Abstract
Objective: The pathogenesis of diabetic cardiomyopathy (DCM) is complex, and the therapeutic options available to treat DCM are limited. The present study was designed to investigate the effect of Aegle marmelos (L.) Correa (Rutaceae) leaf extract on early stage DCM in alloxan-induced diabetic rats.
Methods: Diabetes was induced in Wistar rats (150–200 g) by injecting alloxan (150 mg kg−1; i.p.). Ethanol extract of A. marmelos leaves was administered at varying doses (100, 200, and 400 mg kg−1) and tolbutamide (100 mg kg−1) as standard. Fasting blood glucose (FBG), total cholesterol, thiobarbituric acid reactive substances (TBARS), reduced glutathione (GSH), catalase (CAT), superoxide dismutase (SOD), lactate dehydrogenase (LDH) and creatine kinase (CK) were determined by standard methods.
Results: A. marmelos extract (AME) was found to decrease the levels of FBG, total cholesterol, TBARS, LDH and CK, and increase the levels of GSH, CAT and SOD dose dependently as compared to diabetic control groups. The maximum dose-dependent decrease in TBARS (63.46%), LDH (34.04%), CK (53.14%), and increase in GSH (64.91%), CAT (59.34%), SOD (69.65%) was evident at an optimum dose of 200 mg kg−1. Histopathological studies revealed salvage in the morphological derangements as indicated by absence of necrosis and marked decrease in inflammatory cells in AME-treated groups as compared to diabetic control.
Conclusions: The present investigations conclude that treatment with AME attenuates the severity and improves the myocardium in the early stages of alloxan-induced DCM at a dose of 200 mg kg−1.
Introduction
Diabetic cardiomyopathy (DCM) is one of the leading causes of morbidity and mortality in the diabetic patients worldwide. DCM is characterized by diastolic dysfunction, accumulation of extracellular matrix, activation of the inflammatory mechanisms, apoptosis and alterations in calcium homeostasis in the cardiomyocytes (CitationCai et al., 2006). The exact pathogenesis of DCM is complex and multifactorial. However, early factors leading to diabetic cardiac cell damage are documented to result from various stimuli such as hyperglycemia, glucose oxidation to reactive oxygen species (ROS), reactive nitrogen species, up-regulation of extracellular matrix proteins, myocardial fibrosis, activation of protein kinase C, cytokines and renin angiotensin system (CitationAsbun & Villarreal, 2006).
Studies have demonstrated that excess of glucose coupled with alterations in collagen structure leads to the formation of advanced glycation end (AGE) products that result in oxidative stress in the tissues (CitationAsbun & Villarreal, 2006). Excessive generation of ROS such as superoxide can result in cardiovascular complications (CitationHaidara et al., 2006). The heart has low levels of free radical scavenging mechanisms such as metalloproteinases and is therefore predisposed to oxidative damage in diabetes (CitationBabu et al., 2006). The increasing prevalence of the complications of diabetes and limited therapeutic options available has led to a renewed interest in medicinal plants effective in the treatment of diabetic syndrome. CitationBabu et al. (2006) have reported that green tea attenuates cardiac dysfunction in DCM. Investigations have revealed improved enzyme homeostasis and end organ function with Citrullus colocynthis (L.) Schrad (CitationDallak et al., 2009) and protective effect of Embelica ribes Burm (Myrsinaceae) fruit extract in isoproterenol-induced cardiotoxicity in diabetic rats (CitationBhandari & Ansari, 2009).
Aegle marmelos (L.) Correa (Rutaceae) is a medium sized, armed deciduous tree found throughout the Indian peninsula, Bangladesh, Ceylon, Thailand and Indo-China. All parts of the plant including the leaves, stem bark, fruit and seeds are used for a variety of ailments such as intermittent fever, hypo-chondriasis, palpitation, melancholia, diarrhea etc. (CitationMazumder et al., 2006; CitationMaity et al., 2009). The plant including the leaves, stem bark, fruit and seeds are documented to possess hypoglycaemic activity (CitationKar et al., 2003; CitationKesari et al., 2006). However, there is no documentation for the effect of A. marmelos on DCM. Therefore, the current study is aimed at investigating the effect of A. marmelos on early stage of cardiomyopathy in alloxan-induced diabetic rats.
