Abstract
Context: Grifola frondosa (Polyporaceae), maitake, is a widely consumed edible mushroom in some Asian countries. The fruit bodies and mycelia of maitake have shown different bioactive compounds with anticancer and other therapeutic properties.
Objective: This study evaluated three chemically modified maitake polysaccharide-peptides’ (MPSP) adjuvant effect (in vivo) and anticancer activity (in vitro growth inhibitory effect) compared with crude MPSP from G. frondosa.
Materials and methods: We investigated the possibility of enhancing the adjuvant effect and anticancer effect of crude MPSP by using simple chemical modification methods to convert crude MPSP to phosphorylated, acetylated or esterified MPSPs. The adjuvant effect and growth inhibitory effect were evaluated by C6 cell inoculated rat model with cyclophosphamide (CPA) treatment and in vitro cell viability assay, respectively.
Results: All four tested MPSPs showed significant adjuvant effect to CPA treatment on rats inoculated with C6 cancer cells. In addition, an obvious growth inhibitory effect was observed in C6 cancer cells but not in normal brain cells treated with various forms of MPSPs. Only phosphorylation could significantly (p < 0.05) improve the adjuvant effect (in vivo) and growth inhibitory effect. A same rank order (phosphorylated MPSP > esterified MPSP ≥ acetylated MPSP ≥ crude MPSP) of efficacy was observed in both the in vivo and in vitro assays.
Discussion and conclusion: This study showed chemical phosphorylation could markedly enhance both adjuvant effects and growth inhibitory effects. This study demonstrated the feasibility of enhancing the efficacy of MPSP by using a simple chemical modification method, and this provides a foundation for future study in this area.
Introduction
Grifola frondosa (Polyporaceae), maitake, a widely consumed edible mushroom in some Asian countries, is a basidiomycete. It is also called the king of mushrooms and the hen of the woods (CitationStamets, 2000). The fruit bodies and mycelia of maitake have shown different bioactive compounds with antitumor (CitationSuzuki et al., 1984; CitationLee et al., 2003; CitationCui et al., 2006; Citation2007a,b) and other therapeutic properties, properties such as anti-hyperlipidemia, hypotension, immunomodulation, antiviral and anti-hepatitis (CitationMayell, 2001; CitationGu et al., 2006). For cancer, Maitake D extract exhibits potent immune-enhancing activity, inhibiting both carcinogenesis (initiation of cancer) and metastasis (CitationNanba, 1995). The major antitumor substances found in maitake are attributed to polysaccharides (CitationZhang et al., 2007). These polysaccharides belong to polysaccharide-proteins and have been identified as having various types of glucans as their major component. They are homoglycans [with a basic structure of a (1-6)-β-branched (1-3)-β-d-glucan], heteroglycans and heteroglycan-protein complex (or maitake polysaccharide-peptide, MPSP) (CitationOhno et al., 1985; CitationZhang et al., 2007).
Cancer is one of the leading causes of death around the world due to a lack of general and comprehensive early detection methods, the associated poor prognosis of patients diagnosed in later stages of disease, and the increasing incidence of cancers on a global scale (CitationLi et al., 2009). Indeed, struggles to combat cancer are one of the greatest challenges to mankind. Treatments of cancer include surgery, radiation therapy, chemotherapy, hormonal therapy and combinations of these. In general, chemotherapy is the choice of treatment in the metastatic (advanced) stage of various cancers, such as breast, liver, prostate, lung and brain cancers (CitationGordon & Curtin, 2000; CitationSchuette, 2001; CitationNewton, 2002), and as adjuvant treatment after surgery or radiation (CitationCaval li et al., 2004). However, the most commonly used anticancer drugs, such as cyclophosphamide (CPA), may cause toxicities and side effects that are harmful to the physical, emotional, social, and spiritual well-being of patients (CitationFan et al., 2007; CitationKwilosz et al., 2007). These side effects can result in treatment withdrawal.
