Abstract
Context: Carthamus tinctorius injection (CTI) is a traditional Chinese medicine (TCM) specifically used for the treatment of cerebral ischemia and myocardial ischemia.
Objective: This study evaluated the protective effects of CTI on isoprenaline-induced acute myocardial ischemia (AMI) in rats and explored the underlying mechanisms.
Materials and methods: (i) Sprague-Dawley rats were randomly divided into 5 groups: control, myocardial ischemia model, and high-, low-dose of CTI groups (2.5 and 0.625 g/kg, respectively, i.p. for 5 days), and Xiang-Dan (20 g/kg) group (n = 10 in each group). AMI was induced by isoproterenol (5 mg/kg) by intraperitoneal injection. Assessment of electrocardiograms (ECG) was carried out. (ii) Another 40 rats were randomly divided into 5 groups, the concentration of IL-6 and TNF-α in serum were measured by radioimmunological assay; Bcl-2 and Bax protein expression were measured by immunohistochemistry.
Results: CTI (2.5 and 0.625 g/kg) significantly inhibited the typical ECG S-T segment elevation, reduced concentration of IL-6 and TNF-α in serum, suppressed overexpression of Bax protein and also inhibited the reduction of Bcl-2 expression and markedly depressed the Bax/Bcl-2 ratio.
Discussion and conclusion: These findings demonstrate that CTI is cardioprotective against AMI in rats and is likely to related to decrease inflammatory response mediated by TNF-α and IL-6, down-regulate protein level of Bax and up-regulate that of Bcl-2 in the heart tissue.
Introduction
Acute myocardial infarction (AMI) is a major contributor to ischemic heart disease (IHD) (CitationKloner, 2006), a leading cause of mortality in the modern world (CitationVenardos et al., 2007). Accumulating evidence has recently documented that apoptosis (CitationKang & Izumo, 2000; CitationAnselmi et al., 2004) and the inflammatory response (CitationRossen et al., 1985) play a critical role in AMI. Several results suggest the process of apoptosis occurred after the expression of proinflammatory cytokines in AMI myocardium (CitationZhang et al., 2006).
In recent years, folk medicines and their extracts have shown cardioprotective effects in AMI (CitationZhou et al., 2000; CitationLing & Lou, 2005). Carthamus tinctorius injection (CTI), the aqueous extract of C. tinctorius, is a traditional Chinese medicine (TCM) widely used in the treatment of myocardial ischemia in clinical practice. The main component of CTI is hydroxysafflor yellow A (), which has been demonstrated anti-myocardial ischemia effects (CitationHan et al., 2009; CitationZeng et al., 2009), but little is known about the mechanism underlying the cardioprotective effects of CTI.
The aims of the present investigation were as follows: (i) to evaluate the effect of CTI on acute myocardial ischemia (AMI) in rat model, and (ii) to make clear whether the cardioprotective effect is related to proinflammatory cytokines and myocardial apoptosis.
Materials and methods
Drug and animals
The CTI (CTI: No. 010801) was manufactured by Sanjiu Medical and Pharmaceutical Co. (Yaan, China). The Xiang-Dan injection (XD: No. Z42020392) was manufactured by Qingda Kangdi Pharmaceutical Co. (Shiyan, China).
Sprague-Dawley rats (♂ ♀, n = 90) weighing 180–220 g were purchased from the Experimental Animal Center of Sichuan University (Chengdu, China). Animals were allowed free access to standard diet and sterile water in a restricted access room with temperature-controlled (20 ± 1°C) and humidity-controlled (60 ± 10%) rooms under 12 h light/dark. All the experimental procedures were performed in accordance with the guidelines of the Experimental Research Institute of Sichuan University.
