Abstract
Context: The destabilization of β-amyloid (Aβ) peptide aggregates and the protection of functional cells are the attractive therapeutic strategies for Alzheimer’s disease (AD). Some active ingredients of Salvia miltiorrhiza f. alba C.Y.Wu & H.W.Li (Lamiaceae) (SM) have attracted increasing attention for the treatment of neurodegenerative diseases.
Objective: Salvianic borneol ester (SBE) is a new compound based on SM formulas. The present study was designed to examine the anti-amyloid effects and neuroprotection of SBE in vitro.
Materials and methods: The destabilizing effects of SBE and its related compounds (salvianic acid A and borneol) on preformed Aβ oligomers were measured by using fluorescence spectroscopy with thioflavin T (ThT) and the destabilizing effects of SBE were further confirmed visually by transmission electron microscopy (TEM). The neuroprotective effects of SBE against hydrogen peroxide (H2O2)-induced toxicity in human neuroblastoma cells (SH-SY5Y) and motor neuron hybridoma cells (VSC 4.1) were shown by MTT assay and morphological observation.
Results: SBE showed the most significant destabilizing effect, though the mixture of salvianic acid A and borneol also destabilized Aβ1–40 oligomers. The destabilizing activity of salvianic acid A or borneol alone was not significant. SBE destabilized Aβ1–40 oligomers in dose- and time-dependent manners and the destabilizing effect could also be seen in the photographs of TEM. Furthermore, SBE could protect SH-SY5Y cells and VSC 4.1 cells against H2O2-induced toxicity in a dose-dependent manner.
Discussion and conclusion: SBE had the bifunctional activities of anti-amyloid and neuroprotection. It may have therapeutic potential for AD and be an alternative lead compound for developing new drugs against AD.
Introduction
Alzheimer’s disease (AD) is a neurodegenerative disease characterized by loss of memory and cognition especially in the elderly. Since existing treatments for AD only offer limited symptom alleviation (CitationLleó et al., 2006; CitationJakob-Roetne Jacobsen, 2009), new alternative agents are urgently needed. One of the major pathological features of AD is the appearance of senile plaques composed mainly of β-amyloid (Aβ) peptides (CitationJakob-Roetne Jacobsen, 2009). Monomeric Aβ can aggregate into oligomeric and fibrillar forms and finally become senile plaques in the brain. The Aβ aggregates possess neurotoxicity in vivo and in vitro (CitationYankner et al., 1990; CitationHardy Higgins, 1992). Recent evidence has shown that oligomeric form of Aβ rather than the fibril is primarily responsible for the neuronal injury and death (CitationCleary et al., 2005). Therefore, some therapeutic efforts are targeted at finding anti-amyloid agents to disrupt Aβ aggregation, especially to reduce oligomers in AD (CitationHirohata et al., 2008; CitationShah et al., 2008).
Common Aβ oligomer neurotoxic mechanisms are related with oxidative stress (CitationBehl, 1997). Aβ oligomers generated reactive oxygen species (ROS), which can cause damage to cellular lipids, proteins, or DNA and eventually lead to neuronal death (CitationZhu et al., 2007). As the major component of ROS, hydrogen peroxide (H2O2) is generally used as an inducer of oxidative stress in vitro models by many laboratories (CitationTabner et al., 2002; CitationZhang et al., 2007; CitationBi et al., 2008). Some compounds have been proven to possess neuroprotective potential as antioxidants, such as curcumin, resveratrol, lipoic acid, (−)-epigallocatechin-3-gallate (EGCG), and melatonin (CitationMecocci et al., 2008; CitationZhao, 2009).
Salvia miltiorrhiza f. alba C.Y.Wu & H.W.Li (Lamiaceae) (SM) is a traditional medicinal plant. SM formulas composed mainly of SM, borneol, and Panax notoginseng (Burkill) F.H.Chen ex C.Y.Wu & K.M.Feng (Araliaceae) are commonly used in the clinical treatment of cardiovascular diseases and cerebrovascular diseases (CitationZhou et al., 2005). Recently, SM and its active ingredients have attracted increasing attention for the treatment of neurodegenerative diseases. For AD, some components of SM such as hydrotanshinone and cryptotanshinone have been reported to have potential therapeutic effects as inhibitors of acetylcholinesterase in vitro (CitationRen et al., 2004; CitationLin et al., 2008). In addition, it had been reported that cryptotanshinone attenuated amyloid plaque deposition in the brain of transgenic mouse and decreased Aβ generation in rat cortical neuronal cells overexpressing human APP (CitationYu et al., 2007; CitationMei et al., 2009).
