Abstract
Context: Natural products are reported to have substantial neuroprotective activity due to their radical scavenging capacity, and also acetylcholinesterase (AChE) inhibitory capacity, both activities important in neurodegeneration.
Objective: The undesirable side effects of compounds in pharmacological use make it important to identify natural neuroprotective molecules. This work assesses the potential of five endemic Portuguese plants as sources of neuroprotective compounds.
Materials and methods: Antioxidant capacity for peroxyl radical was determined by Oxygen Radical Absorbance Capacity method and for hydroxyl by Electron Paramagnetic Resonance, as well as AChE inhibitory capacity of the plant hydroethanolic extracts. The molecules responsible for these valuable properties were also tentatively identified by HPLC.
Results and discussion: Armeria rouyana and Thymus capitellatus presented some of the highest phenolic contents (76.60 ± 7.19 and 12.82 ± 0.24 mg GAE g−1 dw, respectively) and antioxidant capacities (592 ± 116 and 449 ± 57 μmol TE g−1 dw, respectively). The flavonoids were identified as the phytomolecules related to the antioxidant capacity of these plant extracts; in the case of A. rouyana, l-ascorbic acid also made an important contribution (3.27 ± 0.26 mg g−1 dw). Plant extracts clearly demonstrated effective AChE inhibitory activity (480 ± 98 and 490 ± 46 μg mL−1, respectively), that could be associated to polyphenols.
Conclusions: The extracts of A. rouyana and T. capitellatus and their active components, especially polyphenols, demonstrate interesting neuroprotective potential. They, therefore, deserve further study as their phytomolecules are promising sources of either natural neuroprotective products and/or novel lead compounds.
Introduction
Neurodegenerative diseases are multifactorial disorders in which many biological processes become unregulated. They share a substantial number of processes, including cell signaling, oxidative stress, inflammation, apoptosis, accumulation of aggregation-prone misfolded protein, among others (CitationJoyner & Cichewicz, 2011). A multitarget therapeutic strategy aiming at different pharmacological mechanisms might provide a more rational and improved dementia treatment approach. Natural products have made significant contributions towards the treatment of degenerative diseases (CitationNewman & Cragg, 2007). Secondary metabolites have long contributed to the development of small-molecule therapeutics, due, in part, to their combination of unique chemical features and potent bioactivities; plants are a rich source of such metabolites. Several small molecules have been reported to exhibit inhibitory properties in neurodegeneration (CitationMandel et al., 2006; CitationRamassamy, 2006; CitationWilliams et al., 2011).
Alterations produced by neuropathologies include oxidative stress markers (CitationAndersen, 2004) as well as the decline in cognitive function associated with cholinergic deficits (CitationBachurin, 2003). The limited resources for combating oxidative stress by the central nervous system include the following: Vitamins, bioactive molecules, lipoic acid, antioxidant enzymes and redox sensitive protein transcriptional factors. Furthermore, this defense system can be activated/modulated by natural products such as polyphenols (CitationMandel et al., 2008; CitationRamassamy, 2006).
Acetylcholinesterase (AChE) inhibition may help in the treatment of Alzheimer’s disease (AD) as well as senile dementia, myasthenia gravis (CitationThanvi & Lo, 2004), Parkinson’s disease (CitationSoreq & Seidman, 2001) and ataxia (CitationMukherjee et al., 2007a) due to the associated cholinergic deficit (CitationBachurin, 2003). Few of this class of inhibitors have yet been approved for AD therapy (CitationBachurin, 2003) and most of them have short half-life and peripheral cholinergic side effects (CitationFang et al., 2008; CitationMukherjee et al., 2007b; CitationNordberg & Svensson, 1998; CitationPerry et al., 2003) which significantly limit its therapeutic use. Therefore, natural sources of compounds showing AChE inhibitory capacity must be sought.
The enormous economic and social costs of neurodegenerative disorders (CitationBeking & Vieira, 2010; CitationWimo et al., 2011) in conjunction with the lack of any cure, make the development of new therapies for halting or reversing such diseases urgent. The present study strived to identify plant extracts demonstrating antioxidant and AChE inhibitory activities and serving as potential novel therapies for neurodegeneration.
The plants selected were collected in a coastal area of Southwestern Portugal, characterized by soils which are sandy, poor in nutrients and subject to salinity. This site, which is part of Europe’s Natura 2000 network, is classified of community importance for the Mediterranean biogeographical region (Comporta/Galé) and is rich in endemic flora that have been poorly explored. Few pharmacological or phytochemical studies have been carried out on the species of this ecosystem.
Extracts were assayed for antioxidant properties for two of the most important biological free radicals (peroxyl and hydroxyl) and for their inhibitory effects on AChE. HPLC profiles determined their chemical composition.
Materials and methods
Plant materials
In this study, we used leaves from Portuguese endemic plants. The plants in the study were Anchusa calcarea Boiss. (Boraginaceae), Armeria rouyana Daveau (Plumbaginaceae), Santolina impressa Hoffmanns. & Link (Asteraceae), Thymus capitellatus Hoffmanns. & Link (Lamiaceae) and Ulex australis Welw. ex Webb subsp. welwitschianus (Planch.) M. D. Espírito Santo, Cubas, M. F. Lousã, C. Pardo & J. C. Costa (Leguminosae). All samples were collected in Comporta area in 2007 and vouchers sample representative (1069/2007; 1070/2007; 1065/2007; LISI 1071/2007; 1068/2007) were authenticated by Dalila Espírito-Santo, Instituto Superior de Agronomia and deposited on Herbário “João de Carvalho e Vasconcelos”, Instituto Superior de Agronomia, Lisbon. For Armeria rouyana, since it is a Portuguese endemic and threatened plant classified as a priority species (Directive 92/43/EEC, Annex II), we obtained a license for capture (137/2007/CAPT). Plants were collected and stored at −80°C. Prior to extraction, plants were ground.