AMI model (Experiment 1)
Fifty rats were randomly divided into 5 groups: sham, AMI model, CTIL, CTIH and Xiang-Dan injection (XD) group (n = 10). The rats in the CTIL and CTIH were administered the appropriate doses (0.625 g/kg, equal to 5-fold the clinic dosage; 2.5 g/kg; equal to 20-fold the clinic dosage, respectively) of CTI each day for 5 d by intraperitoneal injection (i.p.). The rats in the XD were administered the appropriate doses of XD (20 g/kg; equal to 20-fold the clinic dosage) each day for 5 d by intraperitoneal injection (i.p.). MI rats received an equal volume of distilled water as did control rats. At D3, 1 h after the treatment, rats from the MI, CTIL, CTIH and XD were administered isoproterenol (ISO, 5 mg/kg, i.p.). All animals were allowed to move and feed ad libitum.
Electrocardiogram recording
Rats were anesthetized intraperitoneally with 10% chloral hydrate dose of 300 mg/kg and subcutaneous peripheral limb electrodes were inserted. Electrocardiogram (ECG) was recorded at 24 h before AMI model established, 1 and 48 h after AMI model established, respectively. ECG waveforms were inspected visually for rhythm and configuration abnormalities. ECG parameters (S-T segment and T wave) were generated using the BL-410 experimental system (TME Technology Co, China).
AMI model (Experiment 2)
In the second part, 40 rats were randomly divided into 5 groups (n = 8) mentioned above (2.2.1.). One hour after the last treatment, all the rats were anesthetized intraperitoneally with 10% chloral hydrate dose of 300 mg/kg, blood samples were collected from the abdominal aorta and the serum was obtained through centrifugation at 3000×g for 10 min. At the same time, the tissues of the cardiac apex were fixed by transcardiac perfusion with 4% paraformaldehyde in phosphate buffer saline and post-fixation in the same solution for 24 h at 4°C for histopathological analysis and immunohistochemistry measurement.
Measurement of serum TNF-α and IL-6
Levels of TNF-α and IL-6 in serum were measured by radioimmunological assay and liquid phase competitive radioassay according to the manufacturer’s instruction (Boster, Wuhan, China).
Histopathological examination
The heart tissues obtained from all experimental groups were embedded in paraffin, sectioned at 4 μm and stained with HE. The sections were examined under light microscope (200×) and then photomicrographs were taken.
Measurement of myocardial Bcl-2 and Bax protein
Sections were cleared of paraffin, and endogenous peroxidases were blocked by incubation with 3% H2O2 and washed. Sections of the tissues were then incubated with rabbit serum for 10 min at ambient temperature. Subsequently, the sections were incubated overnight with a goat polyclonal anti-Bax antibody (Boster, China, 1:50) and anti-Bcl-2 antibody (Boster, China, 1:50) at 4°C, followed by the addition of biotinylated rabbit anti-goat IgG secondary antibody (Jinshan, BJ, China). To verify the binding specificity for Bax and Bcl-2, some sections were also incubated with primary antibody only (no secondary) or with secondary antibody only (no primary). In these situations, no positive staining was found in the sections, indicating that the immunoreactions were positive in all the experiments carried out. Immunohistochemistry staining was processed in accordance with the manufacturer’s instructions and visualized by the use of diaminobenzidine (DAB) staining. Counterstaining was carried out with Harris hematoxylin (Sigma). Five sections were analyzed for each animal and the four images were acquired from a randomly selected location in each slide. Digital photos were analyzed with Image-pro plus (Media Cybernetics, USA) by an observer blind to the treatment groups.
Statistical analysis
Values are presented as means ± standard deviation. Statistical significance was determined by one-way ANOVA and Student’s t-test using SPSS13.0 for Windows. P < 0.05 was considered to indicate statistical significance.
Results
Effect of CTI on ECG
ECG was measured 24 h before AMI model established. There was no difference of ECG for each group. However, the morphology of ECG demonstrated variability in AMI rats 1 h after the ISO administration and gradually stabilized 48 h after the ISO administration in all of the animals (). As shown in , S-T segment and T wave showed elevation in AMI rats at 1 and 48 h after the ISO administration (p < 0.05). At the same time, the typical ECG S-T segment and T wave elevation were significantly inhibited by CTIH and XD (p < 0.05) at 1 h and 48 h after establishing model of AMI( and ).