Various polysaccharide-proteins are clinically used as an adjuvant to cancer chemotherapy and radiotherapy in China (CitationLiu et al., 1999; CitationOoi & Liu, 2000). The administration of polysaccharide-proteins with a chemotherapeutic agent allows a lower effective dosage, thus producing less toxicity and far fewer side effects to patients (CitationGordon & Curtin, 2000). Furthermore, polysaccharide-proteins have been shown to restore CPA-induced immunosuppression in patients by improving the functions of natural killer cells and increasing the level of interleukin-2 (CitationQian et al., 1997). Moreover, it has been shown that the adjuvant effect of polysaccharide-proteins is related to the reduction of CPA clearance in vivo (CitationChan & Yeung, 2006). Although much effort has been made to understand the underlying mechanism(s) of polysaccharide-proteins as an adjuvant treatment in cancer therapy, the use of polysaccharide-proteins is still limited to the Chinese communities and is not accepted by orthodox medicine. This may explain the small treatment effect of polysaccharide-proteins. Therefore, in the current study, we investigated the possibility of enhancing the adjuvant effect and anticancer effect of polysaccharide-proteins from maitake by using simple chemical modification methods to convert crude MPSP to phosphorylated, acetylated or esterified MPSP. The adjuvant effect and growth inhibitory effect were evaluated by C6 cell inoculated rat model with CPA treatment and in vitro cell viability assay, respectively.
Materials and methods
Chemicals and materials
Celltiter 96 aqueous MTS reagent was purchased from Promega Chemicals Co. (Madison, WI). CPA was obtained from Sigma Chemicals Co. (St. Louis, MO). All other chemicals used were of analytical grade and were purchased from Sigma Chemicals Co. (St. Louis, MO). Cell culture medium, Dulbecco’s modified eagle medium (DMEM), and fetal bovine serum (FBS) were purchased from Gibco Laboratories (Grand Island, NY). Rat Glioma C6 cells were obtained from the American Type Culture Collection (ATCC, Manassas, VA).
Preparation of crude MPSP and chemically modified samples
Crude MPSP was purified from the Maitake D-fraction (Yee Cheong Pharmaceutical Co., Ltd., Hong Kong) by applying the water-soluble polysaccharide fraction to the diethylaminoethyl-Sepharose fast-flow anionic resin (Pharmacia AP, Sweden) column and Sephadex G-100 (Amersham Biosciences, Sweden) using an AKTA purifier (Amersham Biosciences, Sweden). The fractions collected from the column were tested for protein concentration through an UV spectrophotometer at 230 nm and the carbohydrate content was tested by phenol sulfuric acid method. The fractions with the highest readings from both assays were chosen for further concentration using the Centricon® Plus-80. The crude PSP [composed of 89% polysaccharides (73.6% glucose, 4.8% xylose, 2.7% galactose, 1.5% mannose, and 2.4% fucose) and 11% peptides (18 different amino acids, mainly aspartic acid and glutamic acid)] was freeze-dried to complete dryness and underwent different chemical modifications. Various forms of MPSP were characterized by SDS-PAGE before the in vitro and in vivo experiments. Low-range marker sc-2360 (Santa Cruz Biotechnology, Inc., CA) and bovine serum albumin (BSA) were used as reference and control, respectively.
Phosphorylation
Crude MPSP was phosphorylated by a method previously described (CitationInoue et al., 1983). Crude MPSP (1.0 g) was dissolved in formamide (50 mL), tributylamine (5 mL), and polyphosphoric acid (2.5 g). The mixture was stirred for 24 h at room temperature, and then poured into ethanol (300 mL). The resulting precipitate was collected by centrifugation and redissolved in water (50 mL). The pH of the solution was adjusted to 10 with NaOH, and the solution was evaporated at 37°C and dialyzed against de-ionized water. The nondialyzable suspension was concentrated to ~50 mL and filtered. Then, sodium acetate (0.1 g) was added to the filtrate. Phosphorylated MPSP was precipitated with ethanol (600 mL) and collected by centrifugation. Then the sample was dried in oven at 37°C (yield: 0.95 g).
Acetylation
Crude MPSP (1.0 g) was resuspended in 50 mL of tetrahydrofuran. Acetic anhydride (1.0 mL) was added to the suspension at room temperature with occasional shaking. The reaction was allowed to react for 24 h. At the end of the reaction, 1 mL of distilled water was added to convert excess acetic anhydride to acetic acid. The resultant acetylated MPSP was dried in oven at 37°C (yield: 0.87 g).