Salvianic borneol ester (SBE, benzenepropanoic acid, α,3,4-trihydroxy-(1R, 2S, 4R)-1,7,7-trimethylbicyclo[2.2.1]hept-2-yl ester, C19H26O5, MW = 334.41) designed based on SM formulas is a new compound synthesized by salvianic acid A and borneol () (CitationZheng et al., 2007; CitationHan et al., 2009). Salvianic acid A and borneol are main components of SM and borneol, respectively. To date, the role of SBE in treatment of AD has not been reported. In this study, the anti-amyloidogenic and antioxidative properties of SBE were reported. We examined the effects of SBE and its related compounds on the destabilization of preformed Aβ oligomers by using fluorescence spectroscopy with thioflavin T (ThT) and the destabilizing effect of SBE was further confirmed by transmission electron microscopy (TEM). Then, we demonstrated the neuroprotective effects of SBE against H2O2-induced toxicity in human neuroblastoma cells (SH-SY5Y) and motor neuron hybridoma cells (VSC 4.1) by a 3-(4,5-dimethylthiazol-2-yl)-25-diphenyl tetrazolium bromide (MTT) assay.
Materials and methods
Reagents
Salvianic acid A and borneol were purchased from the National Institute for the Control of Pharmaceutical and Biological Products (Beijing, China), with Standard Medical Compound Certifications issued by the State Food and Drug Administration (SFDA), China. SBE (>95% purity) was kindly provided by Prof. Xiaohui Zheng. The Aβ1–40 peptide was purchased from Bachem AG (Bubendorf, Switzerland). ThT was obtained from Sigma-Aldrich Chemical Company (St. Louis, MO). All reagents used in this study were of analytical grade.
Preparation and destabilization of Aβ oligomers
Aβ oligomers were prepared according to the method published previously (CitationKlunk et al., 1999), with slight modifications. Aβ1–40 peptide (1 mg) was dissolved in 2 mL 0.01 M phosphate-buffered saline (PBS, pH = 7.4) containing 1 mM EDTA. The solution was incubated at 37°C for 72 h with shaking. The resulting Aβ1–40 oligomers were stored at −80°C and were diluted 5-fold to give a concentration of 0.1 mg/mL prior to assay.
The reaction mixtures containing aliquots of Aβ oligomers with/without a test agent were incubated at 37°C for 30 min with shaking. The agents used in this experiment included SBE, salvianic acid A, borneol at a final concentration of 10 µg/mL, and a mixture of salvianic acid A and borneol at 1:1 (5 µg/mL, respectively). Further, the incubation of Aβ oligomers with SBE was performed at various final concentrations of SBE (0.31–40 µg/mL) and hours (0–6 h) (10 µg/mL of SBE).
ThT fluorescence assay
ThT-induced fluorescence changes were measured to quantify Aβ oligomers by using a RF-5301PC Spectrofluorometer (Shimadzu, Japan) according to the method described by CitationFujiwara et al. (2006). The mixtures containing Aβ oligomers with/without a 10 µg/mL test agent were added to PBS (0.01 M, pH = 7.4) containing 3 µM ThT (Sigma). Each assay was run in triplicate. Excitation and emission wavelengths were set at 445 and 485 nm, respectively. The fluorescence intensity of 3 µM ThT was measured as the background. The fluorescence intensity of 3 µM ThT and Aβ oligomers without test agent was considered to be 100% as control. The amount of Aβ oligomers were calculated as a percentage of control.
Transmission electron microscopy
Aβ1–40 oligomers were incubated with/without SBE at concentrations 2.5, 20, and 40 µg/mL for 30 min at 37°C with shaking. Following incubation, 5 µL of samples were placed on copper grids for 60 sec; excess solutions were removed by using filter papers to touch the edge of the grids. The grids were washed with distilled water. After air-drying, the specimens were examined under H800 transmission electron microscope (TEM) (Hitachi, Japan) at an instrumental magnification of 100,000×. The length of Aβ1–40 oligomers was measured.
