Abstract
Context: Cisplatin-induced nephrotoxicity has been accepted as an important obstacle for efficient cisplatin-based chemotherapy. Silymarin from seeds of milk thistle [Silybum marianum L. (Asteraceae)] has been shown to possess various potential pharmacological properties; however, whether or not this agent selectively protects renal cells from cisplatin-induced cell death with no interfering effect on cancer cells is not clear.
Objective: Potential of silymarin in protection of cisplatin-induced renal cell death without compromising effect on anticancer activity of cisplatin was demonstrated in this study.
Materials and methods: Cisplatin-induced cell death was evaluated in human proximal tubular HK-2, lung carcinoma H460, and melanoma G361 cells using MTT, Hoechst 33342, and propidium iodide assays.
Results: Cisplatin induced both apoptosis and necrosis in HK-2 cells and caused a decrease in cell viability by ~40% and 60% at the doses of 25 and 100 µM, respectively. Pretreatment with 25–200 µM of silymarin significantly protected against cisplatin-induced cell death in a dose-dependent manner. In contrast, pretreatment of silymarin (25–100 µM) caused no significant change on cisplatin-induced cell death in H460 cells but significantly potentiated cisplatin-induced apoptosis in G361 cells.
Discussion and conclusion: These findings reveal the selectivity of silymarin in protection of renal cells from cisplatin-induced cell death and could be beneficial for the development of this considerately safe compound as a renoprotective agent.
Introduction
Cisplatin [cis-diamminedichloroplatinum (II)] is accepted as one of the most prescribed drugs for the treatment of many solid tumors such as testicular, head and neck, lung, melanoma, and ovarian cancers (CitationPatrick & Lawrence, 1984; CitationAtallah & Flaherty, 2005; CitationKelland, 2007). However, nephrotoxicity-induced by cisplatin has been shown to be an important obstacle for efficient cisplatin-based chemotherapy in many patients (CitationPatrick & Lawrence, 1984; CitationLaunay-Vacher et al., 2008; CitationPabla & Dong, 2008). Co-administration of cisplatin and effective nephroprotective agents protecting renal cells from cisplatin-induced damage while having minimal effect on cisplatin actions against cancer cells should be a recommended part of the treatment regimen of cancer. Silymarin, a mixture of flavonolignans extracted from seeds of milk thistle [Silybum marianum L. (Asteraceae)], has long been used for the treatment of various liver diseases and the hepatoprotection against several toxic substances (CitationMuriel et al., 1992; CitationFlora et al., 1998; CitationSaller et al., 2001; CitationMansour et al., 2006). Silymarin has been shown to possess various potential pharmacological properties including the following: scavenge reactive oxygen species (CitationMira et al., 1994; CitationSvobodová et al., 2006; CitationAsghar and Masood, 2008), inhibit lipid peroxidation (CitationBosisio et al., 1992; CitationMuriel et al., 1992), increase glutathione and superoxide dismutase levels (CitationFehér et al., 1987; CitationValenzuela et al., 1989), induce tissue regeneration (CitationSonnenbichler et al., 1999), and promote DNA, RNA, and protein synthesis (CitationSonnenbichler & Zetl, 1986; CitationSonnenbichler et al., 1999). Moreover, silymarin exerts cancer-suppressing activity in many kinds of tumor cells such as lung, prostate, cervical, leukemia, and breast cancers (CitationZi et al., 1998; CitationSharma et al., 2003; CitationHuang et al., 2005; CitationDeep et al., 2006; CitationZhong et al., 2006). Although silymarin has potential activities, namely, antioxidant and tissue-stimulating effects, whether or not this agent selectively protects renal cell damage without interfering effect on cancer cells is not known. The present study aims to investigate the effects of silymarin for its potential to be developed for further use in benefiting cisplatin-based chemotherapy.
Materials and methods
Cells and reagents
Human proximal tubular epithelial HK-2, human melanoma G361, and human lung cancer epithelial H460 cells were obtained from the American Type Culture Collection (ATCC, Manassas, VA). HK-2 and G361 cells were cultured in Dulbecco’s modified Eagle’s medium (DMEM), whereas H460 cell was cultured in RPMI 1640 medium in a 5% CO2 environment at 37°C. All media were supplemented with 2 mM l-glutamine, 10% fetal bovine serum, and 100 units/mL of penicillin/streptomycin (Gibco, Gaithersburg, MA). Cisplatin, silymarin, 3-(4,5-dimethylthiazol-2-yl)-2,5-diphenyltetrazolium bromide (MTT), Hoechst 33342, and propidium iodide (PI) were obtained from Sigma Chemical, Inc. (St. Louis, MO).