Esterification
Crude MPSP (1.0 g) was first dissolved in 100 mL of 75% ethanol in a conical flask. Dissolution was facilitated by warming up the ethanol in a boiling water bath. A few drops of dilute HCl (0.1 M) were added to the mixture. The resultant mixture was heated under reflux at about 60–70°C for 48 h in a fume cupboard. After reflux was completed, any excess ethanol or HCl was evaporated in a boiling water bath until it dried. The resultant esterified MPSP was resuspended in water and freeze-dried for further use (yield: 0.85 g).
Cell culture
The rat glioma C6 cell line (CCL 107) was maintained in DMEM supplemented with 10% (v/v) FBS, 100 U/mL penicillin and 100 µg/mL streptomycin (Sigma, St. Louis, MO) on 100-mm culture dishes (SARSTEDT, Newton, NC) at 37°C in a humidified incubator with 5% carbon dioxide air atmosphere. The cells were fed every 2–3 days and subcultured once they reached 70–80% confluence. Since C6 glioma cells were originally derived from rats, rat cerebellar granule (RCG) cells were selected as the normal brain cells for comparison. The cells derived from the cerebella of 8-day-old Sprague-Dawley rat, as described previously (CitationMei et al., 2000), have often been used as a brain cell model in vitro (CitationGorter et al., 1995). Isolated cells were plated onto 35-mm Petri dishes. The DMEM culture medium was supplemented with 10% FBS, glutamine (2 mM), insulin (0.5 g/mL), KCl (25 mM), and 1% of the antibiotic-antimycotic solution. After cell seeding, cytosine β-d-arabinofuranoside (10 μm) was added to the culture medium to inhibit the proliferation of non-neural cells. The cultured RCG cells were maintained in a 5% CO2 incubator for 1–6 days before being used in experiments. The experimental procedures were approved by the Animal Ethical Sub-committee of The Hong Kong Polytechnic University and were consistent with the Guide for the Care and Use of Laboratory Animals Committee to Revise the Guide, Institute of Laboratory Animal Resources Council, Commission on Life Sciences, National Research Council, 1996).
In vivo evaluation of the adjuvant effect of various forms of MPSP on tumor treatment
Fifty-four adult male Sprague-Dawley rats (7 weeks old, 200–220 g) were housed (2–3 rats/cage) in a temperature-controlled (25 ± 2°C) room with regular cycles of 12 h of light and 12 h of darkness. They were fed standard laboratory food (rat chow) for 1 week. Nine of the rats were randomly selected as the Normal group. The rest of the animals were intracerebrally injected with C6 cells as previously described (CitationTseng et al., 1997). Briefly, cell suspensions were prepared in DMEM/5% FBS culture medium at 2 × 104/µl. By using a Hamilton syringe, 5 µl of cell suspension was sterotaxically injected over a 5-min period of time. Tumor inoculation was performed at 4.5 mm from the dural surface and at 4 mm lateral to the bregma suture. Two weeks after the C6 cells inoculation, blood samples were collected from the orbital sinus of the animals. The total sialic acid (TSA) level in serum from each rat was quantified by the periodate-resorcinol assay (CitationInoue et al., 1983). Animals with elevated TSA content were defined as rats with C6 cells successfully transplanted and randomly divided into 5 groups (each, n = 9). They received drug treatment for another 2 weeks. The groups were: CPA [animals treated with CPA (20 mg/kg/day, i.p.)]; CPA + CMPSP [animals treated with CPA (20 mg/kg/day, i.p.] plus crude MPSP (20 mg/kg/day, i.p.)); CPA + PMPSP [animals treated with CPA (20 mg/kg/day, i.p.] plus phosphorylated MPSP (20 mg/kg/day, i.p.)); CPA + AMPSP [animals treated with CPA (20 mg/kg/day, i.p.) plus acetylated MPSP (20 mg/kg/day, i.p.)]; CPA + EMPSP [animals treated with CPA (20 mg/kg/day, i.p.) plus esterified MPSP (20 mg/kg/day, i.p.)]. At the end of the 2-week treatment, serum TSA content in each rat was evaluated again.