Cell culture and neurotoxicity assay
SH-SY5Y cells and VSC 4.1 cells (obtained from Peking University Health Science Center) were cultured in Dulbecco’s modified Eagle’s medium (DMEM)/F12 medium with 10% fetal bovine serum (Gibco, Carlsbad, CA) in 5% CO2 humidified atmosphere at 37°C. The culture medium was changed every 48 h.
Our preliminary test found the cytotoxicity was significant at 24 h after 200 µM H2O2 was added to cultured SH-SY5Y and VSC 4.1 (data not shown). SH-SY5Y cells and VSC 4.1 cells were separately plated in 96-well plates at a density of 1.67 × 104 cells/mL. Cells were pre-incubated with various concentrations of SBE in culture medium for 24 h. The final concentrations of SBE ranged between 0.3 and 10 µM. After that, cells were exposed to 200 μM H2O2 for another 24 h. After washing with PBS, 10 µL MTT (5 mg/mL) solution was added and incubated for 4 h. Then, formazan crystals were dissolved with sodium dodecyl sulfate solution and measured by VICTOR3TMV Multilabel Counter (PerkinElmer, Boston, MA) multilabel counter at a wavelength of 570 nm. The cell viability was expressed at percentage of viable cells in wells with SBE as compared with cells in control wells.
Data analysis
Each EC50 value was calculated by the Bliss method using the Lan Zhou Pharmaceutical software series for EC50 data processing, version 1.01 (Lan Zhou Inc., Sheng Yang, China). Statistical comparisons were made by one-way ANOVA analysis with Dunnett’s post hoc test using SPSS software (version 13.0). *P < 0.05, **P < 0.001. All values were represented as mean ± SD.
Results
We compared the effects of SBE, salvianic acid A, borneol, and a mixture of salvianic acid A and borneol on the destabilization of Aβ1–40 oligomers. As shown in , Aβ1–40 oligomers was significantly reduced in the presence of SBE and the mixture (salvianic acid A and borneol, 1:1), but the destabilizing activity of salvianic acid A or borneol alone was not significant. Though the mixture of salvianic acid A and borneol was effective, SBE showed stronger destabilizing effect.
Figure 2. The destabilization of Aβ1–40 oligomers measured by the thioflavin T fluorescence assay. Preformed Aβ1–40 oligomers were incubated in the presence of salvianic borneol ester (SBE) (10 µg/mL), borneol (10 µg/mL), salvianic acid A (10 µg/mL), or the mixture of borneol and salvianic acid A (1:1, both 5 µg/mL) for 30 min. Values are reported as mean ± SD (n = 3). *P < 0.05, **P < 0.001 versus control (Aβ1–40 oligomers without treatment) (one-way ANOVA analysis with Dunnett’s post hoc test).
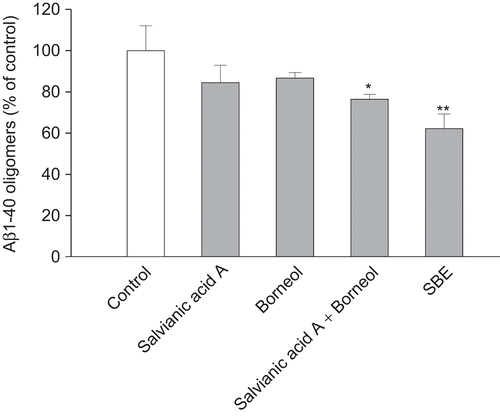
SBE destabilized preformed Aβ1–40 oligomers. Significant destabilizing effects of SBE were observed dose-dependently () and time-dependently (). The EC50 value was 19.4 µg/mL. Furthermore, TEM was used to confirm the form of the aggregates and the effect of SBE on Aβ1–40 oligomers. showed the Aβ1–40 oligomers without treatment, and the mean length of oligomers was 85.2 ± 6.3 nm. As is shown in , in the presence of SBE, less Aβ1–40 oligomers was observed visually by TEM. The Aβ1–40 oligomers were destabilized by SBE dose-dependently.