Cytotoxicity assay
Cell viability was determined by the MTT colorimetric assay. In brief, cells in 96-well plate were incubated with 500 μg/mL of MTT for 4 h at 37°C. The supernatant was then removed and dimethyl sulfoxide (DMSO) was added to dissolve the formazan product. The intensity was spectrophotometrically measured at 570 nm using an ELISA reader (Anthros, Durham, NC). All analyses were performed in at least three independent replicate cultures. The absorbance ratio of treated to non-treated control cells was calculated and presented in terms of relative cell viability.
Apoptosis and necrosis assay
Apoptotic and necrotic cell death was detected by Hoechst 33342 and PI co-staining. After specific treatments, cells were stained with 10 µM of the Hoechst and 5 µg/mL PI dye for 30 min at 37°C. The apoptotic cells having condensed chromatin and/or fragmented nuclei stained by Hoechst 33342 and PI-positive necrotic cells were visualized and scored under a fluorescence microscope (Olympus IX51 with DP70).
Statistical analysis
Data were presented as the means ± SD from three or more independent experiments. Statistical analysis was performed by Student’s t-test at a significance level of P < 0.05.
Results
Cisplatin induces cell death
According to clinical evidence, cisplatin-induced nephrotoxicity is a severe side effect, which frequently limits the clinical use of cisplatin in patients (CitationPatrick & Lawrence, 1984; CitationLaunay-Vacher et al., 2008; CitationPabla & Dong, 2008). Cisplatin was recommended for the treatment of many tumors including lung cancer and melanoma (CitationPatrick & Lawrence, 1984; CitationAtallah & Flaherty, 2005; CitationKelland, 2007). We thus demonstrated cisplatin-induced toxicity and selectivity in protection of silymarin in human proximal tubular epithelial HK-2, human lung cancer epithelial H460, and human melanoma G361. First, we characterized cisplatin-induced cell death in these cells by leaving the cells untreated or treated with various concentrations of cisplatin (5–300 µM). Cell viability was then measured after 24 h by the MTT assay. – demonstrates that cisplatin treatment caused a dose-dependent decrease in cell viability with ~50% of the cells remaining viable at cisplatin concentrations of 50, 100, and 100 µM in HK-2, H460, and G361 cells, respectively. As apoptosis and necrosis have been shown to be two major modes of cisplatin-induced cell death, we further identified mode of cell death in response to cisplatin treatment in these cells. A Hoechst 33342 assay was performed for apoptosis detection, and propidium iodide staining assay was used for necrosis detection. – shows that the number of apoptotic cells exhibiting intense nuclear fluorescence and DNA condensation and necrotic cells with positive PI staining were increased in response to cisplatin treatment in HK-2 and H460 cells, whereas only Hoechst-positive cells were observed in G361 cells. These results suggest that cisplatin-induced cytotoxicity in HK-2 and H460 cells was due to apoptosis and necrosis cell death, although cisplatin induced G361 cell damage mainly through the apoptosis mechanism.
Figure 1. Cytotoxic effect of cisplatin on HK-2, H460, and G361 cells. (A–C) Cells were treated with various concentrations of cisplatin (0–300 µM) for 24 h and cell viability was measured by MTT assay. Values are means ± SD of three independent experiments, *P < 0.05 versus non-treated control. (D–F) Apoptotic and necrotic cells were detected by Hoechst 33342 and propidium iodide co-staining assay and examined under a fluorescence microscope.