Figure 2. Effect of CTI on S-T segment deviation (mv) of acute myocardial ischemia (AMI) rats. (A) Representative chants of ECG. Differences in the ECG patterns were observed between the groups at 1 and 48 h after isoproterenol-induced AMI model in rats. As the arrows show, the S-T segment and T wave of rats were elevated significantly and were inhibited by CTI and XD. (a), (b), (c), (d), (e), (f), (g), (h) and (i) correspond to groups: (a) control group; (b) model group at 1 h after AMI model established; (c) model group at 48 h after AMI model established; (d) CTIH group at 1 h after AMI model established; (e) CTIH group at 48 h after AMI model established; (f) CTIL group at 1 h after AMI model established; (g) CTIL group at 48 h after AMI model established; (h) XD group at 1 h after AMI model established; (i) XD group at 48 h after AMI model established. The paper speed was 100 mm/s. (B) Effect of CTI on S-T segment deviation (mv) of acute myocardial ischemia (AMI) rats. Results are mean ± SD (n = 10). Compared with sham: *p < 0.05. Compared with AMI: #p < 0.05.
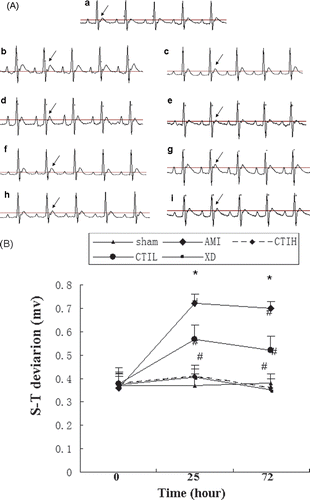
Inhibition effects of CTI on serum TNF-α and IL-6 level
Anti-inflammation activity of CTI was investigated by radioimmunological assay and liquid phase competitive radioassay. One hour after the last treatment, the levels of TNF-α and IL-6 in AMI were higher than that in the control (p < 0.05). Moreover, the serum levels of TNF-α and IL-6 in the CTI-treated groups decreased significantly in a dose-dependent manner as compared with the AMI (p < 0.05) ().
Protective effects of CTI on histopathological changes
The myocardial lesions of AMI were mainly localized in the endocardial and middle layers of the myocardium. ISO-induced AMI was characterized by multiple focal necroses with infiltration of inflammatory cells (). In the animals that received CTI and XD, the myocardial lesions were similar to the lesions in the control group: the size of patchy lesions in CTI and XD treated rat hearts were smaller, and the number of inflammatory cells was reduced as compared to those in AMI group.
Figure 4. Representative HE staining of the myocardium in rats (×400). (a), (b), (c), (d), and (e) correspond to groups: (a) control group showing normal cardiac fiber without inflammatory cells; (b) AMI group showing multiple focal necroses with infiltration of inflammatory cells; (c-e) AMI rats treated with CTI (0.625 and 2.5 g/kg) and XD (20 g/kg).
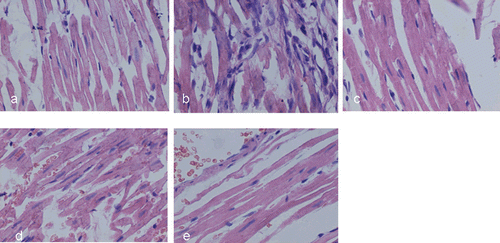
Effects of CTI on myocardial Bcl-2 and Bax protein level
To test whether the effect of CTI on the regulation of apoptosis gene, immunohistochemistry staining was performed for Bcl-2 and Bax protein. showed that the protein levels of these products changed in CTI-treated myocardial ischemia rat. One hour after the last treatment, the level of Bcl-2 and Bax in AMI were higher than that in the control (p < 0.05). As shown in and , CTI and XD treatment increased expression of the anti-apoptotic protein Bcl-2 compared with the AMI (p < 0.05). The expression of the pro-apoptotic protein Bax was attenuated significantly by CTI and XD treatment in a dose-dependent manner as compared with the AMI (p < 0.05). A marked depression of the Bax/Bcl-2 ratio was observed after CTI and XD treatment in (p < 0.05). There was no significant difference between these two kinds of CTI dosages (p > 0.05).
