tissue necrosis, which is a clear indication that this oil concentration did not act as a physical barrier to TPA penetration.
Some of the identified compounds in the extract were reported to possess antitumor activity. Among the sesquiterpenes found in DCOE, β-caryophyllene and α-humulene were reported to exhibit activity against Ehrlich ascites tumor in mice where a 20 mg/kg dose of each increased significantly the survival of the tumor bearing mice (CitationDa Silva et al., 2007). Both compounds were also found to display selective toxicity against HeLa, A-549, and HT-29 tumor cells when compared with nontumor cells (CitationDa Silva et al., 2008). In vitro studies revealed cytotoxic activity of β-caryophyllene against Ehrlich ascites tumor cells (CitationDa Silva et al., 2007) and α-humulene against MCF-7, PC3, A-549, DLD-1, M4BEU, and CT26 cell lines (CitationLegault et al., 2003). Also, a noncytotoxic dose of β-caryophyllene was found to potentiate the anticancer activity of paclitaxel on MCF-7, DLD-1, and L-929 cell lines (CitationLegault et al., 2003). Similarly, β-caryophyllene significantly increased the anticancer activity of α-humulene isocaryophyllene on MCF-7 cell line (CitationLegault Pichette, 2007). According to CitationLima et al. (2003), the copaiba oil resin (COR) from Copaifera multijuga Hayne (Fabaceae), rich in β-caryophyllene (57.46%) and α-humulene (8.28%), produced a significant decrease in tumor growth of subcutaneously injected melanoma cell line (B16F10) in mice in addition to its in vitro cytotoxic activity. Similarly, CitationSylvestre et al. (2006) reported anticancer activity of Croton flavens L. (Euphorbiaceae) essential oil against human lung carcinoma and human colon adenocarcinoma cell lines. The sesquiterpenes β-caryophyllene, caryophyllene oxide, α-humulene, and allo-aromadendrene identified in DCOE were among the compounds present in C. flavens essential oil. Likewise, methyl isoeugenol, a major component of DCOE, was reported to have a modest but specific inhibition of melanoma cell (GR-Mel) growth at 100 µM concentration (CitationPisano et al., 2007). Widdrol, an odorous compound present in DCOE, has been also shown to inhibit the proliferation of human colon adenocarcinoma cells (CitationKwon et al., 2010). Along the same line, extract of myrrh, which contains a diverse range of constituents including β-selinene (CitationHanus et al., 2005), exhibited tumoricidal properties against lung, breast, and prostate cancer cell lines (CitationMazzio Soliman, 2009).
The anticancer activity of DCOE may be attributed to the specific components mentioned above. However, their low concentration (β-caryophyllene 1.26%, caryophyllene oxide 1.3%, and α-humulene 2.63%) may not solely explain such activity, which means some other compounds are active in the DCOE especially that about 43% of the oil component are minor or unidentified compounds. C15H26O a major unidentified compound (32.36%) is currently under investigation for potential anticancer property. It is also possible that synergistic effects may exist between the active compounds and/or the minor/unidentified components.
The antioxidant activity of DCOE was recently evaluated in our laboratory (data not published) by assessing the free radical scavenging activity using DPPH and FRAP assays. Preliminary data revealed significant antioxidant activity of DCOE, a finding that can be partially related to the anticancer activity since the relationship between cytotoxicity and antioxidant activity is well-established (CitationOwen et al., 2000; CitationDwivedi et al., 2003).
Conclusion
DCOE exhibited remarkable antitumor potential against chemically induced carcinogenesis model. Upon the evaluation of each mode of oil treatment, both intraperitoneal and topical treatments appeared to be effective in reducing tumor yield and volume with concomitant improvement in tissue histology. Current investigations in our laboratory are underway to characterize the new active components and unravel their mode of action.
Acknowledgement
We thank Ms. Jessica Saliba and Ms. Simona Ghanem for the histological processing; Mr. Jean Karam for taking care of the animals; and Mrs. Helena Bou Farah Aoukar for lab assistance.
Declaration of interest
The authors report no declarations of interest.
References
- Barnes J. (1998). Herbal medicine. Pharm J, 260, 344–348.
- Committee for the Update of the Guide for the Care and Use of Laboratory Animals, National Research Council. (2010). Guide for the Care and Use of Laboratory Animals, 8th ed. The National Academies Press, Washington, DC. Retrieved from http://www.nap.edu
- Da Silva SL, Da Silva Chaar J, Figueiredo PMS, Yano T. (2008). Cytotoxic evaluation of essential oil from Casearia sylvestris Sw on human cancer cells and erythrocytes. Acta Amaz, 38, 107–112.
- Da Silva SL, Figueiredo PM, Yano T. (2007). Chemotherapeutic potential of the volatile oils from Zanthoxylum rhoifolium Lam leaves. Eur J Pharmacol, 576, 180–188.
- Diab-Assaf M, Mroueh M, El-Sharif S. (2007). Evaluation of anticancer effect of Daucus carota on the human promyelocytic leukemia HL-60 cells. AACR International Conference on Molecular Diagnostics in Cancer Therapeutic Development, Atlanta, USA, p. 61.