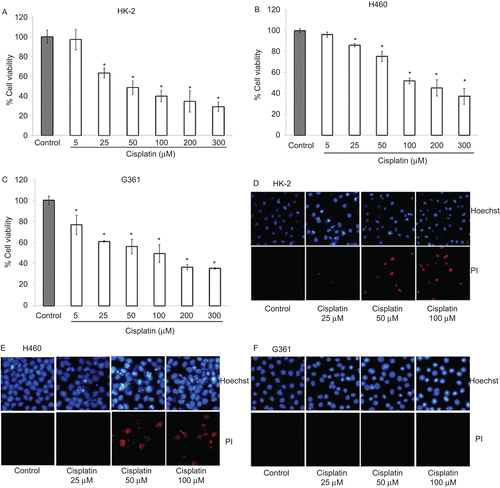
Silymarin exhibits anticancer activity against lung cancer H460 and melanoma G361 cells
To determine whether silymarin has direct anticancer activity, HK-2, H460, and G361 cells were incubated with various concentrations of silymarin (25–200 µM) for 24 h, and cell viability and mode of cell death were analyzed by MTT, Hoechst 33342 staining, and PI staining assays. indicates that silymarin at low concentrations (25–50 µM) has neither cytotoxic nor proliferative effects on HK-2 cells. However, at increased concentrations of up to 100 and 200 µM, silymarin caused a significant increase in renal epithelial HK-2 cell proliferation. Treatment of the H460 cells with low concentrations of silymarin showed only a minimal effect on cell viability, but at concentrations of 200 µM, silymarin caused a significant toxic effect on these cells with ~30% reduction of cell viability (). In melanoma cells, silymarin exhibited similar dose-dependent cytotoxic effects with ~50% reduction in cell viability in response to 200 µM of silymarin treatment (). Accordingly, results of Hoechst 33342 and PI co-staining assays in – show that treatment with silymarin alone caused no significant apoptosis and necrosis in HK-2 cells, whereas silymarin treatment caused a dose-dependent increase in the number of apoptotic cells in H460 and G361 cells. Notably, at a high concentration (200 µM), significant necrosis was detected in H460 cells, whereas only minimal necrosis was detected in G361 cells.
Figure 2. Effect of silymarin on HK-2, H460, and G361 cells. (A–C) Cells were treated with various concentrations of silymarin (0–200 µM) for 24 h and cell viability was measured by MTT assay. Values are means ± SD of three independent experiments, *P < 0.05 versus non-treated control. (E–F) Nuclear morphology of apoptosis and necrosis detected by Hoechst 33342 and propidium iodide assay.
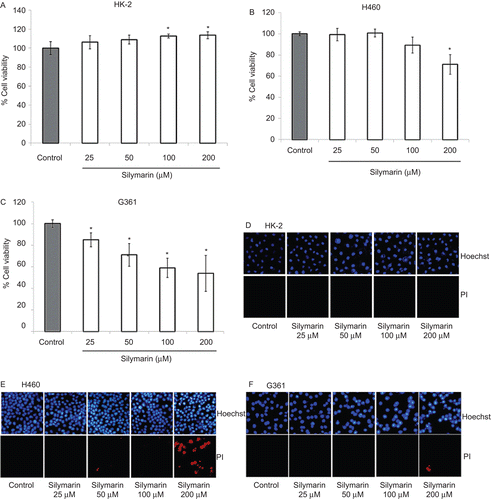
Silymarin protects HK-2 cells from cisplatin-induced cytotoxicity
Cells were pre-incubated with various concentrations of silymarin (25–200 µM) for 1 h and treated with various concentrations of cisplatin (25–100 µM). After 24-h incubation, cell viability and cell death detection were performed. Treatment of the HK-2 cells with cisplatin alone at concentrations of 25, 50, and 100 µM caused a reduction in cell survival by approximately 40, 50, and 60%, respectively. shows that pretreatment of HK-2 cells with silymarin (25–200 µM) significantly prevented the loss of cell viability induced by cisplatin treatment in the concentration range of 25–100 µM. In addition, the results of Hoechst 33342 and PI assays show that silymarin succeeded in decreasing both apoptosis and necrosis induced by cisplatin in HK-2 cells (–). These results indicate that silymarin treatment was able to ameliorate cisplatin-induced HK-2 cell damage.
Figure 3. Protective effect of silymarin on cisplatin-induced HK-2 cell death. (A) Cells were pretreated with various concentrations of silymarin (25–200 µM) for 1 h prior to 24-h cisplatin exposure. Cell viability was measured by MTT assay. Values are means ± SD of three independent experiments, *P < 0.05 versus non-treated control, and #P < 0.05 versus cisplatin-treated control. (B–D) Nuclear morphology of apoptosis and necrosis detected by Hoechst 33342 and propidium iodide assay.