Figure 5. Expression of Bax and Bcl-2 proteins in myocardium. (A) Representative immunostaining for Bax and Bcl-2 proteins in myocardium in rats (×400). (a), (b), (c), (d), (e), (f), (g), (h), (i), and (j) correspond to groups: (a) control group for Bax; (b) AMI group for Bax; (c) CTIH group for Bax; (d) CTIL group for Bax; (e) XD group for Bax; (f) normal group for Bcl-2; (g) AMI group for Bcl-2; (h) CTIH group for Bcl-2; (i) CTIL group for Bcl-2; (j) XD group for Bcl-2; (B) Semi-quantitative analysis of the optical density of Bax; (C) Semi-quantitative analysis of the optical density of Bcl-2; (D) The calculated Bax/Bcl-2 ratio. Values are shown as mean ± SD (n = 8). Compared with sham: *p < 0.05. Compared with AMI: #p < 0.05.
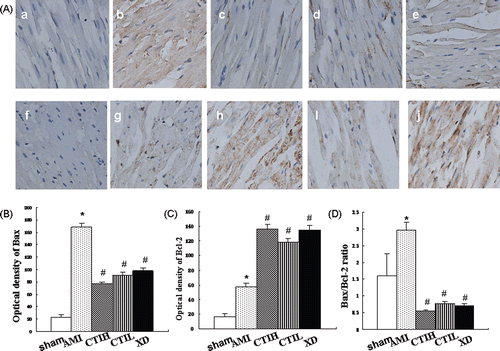
Discussion
The present study is the first to demonstrate that CTI (2.5 and 0.625 g/kg) had potent anti-myocardial ischemia effects in the ISO-induced AMI rat model, which led to attenuation of S-T segment elevation and improvement of myocardial lesions. The mechanism was that CTI reduced the concentration of IL-6 and TNF-α in serum, suppressed overexpression of Bax protein and also inhibited the reduction of Bcl-2 expression and markedly depressed the Bax/Bcl-2 ratio.
ECG is considered to be the best way to diagnose myocardial ischemia and evaluate the potential drugs against myocardial ischemia. ECG abnormalities such as ST-segment deviation could be due to the consecutive loss of cell membrane in injured myocardium (CitationSumitra et al., 2001). About 40% of all AMIs demonstrated S-T segment elevation (CitationHellermann et al., 2002). A large amount of evidence has demonstrated that C. tinctorius (safflower) or its components possess protective effects against myocardial or cerebral ischemia (CitationZheng et al., 2003; CitationWang et al., 2007). In our study, administration of CTI (2.5 and 0.625 g/kg, respectively, for 5 days) could markedly attenuate the increase of ST-segment elevation.
Some studies indicated that ISO-induced acute myocardial infarction after ischemia is caused by myocardial hyperactivity and coronary hypotension (CitationYeager & Iams, 1981), the inflammatory response (CitationSteffens et al., 2009) and cell apoptosis (CitationTanaka et al., 2004). To further examine the cardioprotective effect, the cardiac damage was determined using HE stain. Our study indicated that in the ISO-induced AMI group, there were multiple focal necroses with infiltration of inflammatory cells in the endocardial and middle layers of the myocardium. Both of two dosages of CTI relieved the multiple focal necroses degree and the infiltration of inflammatory cells of ischemic myocardium. Both of them suggest that CTI has definite cardioprotective effect and can ameliorate the supply of blood and oxygen for myocardium.
Inflammatory response and cytokine elaboration are integral components of the host immune response to tissue injury and play an active role after myocardial infarction (CitationNian et al., 2004). Upregulation of proinflammatory cytokines, such as TNF-α and IL-6, usually has been clearly shown both in animal and human models during AMI (CitationAkasaka et al., 2006). In our study, the serum levels of TNF-α and IL-6 in AMI were higher than that in the control. Administration of two dosages of CTI could significantly return the altered level of TNF-α and IL-6 in serum.