Materials and methods
Plant material
The leaves of A. marmelos were collected in March–April from the botanical garden of Guru Nanak Dev University, Amritsar, India. The plant species was identified and authenticated by Dr. A.S. Soodan, Department of Botanical and Environmental Sciences, Guru Nanak Dev University, Amritsar, where the voucher specimen (SR./Bot.Sci./0350) was deposited.
Preparation of A. marmelos leaf extract
A. marmelos leaves (500 g) were cleaned, shade dried, and crushed in a grinder to give a coarse powder. The ethanol extract was prepared using Soxhlet apparatus. The solvent was evaporated under reduced pressure in rotatory evaporator (Laborota 4001, Heidolph) to give a yield of 9.6% w/w. The extract was stored in air tight bottle at −10°C in incubator (Thermotech TIC-4000, Metrex Scientific, New Delhi, India). Freshly prepared suspension of extract in 0.5% sodium carboxy methyl cellulose (CMC) solution before administration was used for the study.
Experimental design
Wistar rats (150–200 g) of either sex were used in the present study. The animals were kept on straw bedding in cages under natural light and dark cycle in central animal house facility of Guru Nanak Dev University and were acclimatized to the laboratory conditions (ambient temperature) for 7 days before the start of the experiments. Animals were fed with standard rodent diet and water ad libitum. The experimental protocol was duly approved by institutional ethics committee and care of animals was carried out as per the guidelines of Committee for the Purpose of Control and Supervision of Experiments on Animals, India (CitationCPCSEA, 2003).
Drugs and chemicals
Alloxan was procured from Sigma, St. Louis, Germany. Glucose estimation, creatine kinase (CK) and lactate dehydrogenase (LDH) kits were procured from Span Diagnostics, Surat, India. All other chemicals were of AR grade.
Experimental protocols
Wistar rats (150–200 g) were divided into seven groups, eight animals in each group. Group I: Nondiabetic group—the animals received the vehicle 0.5% CMC daily for a period of 14 days. In the remaining groups, diabetes was induced by a single intraperitoneal injection of freshly prepared alloxan at a dose of 150 mg kg−1. The fasting blood glucose (FBS) levels were estimated on 3rd day to confirm hyperglycemia. All the treatments were started from 3rd day. Group II: alloxan-induced diabetic control group. Group III: alloxan-treated diabetic vehicle group. The animals received a daily dose (1 mg kg−1) of vehicle (0.5% sodium CMC solution). Group IV treated with tolbutamide at a dose of 100 mg kg−1. Group V, VI and VII—alloxan diabetic rats treated with A. marmelos extract (AME) 100, 200 and 400 mg kg−1, respectively, from 3rd day onwards up to 14 days. Blood was collected by sinocular puncture under light anesthesia for estimation of LDH and CK. Rats were sacrificed by cervical dislocation, heart was dissected and perfused with ice cold isotonic saline and weighed. Heart homogenate was used for estimation of thiobarbituric acid reactive substances (TBARS), reduced glutathione (GSH), superoxide dismutase (SOD), catalase (CAT) and total protein. The hearts were stored in 10% formalin for histological studies.
Biochemical assays
The hearts were thawed and homogenized in 10% w/v ice cold 50 mM potassium phosphate buffer (pH 7.4). The homogenate was further centrifuged at 2500 rpm for 20 min at 4°C.
Estimation of total protein concentration
Total protein was estimated in cardiac homogenate by biuret method using total protein kit (Crest Biosystems, Goa, India).
Estimation of lipid peroxidation
TBARS, an index of lipid peroxidation was estimated according to the method of CitationOhkawa et al. (1979). The results were expressed as nmoles of malondialdehyde per mg protein.