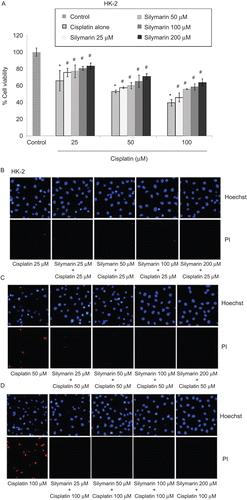
Silymarin potentiates cisplatin-induced melanoma cell damages while causing minimal effect on cisplatin-induced lung cancer cell damages
A limitation of using antioxidants for the protection of normal cell toxicities in chemotherapy is that antioxidants frequently attenuated anticancer activities of drugs on tumor cells (CitationEastman, 1987; CitationRoller & Weller, 1998; CitationMiyajima et al., 1999). We further investigated whether silymarin could reduce efficacy of cisplatin in the induction of cancer cell death. Cells were left untreated or pretreated with various concentrations of silymarin (25–200 µM) for 1 h and treated with various concentrations of cisplatin (25–100 µM). After 24-h incubation, cell viability, apoptosis, and necrosis were determined. shows that silymarin at the concentrations of 100 µM and lower caused no significant change on lung cancer cell viability after cisplatin treatment. As the dose of silymarin was increased up to 200 µM, silymarin dramatically enhanced the cytotoxic effect of cisplatin with ~2-fold reduction as compared with the cisplatin-treated control. In melanoma, treatment with cisplatin alone caused cell survival reduction by approximately 40–50% at the concentration range of 25–100 µM. Addition of silymarin in cisplatin-treated melanoma cells significantly potentiated cisplatin-induced toxicity in a concentration-dependent manner ().
Figure 4. Effect of silymarin on cisplatin-induced H460 and G361 cell death. (A and B) Cells were pretreated with various concentrations of silymarin (25–200 µM) for 1 h prior to 24-h cisplatin treatment. Cell viability was measured by the MTT assay. Values are means ± SD of three independent experiments, *P < 0.05 versus non-treated control, and #P < 0.05 versus cisplatin-treated control. (C–H) Nuclear morphology of apoptosis and necrosis detected by Hoechst 33342 and propidium iodide assay.
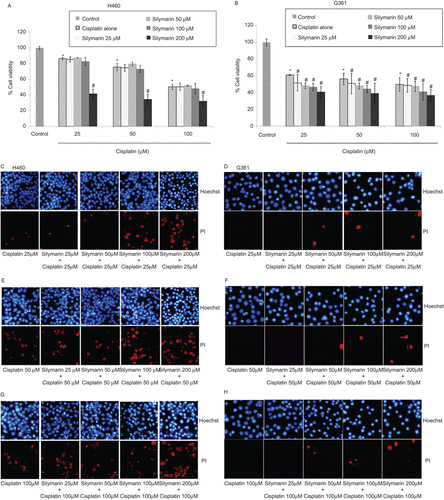
The result of Hoechst 33342 and PI staining assays confirmed that in H460 cells, pretreatment of low concentrations silymarin caused no significant change in mode and quantity of cell death in response to cisplatin treatment. Consistently, at 200 µM silymarin, the number of both apoptotic and necrotic cells significantly increased (, , and 4G). Unlike H460 cells, co-treating the G361 cells with silymarin and cisplatin mainly caused an increase in apoptotic cell death. Our results suggest that the addition of silymarin in cisplatin treatment not only protects against human renal cell damage, but also, at least, either does not interfere with the cytotoxic mechanisms of cisplatin or enhances the response of cancer cells to cisplatin-induced cell death.