After the expression of proinflammatory cytokines, the process of myocyte apoptosis, induced by TNF-α (CitationKrown et al., 1996) via binding to TNFR-1 that contains a death domain (CitationScreaton & Xu, 2000), occurred in myocardium (CitationZhang et al., 2006). Bcl-2 and Bax are important components of the mitochondrial-mediated signal transduction pathway in the control of apoptosis. Bcl-2 can promote cell survival by inhibiting apoptosis, while Bax can promote apoptosis by forming Bcl-2/Bax heterodimers (CitationWang et al., 2002; CitationBuchholz et al., 2003). Therefore, the relative ratio of pro-apoptotic and anti-apoptotic members of the Bcl-2 family is believed to respond to various apoptotic stimuli (CitationYang & Korsmeyer, 1996). In addition, Bcl-2 gene also could beneficial blockade the development of myocardial ischemia by decreasing production of IL-6 and TNF-α (CitationTanaka et al., 2004). In order to explore whether apoptosis-related genes contribute to the cardioprotective effect of CTI on rats, we analyzed the relative Bcl-2 and Bax expression levels induced by CTI in this study. Our results have suggested that to accompany with the significant increases of serum IL-6 and TNF-α, the level of myocardial Bcl-2 and Bax proteins in AMI were significantly changed. Administration of CTI (2.5 and 0.625 g/kg, respectively, for 5 days) also suppressed overexpression of Bax protein and also inhibited the reduction of Bcl-2 expression and markedly depressed the Bax/Bcl-2 ratio. This result suggests that the cardioprotective effects of CTI are most prominently exerted via inhibition of TNF-α and IL-6 and induction of rat cardiac myocyte apoptosis.
In conclusion, we show that CTI exerts a substantial protective effect against ISO-induced acute myocardial infarction after ischemia and demonstrate that this occurs via preventing generation of IL-6 and TNF-α, as well as modulating expression of the Bcl-2 family in rat model. These findings provide a therapeutic potential for future treatment of cardiovascular diseases.
Acknowledgments
The authors gratefully acknowledge the encouragement of Pro. Li-Ming Zhou, Director, Department of Pharmacology, West China Centre of Medical Sciences, Sichuan University, Chengdu, China.
Declaration of interest
The authors report no conflicts of interest.
References
- Akasaka Y, Morimoto N, Ishikawa Y, Fujita K, Ito K, Kimura-Matsumoto M, Ishiguro S, Morita H, Kobayashi Y, Ishii T. (2006). Myocardial apoptosis associated with the expression of proinflammatory cytokines during the course of myocardial infarction. Mod Pathol, 19, 588–598.
- Anselmi A, Abbate A, Girola F, Nasso G, Biondi-Zoccai GG, Possati G, Gaudino M. (2004). Myocardial ischemia, stunning, inflammation, and apoptosis during cardiac surgery: A review of evidence. Eur J Cardiothorac Surg, 25, 304–311.
- Buchholz TA, Davis DW, McConkey DJ, Symmans WF, Valero V, Jhingran A, Tucker SL, Pusztai L, Cristofanilli M, Esteva FJ, Hortobagyi GN, Sahin AA. (2003). Chemotherapy-induced apoptosis and Bcl-2 levels correlate with breast cancer response to chemotherapy. Cancer J, 9, 33–41.
- Han SY, Li HX, Ma X, Zhang K, Ma ZZ, Tu PF. (2009). Protective effects of purified safflower extract on myocardial ischemia in vivo and in vitro. Phytomedicine, 16, 694–702.
- Hellermann JP, Reeder GS, Jacobsen SJ, Weston SA, Killian JM, Roger VL. (2002). Longitudinal trends in the severity of acute myocardial infarction: A population study in Olmsted County, Minnesota. Am j Epidemiol, 156, 246–253.
- Kang PM, Izumo S. (2000). Apoptosis and heart failure: A critical review of the literature. Circ Res, 86, 1107–1113.
- Kloner RA. (2006). Natural and unnatural triggers of myocardial infarction. Prog Cardiovasc Dis, 48, 285–300.