Estimation of GSH
GSH in the heart was estimated by the method of CitationEllman (1959). The results were expressed as nmoles of GSH per mg protein.
Estimation of CAT activity
CAT activity was estimated using method of CitationAebi (1974). The CAT activity was expressed as moles of H2O2 decomposed per minute per mg protein.
Estimation of SOD
The SOD activity was estimated using the method described by CitationMisra and Fridovich (1972).
Estimation of LDH
LDH was estimated in serum according to 2, 4-DNPH method as described by CitationKing (1959).
Estimation of CK
CK-MB was measured in serum according to method of CitationHughes (1962).
Histological studies
For histological studies 4 μm sections were prepared with microtome and stained with haematoxylin for 15 min followed by counter stain with eosin for 2 min. The aberrations along with marked changes were studied through photomicrographs taken with digital camera (Olympus E-520) attached to pathological microscope (Magnus MLXi) using computer software.
Statistical analysis
Values for the enzymatic data were expressed as mean ± standard error of mean (SEM). One way ANOVA followed by post hoc analysis using Tukey’s test was used for statistical evaluation between different groups and values with p < 0.05 were considered to be significant. All statistical analyses were carried out using Instat software version 3.05 (GraphPad Software Inc., San Diego, CA).
Results
Characterization of the extract
Chromatographic analyses of the standard and extracts were performed on pre-coated silica gel aluminum-based plates (20 × 20 cm, Merck, Germany). The HPTLC system comprised of LINOMAT-IV applicator and CAMAG TLC SCANNER-III with CATS 4 software. Aliquots of standard and extracts were applied on TLC plates using 2 μl CAMAG capillaries. TLC plates were developed using hexane-chloroform-methanol (5:4:1) as the mobile phase. The development length was 50 mm. The plates were dried, sprayed with 0.5% anisaldehyde in sulfuric acid and scanned at 560 nm. The chromatograph revealed three prominent peaks at Rf 0.14, 0.52, 0.73 and four minor peaks at Rf 0.28, 0.35, 0.44 ().
Effect of various interventions on the biochemical parameters and heart rate in diabetic rats
Alloxan treatment was found to increase the FBS significantly (p < 0.05) in the diabetic control (54.1%) and diabetic vehicle (54.8%) treated groups as compared to the nondiabetic rats. The treatment with tolbutamide and AME was found to decrease the FBG and total cholesterol significantly (p < 0.05) in a dose-dependent manner as compared to diabetic control group (). The treatment with tolbutamide and different doses (100, 200, and 400 mg kg−1) of the AME was found to produce significant (p < 0.05) dose-dependent decrease in the levels of TBARS, CK and LDH while significant (p < 0.05) dose-dependent increase was observed in the levels of GSH, CAT and SOD as compared to the diabetic control rats (). The results indicated that maximum dose-dependent percentage decrease in TBARS (49.71–63.46%), CK (25.21–53.14%) and LDH (22.29–34.04%) and maximum dose-dependent percentage increase was observed in the levels of GSH (52.45–64.91%), CAT (57.47–59.34%) and SOD (59.39–69.65%) between a dose of 100 and 200 mg kg−1, respectively, of the AME. In order to compare the enhancers and scavengers of oxidant stress on the same scale, normalized values have been calculated by the formula given below and graph plotted for markers of myocardial injury and myocardial salvage () on same scale.
Table 1. Effect of various pharmacological interventions on the biochemical parameters in the diabetic rats.
Histopathological studies
Histopathological examination of the cardiac tissue of normal control group revealed normal architecture with well-preserved cytoplasm and absence of inflammatory cells and necrosis ( and ). The diabetic control heart showed focal area of necrosis () and focal collection of chronic inflammatory cells consisting of lymphocytes, plasma cells, macrophages along with normal looking myocardium (). In AGE-treated heart cardiomyocytes with focally minimal inflammatory infiltrate were observed with no evidence of necrosis. However, congestion was also evident ( and ). In the tolbutamide-treated heart, the inflammatory infiltrate and congestion are more highlighted as compared to the AGE-treated heart but less than the diabetic control heart ( and ).