Discussion
The development of nephrotoxicity during cisplatin treatment has been shown to be a major limitation of efficient therapy (CitationPatrick & Lawrence, 1984; CitationLaunay-Vacher et al., 2008; CitationPabla & Dong, 2008). Cisplatin induces cytotoxicity by the mechanisms involved in DNA-adduct formation and/or intracellular reactive oxygen species (ROS) induction (CitationReedijk & Lohman, 1985; CitationMiyajima et al., 1997; CitationWang & Lippard, 2005; CitationBragado et al., 2007). In cancer cells, DNA-adduct formation seems to be a major mechanism of cisplatin-mediated cell death since the cells have evolved mechanisms such as increase of superoxide dismutase or glutathione levels to protect themselves from intrinsic high oxidative stress resulted from abnormal metabolism (CitationValko et al., 2007; CitationGibellini et al., 2010). Although a sufficient number of studies have indicated that cisplatin-induced renal cell damage is mainly due to its ability to generate oxidative stress (CitationMatsushima et al., 1998; CitationYang et al., 2002; CitationBaek et al., 2003), introducing an antioxidant could be beneficial in preventing renal cell damage during cisplatin therapy (CitationAjith et al., 2007; CitationSung et al., 2008). Unfortunately, many antioxidants fail to be further developed for the use in clinical treatment since their antioxidant activities frequently attenuate the cytotoxic mechanisms of cisplatin in cancerous cells (CitationEastman, 1987; CitationRoller & Weller, 1998; CitationMiyajima et al., 1999). Silymarin has been shown to have antioxidant activity and capability to protect normal cells including liver and renal cells from several death stimuli (CitationMuriel et al., 1992; CitationSonnenbichler et al., 1999; CitationMansour et al., 2006). However, whether or not this compound could be able to inhibit cisplatin-induced renal cell death without interfering with the effects of cisplatin on the cancer cells is still largely unknown.
Our results indicate that silymarin selectively protected only human renal tubular HK-2 cells from cisplatin-induced apoptosis without compromising the anticancer activity of cisplatin as demonstrated in lung carcinoma and melanoma cells. The mechanism by which silymarin protects HK-2 cells from cisplatin-induced damage is not fully understood. Our results indicate that treatment with cisplatin resulted in renal cell death, and necrosis seemed to be the main mode of cell death in this condition (). Previous studies reported that hydroxyl radicals could induce lipid peroxidation of the cell membrane, finally resulting in cell necrosis (CitationGutteridge, 1984; CitationSalahudeen, 1995; CitationMatsushima et al., 1998; CitationYang et al., 2002). Silymarin has been reported to possess potent scavenging activity against hydroxyl radicals (CitationMira et al., 1994), and consequently protect lipid peroxidation of the cell membrane (CitationBosisio et al., 1992; CitationMuriel et al., 1992). These data could be a possible explanation of our finding that treatment with silymarin inhibited cisplatin-induced necrosis in HK-2 cells. Moreover, silymarin has been shown to increase glutathione level in liver, stomach, and intestine of rat (CitationValenzuela et al., 1989) and superoxide dismutase level in lymphocytes (CitationFehér et al., 1987). Therefore, silymarin may protect against renal cell damage via the mechanism involving its antioxidant properties against intracellular ROS induced by cisplatin. Some studies have reported that silymarin exerts antitumor activities in some cancer cells (CitationZi et al., 1998; CitationSharma et al., 2003; CitationHuang et al., 2005; CitationDeep et al., 2006; CitationZhong et al., 2006) and we further confirmed that silymarin has a direct anticancer activity against melanoma G361 cells. However, silymarin seemed to have only minimal anticancer activity on non-small-lung cancer H460 cells.
The possible reasons for silymarin selectivity in the protection of renal cells while not interfering with the mode of cisplatin action in cancer cells include the following: (i) silymarin possesses direct anticancer activity; (ii) renal cells were shown to be highly susceptible to oxidative stress-induced cell damage, whereas many cancer cells have been shown to resist ROS-induced cell death (CitationAndreoli, 1991; CitationNath & Norby, 2000; CitationGalle, 2001; CitationDjamali, 2007), and the main mechanism of cisplatin-induced cancer cell death is through DNA-adduct formation (CitationReedijk & Lohman, 1985; CitationWang & Lippard, 2005).
In summary, we reported herein for the first time that silymarin exhibited a strong protective effect against cisplatin-induced human renal cells damage, has no significant effect on cisplatin-induced lung carcinoma H460 cells death, and enhanced cisplatin-induced melanoma G361 cell death. These findings may advocate the necessity of developing silymarin, a considerately safe compound, for its potential use in clinical cancer therapy in combination with anticancer agents.
Acknowledgement
The authors are grateful to Chulalongkorn University Centenary Academic Development project for facility support.
Declaration of interest
The authors declare that they have no conflicts of interest.
References
- Ajith TA, Usha S, Nivitha V. (2007). Ascorbic acid and alpha-tocopherol protect anticancer drug cisplatin induced nephrotoxicity in mice: A comparative study. Clin Chim Acta, 375, 82–86.
- Andreoli SP. (1991). Reactive oxygen molecules, oxidant injury and renal disease. Pediatr Nephrol, 5, 733–742.