Figure 3. Effects of salvianic borneol ester (SBE) on Aβ1–40 oligomers destabilization measured by the thioflavin T fluorescence assay. SBE destabilized Aβ1–40 oligomers (A) in dose-dependent manner (incubation for 30 min) and in time-dependent manner (SBE at 10 µg/mL) (B). Values are reported as mean ± SD (n = 3). Significant destabilizing activities of SBE were observed dose-dependently at concentration above 5 µg/mL (P < 0.001 vs. control) and time-dependently at and above 1 h (P < 0.001 vs. control) (one-way ANOVA analysis with Dunnett’s post hoc test).
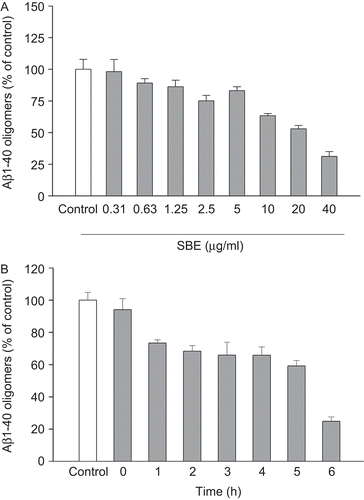
Figure 4. Electron micrographs of Aβ1–40 oligomers. Aβ1–40 oligomers were incubated in the absence (A) or presence of salvianic borneol ester (SBE) 2.5 µg/mL (B), 20 µg/mL (C), or 40 µg/mL (D). Magnification: 100,000×; scale bar = 170 nm.
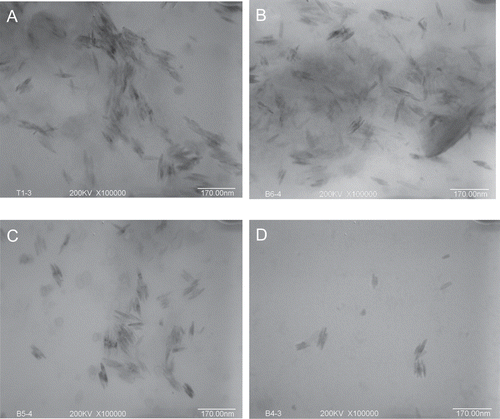
We then examined the neuroprotective effects of SBE on SH-SY5Y cells and VSC4.1 cells. We pre-incubated SH-SY5Y and VSC 4.1 cells with various doses of SBE for 24 h, then exposed cells to 200 µM H2O2 solution for another 24 h and cell viability was finally tested. As shown in , H2O2-induced toxicity was attenuated after cells were incubated with SBE in concentration-dependent manner. Compared with control, SBE at 1, 3, and 10 µM significantly increased the cell viability to 47.0 ± 7.0, 54.7 ± 5.4, and 62.2 ± 3.6%, respectively, in SH-SY5Y cells (), to 39.7 ± 6.0, 59.8 ± 7.0, and 65.9 ± 3.6%, respectively, in VSC4.1 cells (). The protective effects could also be confirmed by the morphological observation (–). After exposure to H2O2, neurite degeneration of cells was evident and a reduction in the number of cells was observed compared with control. In groups of SBE pretreated cells, the neurite degeneration was attenuated and the number of cells increased.
Figure 5. The protection of salvianic borneol ester (SBE) against toxicity induced by H2O2 in SH-SY5Y cells and VSC 4.1 cells. MTT cell viability assay for SH-SY5Y cells (A) and VSC 4.1 cells (B). Values are reported as mean ± SD (n = 4–6). *P < 0.05, **P < 0.001 versus H2O2 alone. ##P < 0.001 versus control (one-way ANOVA analysis with Dunnett’s post hoc test). Representative photomicrographs showing SH-SY5Y cells exposed to vehicle (C), H2O2 (D), or both H2O2 and 10 µM SBE (E); VSC 4.1 cells exposed to vehicle (F), H2O2 (G), or both H2O2 and 10 µM SBE (H). Magnification: 400×.