- Dwivedi C, Muller LA, Goetz-Parten DE, Kasperson K, Mistry VV. (2003). Chemopreventive effects of dietary mustard oil on colon tumor development. Cancer Lett, 196, 29–34.
- Farhat GN, Affara NI, Gali-Muhtasib HU. (2001). Seasonal changes in the composition of the essential oil extract of East Mediterranean sage (Salvia libanotica) and its toxicity in mice. Toxicon, 39, 1601–1605.
- Gali-Muhtasib HU, Affara NI. (2000). Chemopreventive effects of sage oil on skin papillomas in mice. Phytomedicine, 7, 129–136.
- Handa SS. (1986). Natural products and plants as liver protecting drugs. Fitoterapia, 57, 307–351.
- Hanus LO, Rezanka T, Dembitsky VM, Moussaieff A. (2005). Myrrh–Commiphora chemistry. Biomed Pap Med Fac Univ Palacky Olomouc Czech Repub, 149, 3–27.
- Hoffman D. (1991). The New Holistic Herbal, 3rd edition. Element Shaftesbury Dorset, Great Britain, SP7 8BP.
- Indra AK, Castaneda E, Antal MC, Jiang M, Messaddeq N, Meng X, Loehr CV, Gariglio P, Kato S, Wahli W, Desvergne B, Metzger D, Chambon P. (2007). Malignant transformation of DMBA/TPA-induced papillomas and nevi in the skin of mice selectively lacking retinoid-X-receptor alpha in epidermal keratinocytes. J Invest Dermatol, 127, 1250–1260.
- Khavari PA. (2006). Modelling cancer in human skin tissue. Nat Rev Cancer, 6, 270–280.
- Konoshima T, Takasaki M. 2002. Cancer-chemopreventive effects of natural sweeteners and related compounds. Pure Appl Chem, 74, 1309–1316.
- Kwon HJ, Hong YK, Park C, Choi YH, Yun HJ, Lee EW, Kim BW. (2010). Widdrol induces cell cycle arrest, associated with MCM down-regulation, in human colon adenocarcinoma cells. Cancer Lett, 290, 96–103.
- Legault J, Dahl W, Debiton E, Pichette A, Madelmont JC. (2003). Antitumor activity of balsam fir oil: production of reactive oxygen species induced by alpha-humulene as possible mechanism of action. Planta Med, 69, 402–407.
- Legault J, Pichette A. (2007). Potentiating effect of beta-caryophyllene on anticancer activity of alpha-humulene, isocaryophyllene and paclitaxel. J Pharm Pharmacol, 59, 1643–1647.
- Lima SR, Junior VF, Christo HB, Pinto AC, Fernandes PD. (2003). In vivo and in vitro studies on the anticancer activity of Copaifera multijuga hayne and its fractions. Phytother Res, 17, 1048–1053.
- Mazzio EA, Soliman KF. (2009). In vitro screening for the tumoricidal properties of international medicinal herbs. Phytother Res, 23, 385–398.
- Mockute D, Nivinskiene O. (2004). The sabinene chemotype of essential oil of seeds of Daucus carota L. ssp. carota growing wild in Lithuania. J Essent Oil Res, 16, 277–288.
- Owen RW, Giacosa A, Hull WE, Haubner R, Würtele G, Spiegelhalder B, Bartsch H. (2000). Olive-oil consumption and health: The possible role of antioxidants. Lancet Oncol, 1, 107–112.
- Pisano M, Pagnan G, Loi M, Mura ME, Tilocca MG, Palmieri G, Fabbri D, Dettori MA, Delogu G, Ponzoni M, Rozzo C. (2007). Antiproliferative and pro-apoptotic activity of eugenol-related biphenyls on malignant melanoma cells. Mol Cancer, 6, Article 8.
- Reed CF. (1976). Information summaries on 1000 economic plants. Typescripts submitted to the USDA.
- Sporn MB, Suh N. (2000). Chemoprevention of cancer. Carcinogenesis, 21, 525–530.
- Sylvestre M, Pichette A, Longtin A, Nagau F, Legault J. (2006). Essential oil analysis and anticancer activity of leaf essential oil of Croton flavens L. from Guadeloupe. J Ethnopharmacol, 103, 99–102.
- Thomas KJ, Nicholl JP, Coleman P. (2001). Use and expenditure on complementary medicine in England: A population based survey. Complement Ther Med, 9, 2–11.
- Van Wyk B-E, Wink M. (2004). Medicinal Plants of the World. Briza Publications, Pretoria, South Africa, pp. 124.
- Wehbe K, Mroueh M, Daher C. (2009). The potential role of Daucus carota aqueous and methanolic extracts on inflammation and gastric ulcers in rats. J Comp Integ Med, 6, Article 7.
- Zhaorigetu S, Yanaka N, Sasaki M, Watanabe H, Kato N. (2003). Silk protein, sericin, suppresses DMBA–TPA-induced mouse skin tumorigenesis by reducing oxidative stress, inflammatory responses and endogenous tumor promoter TNF-alpha. Oncol Rep, 10, 537–543.