- Asghar Z, Masood Z. (2008). Evaluation of antioxidant properties of silymarin and its potential to inhibit peroxyl radicals in vitro. Pak J Pharm Sci, 21, 249–254.
- Atallah E, Flaherty L. (2005). Treatment of metastatic malignant melanoma. Curr Treat Options Oncol, 6, 185–193.
- Baek SM, Kwon CH, Kim JH, Woo JS, Jung JS, Kim YK. (2003). Differential roles of hydrogen peroxide and hydroxyl radical in cisplatin-induced cell death in renal proximal tubular epithelial cells. J Lab Clin Med, 142, 178–186.
- Bosisio E, Benelli C, Pirola O. (1992). Effect of the flavonolignans of Silybum marianum L. on lipid peroxidation in rat liver microsomes and freshly isolated hepatocytes. Pharmacol Res, 25, 147–154.
- Bragado P, Armesilla A, Silva A, Porras A. (2007). Apoptosis by cisplatin requires p53 mediated p38alpha MAPK activation through ROS generation. Apoptosis, 12, 1733–1742.
- Deep G, Singh RP, Agarwal C, Kroll DJ, Agarwal R. (2006). Silymarin and silibinin cause G1 and G2-M cell cycle arrest via distinct circuitries in human prostate cancer PC3 cells: A comparison of flavonone silibinin with flavonolignan mixture silymarin. Oncogene, 25, 1053–1069.
- Djamali A. (2007). Oxidative stress as a common pathway to chronic tubulointerstitial injury in kidney allografts. Am J Physiol Renal Physiol, 293, F445–F455.
- Eastman A. (1987). Cross-linking of glutathione to DNA by cancer chemotherapeutic platinum coordination complexes. Chem Biol Interact, 61, 241–248.
- Fehér J, Láng I, Nékám K, Csomós G, Müzes G, Deák G. (1987). Effect of silibinin on the activity and expression of superoxide dismutase in lymphocytes from patients with chronic alcoholic liver disease. Free Radic Res Commun, 3, 373–377.
- Flora K, Hahn M, Rosen H, Benner K. (1998). Milk thistle (Silybum marianum) for the therapy of liver disease. Am J Gastroenterol, 93, 139–143.
- Galle J. (2001). Oxidative stress in chronic renal failure. Nephrol Dial Transplant, 16, 2135–2137.
- Gibellini L, Pinti M, Nasi M, Biasi SD, Roat E, Bertoncelli L, Cossarizza A. (2010). Interfering with ROS metabolism in cancer cells: The potential role of quercetin. Cancers, 2, 1288–1311.
- Gutteridge JM. (1984). Lipid peroxidation initiated by superoxide-dependent hydroxyl radicals using complexed iron and hydrogen peroxide. FEBS Lett, 172, 245–249.
- Huang Q, Wu LJ, Tashiro S, Onodera S, Li LH, Ikejima T. (2005). Silymarin augments human cervical cancer HeLa cell apoptosis via P38/JNK MAPK pathways in serum-free medium. J Asian Nat Prod Res, 7, 701–709.
- Kelland L. (2007). The resurgence of platinum-based cancer chemotherapy. Nat Rev Cancer, 7, 573–584.
- Launay-Vacher V, Rey JB, Isnard-Bagnis C, Deray G, Daouphars M; European Society of Clinical Pharmacy Special Interest Group on Cancer Care. (2008). Prevention of cisplatin nephrotoxicity: State of the art and recommendations from the European Society of Clinical Pharmacy Special Interest Group on Cancer Care. Cancer Chemother Pharmacol, 61, 903–909.
- Mansour HH, Hafez HF, Fahmy NM. (2006). Silymarin modulates cisplatin-induced oxidative stress and hepatotoxicity in rats. J Biochem Mol Biol, 39, 656–661.
- Matsushima H, Yonemura K, Ohishi K, Hishida A. (1998). The role of oxygen free radicals in cisplatin-induced acute renal failure in rats. J Lab Clin Med, 131, 518–526.
- Mira L, Silva M, Manso CF. (1994). Scavenging of reactive oxygen species by silibinin dihemisuccinate. Biochem Pharmacol, 48, 753–759.
- Miyajima A, Nakashima J, Tachibana M, Nakamura K, Hayakawa M, Murai M. (1999). N-Acetylcysteine modifies cis-dichlorodiammineplatinum-induced effects in bladder cancer cells. Jpn J Cancer Res, 90, 565–570.