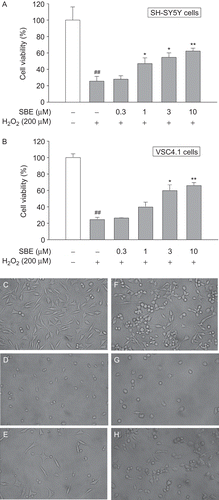
Discussion
A conventional idea for searching a new lead compound from traditional Chinese medicine (TCM) is to separate active ingredients from a single herb. We adopt a new idea to explore new bioactive compounds, which are combined by two compounds of TCM. SBE is a compound formed as a product of synthesis of salvianic acid A and borneol, based on classical SM formulas that are widely used in TCM. This study provides the first evidence that SBE destabilizes preformed Aβ oligomers and protects SH-SY5Y cells and VSC4.1 cell against H2O2-induced cytotoxicity. According to the data, the destabilizing activity of salvianic acid A or borneol alone was not significant. Though the mixture of salvianic acid A and borneol could destabilize Aβ oligomers, the effect of SBE was more significant. SBE destabilized Aβ1–40 oligomers in dose- and time-dependent manners. We also found SBE could destabilize Aβ1-42 oligomers in a higher concentration and time-dependently (data not shown). In addition, it has been reported that cryptotanshinone extracted from SM strongly attenuated amyloid plaque deposition in transgenic mice and decreased Aβ generation in vitro (CitationYu et al., 2007; CitationMei et al., 2009). We found that SBE destabilized Aβ1–40 oligomers at a similar level with cryptotanshinone in ThT fluorescence assay (data not shown). Based on these findings, it is reasonable to conclude that SBE is a novel alternative template for developing therapeutic agents of AD and research for new compounds through combination of chemical components from TCM is one of viable strategies of drug discovery.
Accumulating evidence suggests that Aβ oligomers are the most neurotoxic forms among Aβ aggregates (CitationDahlgren et al., 2002). It was reported that oligomers inhibit neuronal viability 10-fold more than fibrils and ~40-fold more than unaggregated peptides. Protofibrils, one kind of oligomers, are <200 nm in length and bind ThT with increased fluorescence intensity (CitationJan et al., 2010). Fibrils have been described as >1 μm long and Aβ monomers do not change the fluorescence intensity of ThT (CitationJan et al., 2010). In our study, the Aβ aggregates are about 85 nm in length and bind ThT. These results suggest that the Aβ aggregates formed in our experiment are just the protofibrils possessing strong neurotoxicity. SBE could destabilize oligomers, the most toxic Aβ aggregates, which enhances the value of SBE to be a potential AD drug.
Like most other compounds destabilizing Aβ fibrils or oligomers, SBE has aromatic group and phenolic hydroxy groups that have been considered as an important structural feature for an effective anti-amyloid agent (CitationPorat et al., 2006; CitationHawkes et al., 2009). On the one hand, it was reported that some polyphenolic compounds preventing amyloid accumulation have two aromatic end groups and the length and flexibility of the linker region are related to inhibitory activity (CitationReinke Gestwicki, 2007). On the other hand, some nonsteroidal anti-inflammatory drugs (such as ibuprofen) that have an aromatic-based hydrophobic structure with some fused ring structures and methyl and/or carboxyl groups can also destabilize Aβ aggregates (CitationHirohata et al., 2008). Therefore, the structures of amyloid-destabilizing compounds are various and the exact destabilizing mechanisms are unclear. In the present study, salvianic acid A and borneol alone could not destabilize Aβ1–40 oligomers, may be because their sizes were too small to fully occupy the binding pocket of oligomers. When they exist simultaneously in the form of mixture or compound SBE, they occupied more binding sites in the binding pocket and destabilized Aβ1–40 oligomers. SBE might bind to oligomers more stably and showed stronger destabilizing effect.