In vitro evaluation of growth inhibitory effects of various forms of MPSP
The effects of various forms of MPSP on the inhibition of cell growth were measured by the MTS [3-(4,5-dimethylthiazol-2-yl)-5-(3-carboxymethoxyphenyl)-2-(4-sulfophenyl)-2H-tetrazolium] growth inhibition assay. The MTS growth inhibition assay was performed according to the instructions provided by the manufacturer (Promega, Madison, WI). Briefly, the same number of cells was seeded into each well of a 96-well plate on Day 1. On Day 2, the cells were either treated with different concentrations of various forms of MPSP for 24 h or remained as untreated controls. After the treatment, the culture medium in each well was replaced with drug-free fresh complete medium containing 10 µl of MTS solution and further incubated for 2 h. Optical density of each culture was then recorded at 490 nm using a microplate reader (Bio-Rad, model 550). Each experiment was performed in triplicate. Results are expressed as the percentage growth inhibition with respect to the untreated cells.
Statistical analysis
Data were expressed as means ± SEM and n denotes the number of replications for each data point. Statistical analysis was performed using analysis of variance or Student’s t-test where appropriate. In all cases, differences between treatment groups were considered significant when p < 0.05. All statistical analysis tests were performed by using GraphPad Prism 5.02 for Windows (GraphPad Software, San Diego, CA).
Results
Characterization of various forms of MPSP
Various forms of MPSP were characterized by SDS-PAGE analysis (). The molecular weights of crude MPSP and acetylated MPSP (66.0 kDa) were similar and found to be slightly lighter than that of BSA (66.2 kDa). The other chemical modification methods could significantly reduce the molecular size of the original MPSP to 65.0 kDa and 53.0 kDa in phosphorylated and esterified forms, respectively. Esterified PSP was the lightest form used in the current study.
In vivo evaluating the adjuvant effect of various forms of MPSP on tumor treatment
In order to evaluate the adjuvant effect of various forms of MPSP on anticancer treatment, C6 cells were inoculated into the brain of the animal. The plasma TSA level, a cancer marker, increased significantly in C6 cell-inoculated animals (from 2.70 ± 0.27 µg/mL to 4.26 ± 0.21 µg/mL, p < 0.001) (). After the 2-week treatment of CPA alone, there were no significant changes in the plasma TSA level (p > 0.05). Interestingly, when MPSP was coadministrated with CPA for 2 weeks, the plasma TSA level dropped significantly (p < 0.05). Only phosphorylation could significantly (p > 0.05) enhance the adjuvant effect of crude MPSP. The rank order of various forms of MPSP on reducing plasma TSA level are phosphorylated MPSP (37.4%) > esterified MPSP (34.1%) ≥ acetylated MPSP (29.5%) ≥ crude MPSP (20.8%) ().
Figure 2. The changes in serum total sialic acid (TSA) in the Normal, CPA, CPA + CMPSP, CPA + PMPSP, CPA + AMPSP and CPA + EMPSP groups. Data are expressed as means ± SEM, n = 9. #p < 0.05 and ###p < 0.001 represent significant differences when comparing the serum TSA before and after drug treatment in each group. *p < 0.05 represents significant differences when comparing various treatment groups’ post-treatment serum TSA with that of the CPA + CMPSP. NS refers to no significant difference.
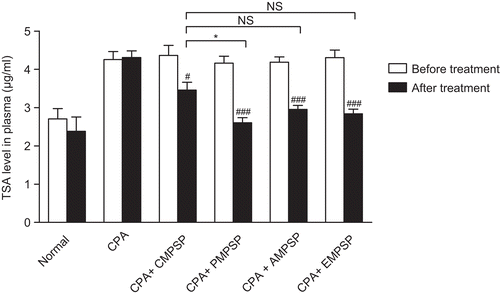
In vitro evaluation of growth inhibitory effects of various forms of MPSP
To test the growth inhibitory effects of various forms of MPSP on C6 cells, a wide concentration range (0.01–100 µg/mL) of various forms of MPSP was used. All tested forms of MPSP showed significant growth inhibitory effects on C6 cells after a 24-h treatment (). The growth inhibitory effects produced by different forms of MPSP were in a concentration-dependent manner. The rank order of maximum growth inhibitory effects on C6 cells are phosphorylated MPSP (36.7%, ) > esterified MPSP (28.7%, ) ≥ acetylated MPSP (27.8%, ) ≥ crude MPSP (21.0%, ). Interestingly, all tested forms of MPSP showed relatively mild (>10%) growth inhibitory effects on normal brain cells (). The data indicate that the growth inhibitory effects of crude MPSP on C6 cells was significantly (p > 0.05) enhanced by phosphorylation (). In addition, the growth inhibitory effects are cell-specific.