- Krown KA, Page MT, Nguyen C, Zechner D, Gutierrez V, Comstock KL, Glembotski CC, Quintana PJ, Sabbadini RA. (1996). Tumor necrosis factor alpha-induced apoptosis in cardiac myocytes. Involvement of the sphingolipid signaling cascade in cardiac cell death. J Clin Invest, 98, 2854–2865.
- Ling H, Lou Y. (2005). Total flavones from Elsholtzia blanda reduce infarct size during acute myocardial ischemia by inhibiting myocardial apoptosis in rats. J Ethnopharmacol, 101, 169–175.
- Nian M, Lee P, Khaper N, Liu P. (2004). Inflammatory cytokines and postmyocardial infarction remodeling. Circ Res, 94, 1543–1553.
- Rossen RD, Swain JL, Michael LH, Weakley S, Giannini E, Entman ML. (1985). Selective accumulation of the first component of complement and leukocytes in ischemic canine heart muscle. A possible initiator of an extra myocardial mechanism of ischemic injury. Circ Res, 57, 119–130.
- Screaton G, Xu XN. (2000). T cell life and death signalling via TNF-receptor family members. Curr Opin Immunol, 12, 316–322.
- Steffens S, Montecucco F, Mach F. (2009). The inflammatory response as a target to reduce myocardial ischaemia and reperfusion injury. Thromb Haemost, 102, 240–247.
- Sumitra M, Manikandan P, Kumar DA, Arutselvan N, Balakrishna K, Manohar BM, Puvanakrishnan R. (2001). Experimental myocardial necrosis in rats: role of arjunolic acid on platelet aggregation, coagulation and antioxidant status. Mol Cell Biochem, 224, 135–142.
- Tanaka M, Nakae S, Terry RD, Mokhtari GK, Gunawan F, Balsam LB, Kaneda H, Kofidis T, Tsao PS, Robbins RC. (2004). Cardiomyocyte-specific Bcl-2 overexpression attenuates ischemia-reperfusion injury, immune response during acute rejection, and graft coronary artery disease. Blood, 104, 3789–3796.
- Wang C, Zhang D, Li G, Liu J, Tian J, Fu F, Liu K. (2007). Neuroprotective effects of safflor yellow B on brain ischemic injury. Exp Brain Res, 177, 533–539.
- Wang QF, Chen JC, Hsieh SJ, Cheng CC, Hsu SL. (2002). Regulation of Bcl-2 family molecules and activation of caspase cascade involved in gypenosides-induced apoptosis in human hepatoma cells. Cancer Lett, 183, 169–178.
- Venardos KM, Perkins A, Headrick J, Kaye DM. (2007). Myocardial ischemia-reperfusion injury, antioxidant enzyme systems, and selenium: A review. Curr Med Chem, 14, 1539–1549.
- Yang E, Korsmeyer SJ. (1996). Molecular thanatopsis: A discourse on the BCL2 family and cell death. Blood, 88, 386–401.
- Yeager JC, Iams SG. (1981). The hemodynamics of isoproterenol-induced cardiac failure in the rat. Circ Shock, 8, 151–163.
- Zeng X, Yang J, Yang X, Hong D, Wu L, Yu J. (2009). Effect of Guanxin No.2 decoction on gene expression in different areas of the myocardial infarcted heart of rats using microarray technology. J Pharm Pharmacol, 61, 213–219.
- Zhang J, Liao Y, Cheng X, Chen J, Chen P, Gao X, Zhang Z. (2006). Myosin specific-T lymphocytes mediated myocardial inflammation in adoptive transferred rats. Cell Mol Immunol, 3, 445–451.
- Zheng WC, Chen DB, Li B, Zhang L. (2003). The preventive effects of safflor yellow on myocardium injury of mycardium ischemic reperfusion in rats. Chin Pharmacol Bull, 19, 1032–1034.
- Zhou JY, Fan Y, Kong JL, Wu DZ, Hu ZB. (2000). Effects of components isolated from Astragalus membranaceus Bunge on cardiac function injured by myocardial ischemia reperfusion in rats. Zhongguo Zhong Yao Za Zhi, 25, 300–302.