SH-SY5Y cells are human neuroblastoma cells that are commonly used nerve cell line in the study of neurodegenerative disease. VSC4.1 cells are motor neuron hybridoma cells that have some characteristics of normal cells. We used these two kinds of cell lines as H2O2-injured cell models. SBE similarly protected SH-SY5Y and VSC 4.1 against H2O2-induced cytotoxicity. SBE at 0.3–10 μM was not cytotoxic, which was detected in our preliminary study (data not shown). In these H2O2-injured cell models, the damage induced by H2O2 was very serious (only about 20% cell viability was left). Cell viability of cells treated with 10 μM SBE reached about 60%, approximately three times the cell viability of the H2O2-injured cells without SBE. SBE showed strong antioxidative bioactivity in both cells.
Based on the bifunctional activities of anti-amyloid and neuroprotection, it is reasonable to suggest that SBE will be an alternative lead compound for the development of new drugs against AD. Additional studies are needed to elucidate the mechanisms underlying the anti-amyloid and antioxidative effects of SBE and to confirm its efficacy in an animal model of AD. Toxicological research of SBE is also quite necessary.
Conclusion
In this study, we showed SBE destabilized preformed Aβ oligomers and protected SH-SY5Y cells and VSC4.1 cells against H2O2-induced toxicity. These results suggest that SBE may have therapeutic potential for the treatment of AD.
Acknowledgements
This work was supported by the National Natural Science Foundation of China (30672491) and by the Beijing New Medical Discipline Based Group (XK100270569). We are grateful to Prof. Xiaohui Zheng for generously providing SBE.
Declaration of interest
There is no conflict of interest to declare.
References
- Behl C. (1997). Amyloid beta-protein toxicity and oxidative stress in Alzheimer’s disease. Cell Tissue Res, 290, 471–480.
- Bi J, Jiang B, Liu JH, Lei C, Zhang XL, An LJ. (2008). Protective effects of catalpol against H2O2-induced oxidative stress in astrocytes primary cultures. Neurosci Lett, 442, 224–227.
- Cleary JP, Walsh DM, Hofmeister JJ, Shankar GM, Kuskowski MA, Selkoe DJ, Ashe KH. (2005). Natural oligomers of the amyloid-beta protein specifically disrupt cognitive function. Nat Neurosci, 8, 79–84.
- Dahlgren KN, Manelli AM, Stine WB Jr, Baker LK, Krafft GA, LaDu MJ. (2002). Oligomeric and fibrillar species of amyloid-beta peptides differentially affect neuronal viability. J Biol Chem, 277, 32046–32053.
- Fujiwara H, Iwasaki K, Furukawa K, Seki T, He M, Maruyama M, Tomita N, Kudo Y, Higuchi M, Saido TC, Maeda S, Takashima A, Hara M, Ohizumi Y, Arai H. (2006). Uncaria rhynchophylla, a Chinese medicinal herb, has potent antiaggregation effects on Alzheimer’s beta-amyloid proteins. J Neurosci Res, 84, 427–433.
- Han M, Zhao CQ, Wang YY. (2009). β-(3,4-Dihydroxyphenyl)-α-hydroxypropanoates as amyloid peptide inhibitors and their preparation, pharmaceutical compositions and use in the treatment of neurodegenerative diseases. Faming Zhuanli Shenqing Gongkai Shuomingshu, Chinese patent, 21 pp. CN 101475483, A 20090708, CAN 151:220848, AN 2009: 840155.
- Hardy JA, Higgins GA. (1992). Alzheimer’s disease: The amyloid cascade hypothesis. Science, 256, 184–185.
- Hawkes CA, Ng V, McLaurin J. (2009). Small molecule inhibitors of Aβ-aggregation and neurotoxicity. Drug Dev Res, 70, 111–124.
- Hirohata M, Ono K, Yamada M. (2008). Non-steroidal anti-inflammatory drugs as anti-amyloidogenic compounds. Curr Pharm Des, 14, 3280–3294.
- Jakob-Roetne R, Jacobsen H. (2009). Alzheimer’s disease: From pathology to therapeutic approaches. Angew Chem Int Ed Engl, 48, 3030–3059.
- Jan A, Hartley DM, Lashuel HA. (2010). Preparation and characterization of toxic Abeta aggregates for structural and functional studies in Alzheimer’s disease research. Nat Protoc, 5, 1186–1209.
- Klunk WE, Jacob RF, Mason RP. (1999). Quantifying amyloid beta-peptide (Abeta) aggregation using the Congo red-Abeta (CR-abeta) spectrophotometric assay. Anal Biochem, 266, 66–76.