Figure 4. Photomicrograph of heart showing normal architecture with well-preserved cytoplasm and absence of inflammatory cells and edema at 10× (A) and 40× (B); diabetic control heart shows marked infiltration of inflammatory cells, edema and necrosis (light stained portion) as marked by the arrows at 10× (C) and 40× (D); AGE-treated heart the inflammatory cells are markedly decreased and partial recovery of the hearts is observed as marked by the arrows at 10× (F) and 40× (G); tolbutamide-treated heart shows decreased inflammatory cells and congestion as marked by the arrows at 10× (G) and 40× (H).
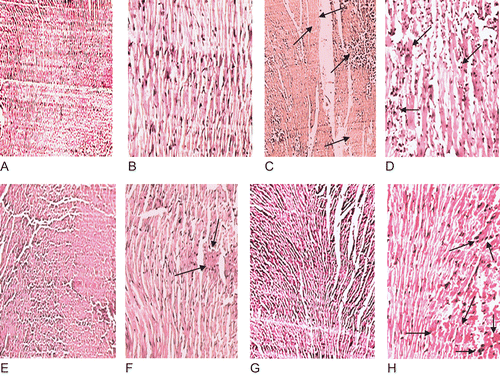
Discussion and conclusions
The present study was designed to investigate the ameliorative effect of AME on the early stages of DCM in alloxan diabetic rats. Alloxan is well documented to induce persistent hyperglycemia by the generation of ROS (CitationDuzguner & Kaya, 2007). These free radical species result in oxidative damage to the heart, kidneys, eyes, nerves, liver, small and large vessels and gastrointestinal system (CitationMishra et al., 2010). Inflammatory changes, hypertrophic remodeling and cell death have been reported to occur in the diabetic hearts in experimental animals as early as 7 days after the induction of the diabetic state (CitationCai et al., 2006). Administration of AME was found to decrease the FBG and total cholesterol significantly as compared to the untreated diabetic animals. AME is postulated to increase the uptake of glucose by increasing the insulin release and inducing the synthesis of insulin (CitationGireesh et al., 2008). The plant is also documented to help in regeneration of the damaged pancreas in diabetic rats and decrease the blood cholesterol levels (CitationDas et al., 1996). Hyperglycemia leads to oxidation of glucose superoxide anion radical generation and lipid peroxidation. Changes in the levels of TBARS, GSH, CAT and SOD have been used to quantify the oxidative damage in the hearts. The treatment with AME was found to decrease the TBARS significantly, whereas the levels of myocardial salvaging mechanisms i.e. GSH, CAT and SOD were increased significantly as compared to the diabetic control rats. SOD and CAT form enzymatic antioxidant defense system that removes the toxic free radicals. SOD brings about the conversion of highly toxic superoxide anion to hydrogen peroxide which is further detoxified by CAT. SOD is documented to get inactivated in diabetes by glycation while the superoxide anion causes inactivation of CAT which is the vital determinant of cardiac antioxidant status (CitationSefi et al., 2010). The decrease in the levels of TBARS and increase in the levels of GSH, SOD, and CAT indicates antioxidant activity of the plant. Therefore, it may be proposed that the antioxidant activity of the plant is responsible for preventing the increase in deleterious markers and increase the protective mechanisms. CK and LDH are well established as the markers of myocardial damage (CitationZhang et al., 2006). Increase in serum CK and LDH due to massive release of these enzymes from the heart has been reported in diabetic rats (CitationDallak et al., 2008). Similar findings were observed in the present study. Treatment with AME was found to decrease the CK and LDH levels significantly (p < 0.05) as compared to the untreated diabetic control rats. The leaf extracts of the plant contain auraptene that has been postulated to decrease calcium overload and myocardial damage in isolated cardiomyocytes (CitationKakiuchi et al., 1991).