- Miyajima A, Nakashima J, Yoshioka K, Tachibana M, Tazaki H, Murai M. (1997). Role of reactive oxygen species in cis-dichlorodiammineplatinum-induced cytotoxicity on bladder cancer cells. Br J Cancer, 76, 206–210.
- Muriel P, Garciapiña T, Perez-Alvarez V, Mourelle M. (1992). Silymarin protects against paracetamol-induced lipid peroxidation and liver damage. J Appl Toxicol, 12, 439–442.
- Nath KA, Norby SM. (2000). Reactive oxygen species and acute renal failure. Am J Med, 109, 665–678.
- Pabla N, Dong Z. (2008). Cisplatin nephrotoxicity: Mechanisms and renoprotective strategies. Kidney Int, 73, 994–1007.
- Patrick JL, Lawrence HE. (1984). Diagnosis and treatment. Drugs five years later. Cisplatin. Ann Intern Med, 100, 704–713.
- Reedijk J, Lohman PH. (1985). Cisplatin: Synthesis, antitumour activity and mechanism of action. Pharm Weekbl Sci, 7, 173–180.
- Roller A, Weller M. (1998). Antioxidants specifically inhibit cisplatin cytotoxicity of human malignant glioma cells. Anticancer Res, 18, 4493–4497.
- Salahudeen AK. (1995). Role of lipid peroxidation in H2O2-induced renal epithelial (LLC-PK1) cell injury. Am J Physiol, 268, F30–F38.
- Saller R, Meier R, Brignoli R. (2001). The use of silymarin in the treatment of liver diseases. Drugs, 61, 2035–2063.
- Sharma G, Singh RP, Chan DC, Agarwal R. (2003). Silibinin induces growth inhibition and apoptotic cell death in human lung carcinoma cells. Anticancer Res, 23, 2649–2655.
- Sonnenbichler J, Scalera F, Sonnenbichler I, Weyhenmeyer R. (1999). Stimulatory effects of silibinin and silicristin from the milk thistle Silybum marianum on kidney cells. J Pharmacol Exp Ther, 290, 1375–1383.
- Sonnenbichler J, Zetl I. (1986). Biochemical effects of the flavonolignane silibinin on RNA, protein and DNA synthesis in rat livers. Prog Clin Biol Res, 213, 319–331.
- Sung MJ, Kim DH, Jung YJ, Kang KP, Lee AS, Lee S, Kim W, Davaatseren M, Hwang JT, Kim HJ, Kim MS, Kwon DY, Park SK. (2008). Genistein protects the kidney from cisplatin-induced injury. Kidney Int, 74, 1538–1547.
- Svobodová A, Walterová D, Psotová J. (2006). Influence of silymarin and its flavonolignans on H2O2-induced oxidative stress in human keratinocytes and mouse fibroblasts. Burns, 32, 973–979.
- Valenzuela A, Aspillaga M, Vial S, Guerra R. (1989). Selectivity of silymarin on the increase of the glutathione content in different tissues of the rat. Planta Med, 55, 420–422.
- Valko M, Leibfritz D, Moncol J, Cronin MT, Mazur M, Telser J. (2007). Free radicals and antioxidants in normal physiological functions and human disease. Int J Biochem Cell Biol, 39, 44–84.
- Wang D, Lippard SJ. (2005). Cellular processing of platinum anticancer drugs. Nat Rev Drug Discov, 4, 307–320.
- Yang YS, Kwak IS, Nah HY, Kim YK. (2002). Role of hydroxyl radicals and lipid peroxidation in cisplatin-induced acute renal failure in rabbits. Korean J Nephrol, 21, 213–221.
- Zhong X, Zhu Y, Lu Q, Zhang J, Ge Z, Zheng S. (2006). Silymarin causes caspases activation and apoptosis in K562 leukemia cells through inactivation of Akt pathway. Toxicology, 227, 211–216.
- Zi X, Feyes DK, Agarwal R. (1998). Anticarcinogenic effect of a flavonoid antioxidant, silymarin, in human breast cancer cells MDA-MB 468: induction of G1 arrest through an increase in Cip1/p21 concomitant with a decrease in kinase activity of cyclin-dependent kinases and associated cyclins. Clin Cancer Res, 4, 1055–1064.