Figure 3. Growth inhibitory effects of crude (A), phosphorylated (B), acetylated (C) and esterified (D) MPSP on C6 and normal brain cells after a 24-h treatment. A graph highlighting the growth inhibitory effects of various forms of MPSP at 100 µg/mL (E). Data are expressed as mean ± SEM, n = 3. *p < 0.05, **p < 0.01, and ***p < 0.001 represent significant differences when comparing with its corresponding concentration in the normal brain cells. #p < 0.05 represents significant differences when comparing the growth inhibitory effects of various forms of MPSP with that of the crude MPSP. NS refers to no significant difference.
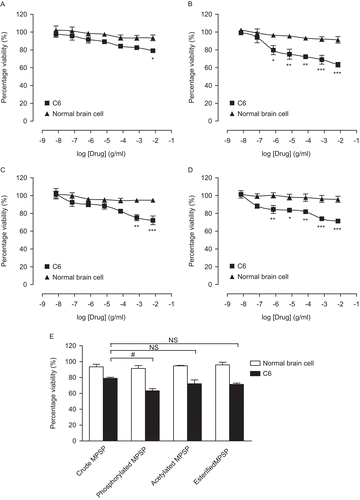
Discussion
Polysaccharide-proteins are mainly used as an adjuvant therapy in cancer treatment (CitationChan & Yeung, 2006). It has demonstrated positive results in the adjuvant treatment of colorectal, gastric, lung, breast, and esophageal cancers (CitationCui et al., 2006; CitationZhang et al., 2007). Our in vivo study, therefore, mainly explored the adjuvant effects of various forms of polysaccharide-proteins with maitake origin. The current results showed that both unmodified and modified forms of MPSP could significantly enhance the anticancer effect (in terms of reducing cancer marker, plasma TSA) of CPA, a commonly used anticancer drug. TSA is a critical cancer marker that increases significantly in cancer patients. The rise in TSA is directly proportional to the degree of metastasis. Effective treatments can lower the increased TSA levels. Plasma TSA is a sensitive marker to detect malignancy and monitor cancer progression. In addition, it can be used to evaluate the efficacy of cancer therapies (CitationTewarson et al., 1993). The beneficial effect of polysaccharide-proteins may be attributed to its immunomodulatory effect (CitationJiménez-Medina et al., 2008) and cytotoxic effect (CitationCui et al., 2006, Citation2007a, Citation2007b). Our study demonstrated for the first time that all chemical modification procedures used hereby could further enhance the anticancer effect of CPA when compared with the original form of MPSP. It was found that phosphorylated MPSP possessed the strongest adjuvant effect on anticancer therapy and its adjuvant effect was significantly (p < 0.05) higher than that of the crude MPSP.
Different forms of MPSP were found to exert obvious inhibitory effects on the growth of C6 cells but they showed no significant effect to normal brain cells in our cell culture study. This is consistent with the results of some other related studies (CitationHattori et al., 2004; CitationLavi et al., 2006; CitationLee et al., 2005; CitationLi et al., 2004; CitationYang et al., 2005). It has been demonstrated that polysaccharide-proteins could inhibit the growth of various cancer cell lines by triggering cell cycle arrest/slowing, induction of caspase-3 expression and apoptosis (Cui et al., Citation2007a, Citation2007b). However, it is not known whether chemically modified MPSP’s growth inhibitory effect is also brought about by similar molecular pathways, and this warrants further study.
Similar to the in vivo study, only phosphorylation could significantly (p < 0.05) improve the growth inhibitory effect of crude MPSP on C6 cells. In addition, the same rank order (phosphorylated MPSP > esterified MPSP ≥ acetylated MPSP ≥ crude MPSP) was observed in both in vivo and in vitro assays. Although the maximum growth inhibition was less than 40%, the cell-specific growth inhibitory effect of MPSP makes it a potential anticancer drug with fewer or even no side effects to cancer patients. This study demonstrated the feasibility to enhance the efficacy of crude MPSP by using simple phosphorylation. It has laid a good foundation for further studies in this area.
In summary, our results showed that chemically phosphorylated MPSP could significantly (p < 0.05) improve anticancer activities (in vivo adjuvant effect and in vitro growth inhibitory effect) in comparison with the original crude MPSP. The same rank order of anticancer activities enhancement (phosphorylated MPSP > esterified MPSP ≥ acetylated MPSP ≥ crude MPSP) was observed in both the in vivo and in vitro assays. However, the precise mechanism(s) of how the structural modification affects MPSP’s biological activity remains unknown. Further investigations are required to understand the mechanism(s) of how modified MPSP inhibits cancer cell growth and the role(s) of modified MPSP in anticancer adjuvant therapy.