Histopathological changes in the diabetic rat hearts showed reduction in cardiac mass, fibrosis, loss of myocardial tissue, hypertrophy and infiltration of inflammatory mediators (CitationCai & Kang, 2003). Similar changes have been observed in the current study. AME was found to decrease the inflammatory cells and restore cardiomyocyte structure in the diabetic rat hearts. Also, A. marmelos has been documented to have a weak PPAR-γ activating property (CitationHuang et al., 2009). PPAR-γ plays a significant role in regulating glucose and lipid metabolism and inflammatory responses in various tissues (CitationKapadia et al., 2008). The therapeutic options available to treat DCM are limited and require simultaneous administration of drugs acting on multiple target sites, due to its complex pathogenesis. The present study shows that AME is effective in attenuating early DCM in rats. This protection may be a result of simultaneous action on the various target sites such as insulin promoting, free radical scavenging, modulation of intracellular calcium, PPAR-γ activation or receptor mediated process. It may provide a useful therapeutic option in the treatment of DCM.
Acknowledgments
The authors are grateful to Dr. Amarjit Singh, Additional Professor, Department of Pathology, Govt. Medical College, Amritsar, India, for guidance in histological studies and Prof. Avinash Nagpal, Department of Botanical and Environmental Sciences, Guru Nanak Dev University, Amritsar, India, for providing facilities for photomicrographs.
Declaration of interest
The authors report no conflicts of interest. The authors alone are responsible for the content and writing of the paper.
References
- Aebi H. (1974). Catalase. Methods of Enzymatic Analysis. 2nd edition, New York: Academic Press, pp. 673–685.
- Asbun J, Villarreal FJ. (2006). The pathogenesis of myocardial fibrosis in the setting of diabetic cardiomyopathy. J Am Coll Cardiol, 47, 693–700.
- Babu PV, Sabitha KE, Shyamaladevi CS. (2006). Therapeutic effect of green tea extract on oxidative stress in aorta and heart of streptozotocin diabetic rats. Chem Biol Interact, 162, 114–120.
- Bhandari U, Ansari MN. (2009). Ameliorative effect of an ethanol extract of Embelica ribes fruits on isoproterenol induced cardiotoxicity in diabetic rats. Pharm Biol, 47, 669–674.
- Cai L, Kang YJ. (2003). Cell death and diabetic cardiomyopathy. Cardiovasc Toxicol, 3, 219–228.
- Cai L, Wang Y, Zhou G, Chen T, Song Y, Li X, Kang YJ. (2006). Attenuation by metallothionein of early cardiac cell death via suppression of mitochondrial oxidative stress results in a prevention of diabetic cardiomyopathy. j Am Coll Cardiol, 48, 1688–1697.
- CPCSEA. (2003). CPCSEA guidelines for laboratory animal facility. Indian J Pharmacol, 35, 257–274.
- Dallak M, Bashir N, Abbas M, Elassa R, Haidara M, Khalil M, Al-Khateeb MA. (2009). Concomitant down regulation of glycolytic enzymes, upregulation of gluconeogenic enzymes and potential hepato-nephroprotective effects following the chronic administration of hypoglycaemic, insulinotropic Citrullus colocynthis pulp extract. Am J Biochem and Biotech, 5, 153–161.
- Dallak MM, Mikhailidis DP, Haidara MA, Bin-Jaliah IM, Tork OM, Rateb MA, Yassin HZ, Al-Refaie ZA, Ibrahim IM, Elawa SM, Rashed LA, Afifi NA. (2008). Oxidative stress as a common mediator for apoptosis induced-cardiac damage in diabetic rats. Open Cardiovasc Med j, 2, 70–78.
- Das AV, Padayatti PS, Paulose CS. (1996). Effect of leaf extract of Aegle marmelose (L.) Correa ex Roxb. on histological and ultrastructural changes in tissues of streptozotocin induced diabetic rats. Indian j Exp Biol, 34, 341–345.