- Lin HQ, Ho MT, Lau LS, Wong KK, Shaw PC, Wan DC. (2008). Anti-acetylcholinesterase activities of traditional Chinese medicine for treating Alzheimer’s disease. Chem Biol Interact, 175, 352–354.
- Lleó A, Greenberg SM, Growdon JH. (2006). Current pharmacotherapy for Alzheimer’s disease. Annu Rev Med, 57, 513–533.
- Mecocci P, Mariani E, Polidori MC, Hensley K, Butterfield DA. (2008). Antioxidant agents in Alzheimer’s disease. Cent Nerv Syst Agents Med Chem, 8, 48–63.
- Mei Z, Zhang F, Tao L, Zheng W, Cao Y, Wang Z, Tang S, Le K, Chen S, Pi R, Liu P. (2009). Cryptotanshinone, a compound from Salvia miltiorrhiza modulates amyloid precursor protein metabolism and attenuates beta-amyloid deposition through upregulating alpha-secretase in vivo and in vitro. Neurosci Lett, 452, 90–95.
- Porat Y, Abramowitz A, Gazit E. (2006). Inhibition of amyloid fibril formation by polyphenols: Structural similarity and aromatic interactions as a common inhibition mechanism. Chem Biol Drug Des, 67, 27–37.
- Reinke AA, Gestwicki JE. (2007). Structure-activity relationships of amyloid beta-aggregation inhibitors based on curcumin: influence of linker length and flexibility. Chem Biol Drug Des, 70, 206–215.
- Ren Y, Houghton PJ, Hider RC, Howes MJ. (2004). Novel diterpenoid acetylcholinesterase inhibitors from Salvia miltiorhiza. Planta Med, 70, 201–204.
- Shah RS, Lee HG, Xiongwei Z, Perry G, Smith MA, Castellani RJ. (2008). Current approaches in the treatment of Alzheimer’s disease. Biomed Pharmacother, 62, 199–207.
- Tabner BJ, Turnbull S, El-Agnaf OM, Allsop D. (2002). Formation of hydrogen peroxide and hydroxyl radicals from A(beta) and alpha-synuclein as a possible mechanism of cell death in Alzheimer’s disease and Parkinson’s disease. Free Radic Biol Med, 32, 1076–1083.
- Yankner BA, Duffy LK, Kirschner DA. (1990). Neurotrophic and neurotoxic effects of amyloid beta protein: Reversal by tachykinin neuropeptides. Science, 250, 279–282.
- Yu XY, Lin SG, Chen X, Zhou ZW, Liang J, Duan W, Chowbay B, Wen JY, Chan E, Cao J, Li CG, Zhou SF. (2007). Transport of cryptotanshinone, a major active triterpenoid in Salvia miltiorrhiza Bunge widely used in the treatment of stroke and Alzheimer’s disease, across the blood–brain barrier. Curr Drug Metab, 8, 365–378.
- Zhang L, Yu H, Sun Y, Lin X, Chen B, Tan C, Cao G, Wang Z. (2007). Protective effects of salidroside on hydrogen peroxide-induced apoptosis in SH-SY5Y human neuroblastoma cells. Eur J Pharmacol, 564, 18–25.
- Zhao B. (2009). Natural antioxidants protect neurons in Alzheimer’s disease and Parkinson’s disease. Neurochem Res, 34, 630–638.
- Zheng XH, Zhang QS, Wang SX, Zhao XF. (2007). Preparation of β-phenyl-α-hydroxypropionic acid derivatives for treatment of cardiovascular and cerebrovascular diseases. PCT Int Appl, 46 pp. WO 2007131446, A1 20071122, CAN 150:514768, AN 2009:647929.
- Zhou L, Zuo Z, Chow MS. (2005). Danshen: An overview of its chemistry, pharmacology, pharmacokinetics, and clinical use. J Clin Pharmacol, 45, 1345–1359.
- Zhu X, Su B, Wang X, Smith MA, Perry G. (2007). Causes of oxidative stress in Alzheimer disease. Cell Mol Life Sci, 64, 2202–2210.