Acknowledgments
We thank the Shenzhen Municipal Key Laboratory Advancement Program, Shenzhen, People’s Republic of China, for its support to the project and Ms. Josephine Hong-Man Leung for proofreading the manuscript.
Declaration of interest
This work was also supported by a grant from the Niche Area Research Grant from The Hong Kong Polytechnic University.
References
- Cavalli F, Hansen HH, Kaye SB. (2004). Textbook of Medical Oncology. London, UK: Taylor & Francis Group.
- Chan SL, Yeung JH. (2006). Effects of polysaccharide peptide (PSP) from Coriolus versicolor on the pharmacokinetics of cyclophosphamide in the rat and cytotoxicity in HepG2 cells. Food Chem Toxicol, 44, 689–694.
- Committee to Revise the Guide, Institute of Laboratory Animal Resources Council, Commission on Life Sciences, National Research Council. (1996). Guide for the Care and Use of Laboratory Animals. 7th edn. Washington, DC: National Academy Press..
- Ooi VE, Liu F. (2000). Immunomodulation and anti-cancer activity of polysaccharide-protein complexes. Curr Med Chem, 7, 715–729.
- Cui FJ, Li Y, Xu YY, Liu ZQ, Huang DM, Zhang ZC, Tao WY. (2007a). Induction of apoptosis in SGC-7901 cells by polysaccharide-peptide GFPS1b from the cultured mycelia of Grifola frondosa GF9801. Toxicol in vitro, 21, 417–427.
- Cui FJ, Li Y, Xu ZH, Xu HY, Sun K, Tao WY. (2006). Optimization of the medium composition for production of mycelial biomass and exo-polymer by Grifola frondosa GF9801 using response surface methodology. Bioresour Technol, 97, 1209–1216.
- Cui FJ, Tao WY, Xu ZH, Guo WJ, Xu HY, Ao ZH, Jin J, Wei YQ. (2007b). Structural analysis of anti-tumor heteropolysaccharide GFPS1b from the cultured mycelia of Grifola frondosa GF9801. Bioresour Technol, 98, 395–401.
- Fan G, Filipczak L, Chow E. (2007). Symptom clusters in cancer patients: A review of the literature. Curr Oncol, 14, 173–179.
- Gordon JS, Curtin S. (2000). Comprehensive cancer care: Integrating alternative, complementary, and conventional therapies. J Natl Cancer Inst, 92, 1945–1946.
- Gorter JA, Aronica E, Hack NJ, Balázs R, Wadman WJ. (1995). Development of voltage-activated potassium currents in cultured cerebellar granule neurons under different growth conditions. J Neurophysiol, 74, 298–306.
- Gu CQ, Li J, Li JW, Chao FH. (2006). Inhibition of hepatitis B virus by D-fraction from Grifola frondosa: Synergistic effect of combination with interferon-alpha in HepG2 2.2.15. Antiviral Res, 72, 162–165.
- Hattori TS, Komatsu N, Shichijo S, Itoh K. (2004). Protein-bound polysaccharide K induced apoptosis of the human Burkitt lymphoma cell line, Namalwa. Biomed Pharmacother, 58, 226–230.
- Inoue K, Kawamoto K, Nakajima H, Kohno M, Kadoya S, Mizuno D. (1983). Chemical modification and antitumor activity of a D-manno-D-glucan from Microellobosporia grisea. Carbohydr Res, 115, 199–208.
- Jiménez-Medina E, Berruguilla E, Romero I, Algarra I, Collado A, Garrido F, Garcia-Lora A. (2008). The immunomodulator PSK induces in vitro cytotoxic activity in tumour cell lines via arrest of cell cycle and induction of apoptosis. BMC Cancer, 8, 78.
- Kwilosz D, Koroukian S, O’Toole E, Smyth K, Townsend A, VonGruenigen V, Rose J. (2007). Psychosocial registry for persons with cancer: A method of facilitating quality of life and symptom research. Psychooncology, 16, 358–364.