- Duzguner V, Kaya S. (2007). Effect of zinc on the lipid peroxidation and the antioxidant defense systems of the alloxan-induced diabetic rabbits. Free Radic Biol Med, 42, 1481–1486.
- Ellman GL. (1959). Tissue sulfhydryl groups. Arch Biochem Biophys, 82, 70–77.
- Gireesh G, Reas SK, Jobin M, Paulose CS. (2008). Decreased muscarinic M1 receptor gene expression in the cerebral cortex of streptozotocin-induced diabetic rats and Aegle marmelose leaf extract’s therapeutic function. j Ethnopharmacol, 116, 296–304.
- Haidara MA, Yassin HZ, Rateb M, Ammar H, Zorkani MA. (2006). Role of oxidative stress in development of cardiovascular complications in diabetes mellitus. Curr Vasc Pharmacol, 4, 215–227.
- Huang TH, Teoh AW, Lin BL, Lin DS, Roufogalis B. (2009). The role of herbal PPAR modulators in the treatment of cardiometabolic syndrome. Pharmacol Res, 60, 195–206.
- Hughes BP. (1962). A method for the estimation of serum creatine kinase and its use in comparing creatine kinase and aldolase activity in normal and pathological sera. Clin Chim Acta, 7, 597–603.
- Kakiuchi N, Senaratne LR, Huang SL, Yang XW, Hattori M, Pilapitiya U, Namba T. (1991). Effects of constituents of Beli (Aegle marmelos) on spontaneous beating and calcium-paradox of myocardial cells. Planta Med, 57, 43–46.
- Kapadia R, Yi JH, Vemuganti R. (2008). Mechanisms of anti-inflammatory and neuroprotective actions of PPAR-γ agonists. Front Biosci, 13, 1813–1826.
- Kar A, Choudhary BK, Bandyopadhyay NG. (2003). Comparative evaluation of hypoglycaemic activity of some Indian medicinal plants in alloxan diabetic rats. j Ethnopharmacol, 84, 105–108.
- Kesari AN, Gupta RK, Singh SK, Diwakar S, Watal G. (2006). Hypoglycemic and antihyperglycemic activity of Aegle marmelos seed extract in normal and diabetic rats. j Ethnopharmacol, 107, 374–379.
- King J. (1959). A routine method for the estimation of lactic dehydrogenase activity. j Med Lab Technol, 16, 265–272.
- Maity P, Hansda D, Bandyopadhyay U, Mishra DK. (2009). Biological activities of crude extracts and chemical constituents of Bael, Aegle marmelos (L.) Corr. Indian j Exp Biol, 47, 849–861.
- Mazumder R, Bhattacharya S, Mazumder A, Pattnaik AK, Tiwary PM, Chaudhary S. (2006). Antidiarrhoeal evaluation of Aegle marmelos (Correa) Linn. root extract. Phytother Res, 20, 82–84.
- Mishra A, Bhatti R, Singh A, Singh Ishar MP. (2010). Ameliorative effect of the cinnamon oil from Cinnamomum zeylanicum upon early stage diabetic nephropathy. Planta Med, 76, 412–417.
- Misra HP, Fridovich I. (1972). The role of superoxide anion in the autoxidation of epinephrine and a simple assay for superoxide dismutase. J Biol Chem, 247, 3170–3175.
- Ohkawa H, Ohishi N, Yagi K. (1979). Assay for lipid peroxides in animal tissues by thiobarbituric acid reaction. Anal Biochem, 95, 351–358.
- Sefi M, Fetoui H, Makni M, Zeghal N. (2010). Mitigating effects of antioxidant properties of Artemisia campestris leaf extract on hyperlipidemia, advanced glycation end products and oxidative stress in alloxan-induced diabetic rats. Food Chem Toxicol, 48, 1986–1993.
- Zhang CH, Zhang WJ, Xu J, Yu XJ, Lv J, Jing AY, Chen LN, Hu H, Sun Q. (2006). A method to produce animal model of diabetic cardiomyopathy. Wei Sheng Yan Jiu, 35, 707–711.