- Lavi I, Friesem D, Geresh S, Hadar Y, Schwartz B. (2006). An aqueous polysaccharide extract from the edible mushroom Pleurotus ostreatus induces anti-proliferative and pro-apoptotic effects on HT-29 colon cancer cells. Cancer Lett, 244, 61–70.
- Lee BC, Bae JT, Pyo HB, Choe TB, Kim SW, Hwang HJ, Yun JW. (2003). Biological activities of the polysaccharides produced from submerged culture of the edible basidiomycete Grifola frondosa. Enzyme Microb Tech, 32, 574–581.
- Lee JC, Lee KY, Son YO, Choi KC, Kim J, Truong TT, Jang YS. (2005). Plant-originated glycoprotein, G-120, inhibits the growth of MCF-7 cells and induces their apoptosis. Food Chem Toxicol, 43, 961–968.
- Li G, Kim DH, Kim TD, Park BJ, Park HD, Park JI, Na MK, Kim HC, Hong ND, Lim K, Hwang BD, Yoon WH. (2004). Protein-bound polysaccharide from Phellinus linteus induces G2/M phase arrest and apoptosis in SW480 human colon cancer cells. Cancer Lett, 216, 175–181.
- Li WY, Chan SW, Guo DJ, Chung MK, Leung TY, Yu PH. (2009). Water extract of Rheum officinale Baill. induces apoptosis in human lung adenocarcinoma A549 and human breast cancer MCF-7 cell lines. J Ethnopharmacol, 124, 251–256.
- Liu JX, Zhou JY, Liu TF. (1999). Phase III clinical trial for the Yun Zhi polysaccharide (PSP) capsules. In Advanced Research in PSP 1999, ed. Yang QY, pp. 295–303. Hong Kong: The Hong Kong Association for Health Care Limited.
- Mayell M. (2001). Maitake extracts and their therapeutic potential. Altern Med Rev, 6, 48–60.
- Mei YA, Wu MM, Huan CL, Sun JT, Zhou HQ, Zhang ZH. (2000). 4-aminopyridine, a specific blocker of K+ channels, inhibited inward Na+ current in rat cerebellar granule cells. Brain Res, 873, 46–53.
- Nanba H. (1995). Activity of maitake D-fraction to inhibit carcinogenesis and metastasis. Ann N Y Acad Sci, 768, 243–245.
- Newton HB. (2002). Chemotherapy for the treatment of metastatic brain tumors. Expert Rev Anticancer Ther, 2, 495–506.
- Ohno N, Iino K, Takeyama T, Suzuki I, Sato K, Oikawa S, Miyazaki T, Yadomae T. (1985). Structural characterization and antitumor activity of the extracts from matted mycelium of cultured Grifola frondosa. Chem Pharm Bull, 33, 3395–3401.
- Qian ZM, Xu MF, Tang PL. (1997). Polysaccharide peptide (PSP) restores immunosuppression induced by cyclophosphamide in rats. Am J Chin Med, 25, 27–35.
- Schuette W. (2001). Chemotherapy as treatment of primary and recurrent small cell lung cancer. Lung Cancer, 33 Suppl 1, S99–107.
- Stamets P. (2000). Growing Gourmet and Medicinal Mushrooms. Berkeley, California: Ten Speed Press.
- Suzuki I, Itani T, Ohno N, Oikawa S, Sato K, Miyazaki T, Yadomae T. (1984). Antitumor activity of a polysaccharide fraction extracted from cultured fruiting bodies of Grifola frondosa. J Pharmacobio-Dyn, 7, 492–500.
- Tewarson SL, Mittal VP, Singh M, Gupta GP. (1993). Serum sialic acid–an important cancer marker. Indian J Cancer, 30, 125–131.
- Tseng SH, Hwang LH, Lin SM. (1997). Induction of antitumor immunity by intracerebrally implanted rat C6 glioma cells genetically engineered to secrete cytokines. J Immunother, 20, 334–342.
- Yang X, Sit WH, Chan DK, Wan JM. (2005). The cell death process of the anticancer agent polysaccharide-peptide (PSP) in human promyelocytic leukemic HL-60 cells. Oncol Rep, 13, 1201–1210.
- Zhang M, Cui SW, Cheung PCK, Wang Q. (2007). Antitumor polysaccharides from mushrooms: A review on their isolation process, structural characteristics and antitumor activity. Trends Food Sci Tech, 18, 4–19.