Chemicals and materials
Acetylthiocholine iodide (ATCI), 5,5-dithiobis-2-nitrobenzoic acid (DTNB), physostigmine, acetylcholinesterase from electric eel (type VI-S; lyophilized powder) and 5,5-dimethyl-1-pyrroline-N-oxide (DMPO) were purchased from Sigma (Sigma-Aldrich).
Extraction of plant phytomolecules
To each 1 g of plant, 6 mL of hydroethanolic solvent were added and extraction was performed as described earlier (CitationTavares et al., 2010a).
Fractionation by solid phase extraction
Hydroethanolic extracts were fractionated by Solid Phase Extraction (SPE) using a Giga tubes 2 g 12 mL−1, C18-E units (Phenomenex®) as described before (CitationTavares et al., 2010b).
Total phenolic content
Determination of total phenolic compounds was performed by the Folin-Ciocalteau method adapted to microplate reader as described in previous work (CitationFortalezas et al., 2010). Gallic acid was used as the standard and results are expressed in mg of gallic acid equivalents per gram of dry weight (mg GAE g−1 dw) of plant material.
Total flavonoid content
Measurement of total flavonoid content was performed by a modification of the AlCl3 complexation method as described before (CitationTavares et al., 2010a).
Free radical scavenging assays
Peroxyl radical scavenging capacity
Peroxyl scavenging capacity of plant extracts was determined by the ORAC method adapted to microplate as described earlier (CitationFortalezas et al., 2010). Trolox was used as standard and results are expressed in μmol of trolox equivalents per gram of plant dry weight (μmol TE g−1 dw).
Hydroxyl radical scavenging assay
The ability of the plant extracts to scavenge the hydroxyl radicals in the presence of hydrogen peroxide was evaluated by electron paramagnetic resonance (EPR) using the spin-trapping agent DMPO, as described previously (CitationTavares et al., 2010a).
HPLC profile
Phytochemical profile was obtained with a LiChrospher C18 column (Merck) on a Thermo Finnigan Surveyor instrument (San Jose, CA, USA) equipped with a diode array detector (Thermo Finnigan-Surveyor), and an electrochemical detector (Dionex, ED40) as previously described (CitationTavares et al., 2010a).
Quantification of ascorbic acid
Ascorbic acid content was quantified in the extract prepared with potassium phosphate buffer (pH 3.5) by an enzymatic method, using the kit K-asco from Megazyme, according to the manufacturer instructions. To confirm and obtain an accurate quantification, it was performed with a new plant extraction using phosphoric acid (6 mL g−1 fw). Ascorbic acid was then determined by a HPLC method validated for organic acids quantification (CitationTavares et al., 2010a).
AChE inhibitory assay
AChE inhibition was determined in a 96-microtiter well plate, based on Ellman’s reaction (CitationEllman et al., 1961). Briefly, 5 µL of extracts (2, 4, 8, 40, 80, 400, 800, 1000, 1500, 2000, 3000 and 4000 µg mL−1) resuspended in methanol containing DMSO 8% were added to 25 µL of 15 mM ATCI in H2O, 125 µL of 3 mM DTNB in 50 mM Tris-HCl buffer containing 0.1 M NaCl and 0.02 M MgCl2 (pH 8.0) and 70 µL of 50 mM Tris-HCl buffer with 0.1% (w/v) BSA (pH 8.0). Changes in absorbance were measured during an 8-min period to assess the spontaneous hydrolysis of the substrate (at λ = 405 nm). Then, 25 µL of 0.226 U mL−1 AChE from electric eel (type VI-S; lyophilized powder) in 50 mM Tris-HCl buffer containing 0.1% (w/v) BSA (pH 8.0) was added and immediately after, microplates were shaken for 15 s and the measurement was repeated during 8 min at λ = 405 nm. The experiments were carried out at 25°C. Effect on AChE activity was calculated as an inhibition percentage (%) of the maximum activity (registered on control wells without inhibitor). Physostigmine was dissolved in methanol and used as positive control. Results were transformed using a nonlinear regression with Origin Pro 6.1 software (OriginLab©, USA) and the IC50 values were determined.
Statistical analysis
Results reported in this work are the average of at least three biological replicates and are represented as the mean ± SD. Differences among treatments were assessed by analysis of variance (CitationPopova et al., 2004) with Tukey HSD (Honest Significant Difference) multiple comparison test (α = 0.05) using SigmaStat 3.10 (Systat).
Results
The Comporta/Galé site, in Southwestern Portugal, offers a wide range of little known plant biodiversity, especially where potential bioactivities are concerned. The antioxidant properties and AChE inhibitory activities of five endemic species (Anchusa calcarea, Armeria rouyana, Santolina impressa, Thymus capitellatus, and Ulex australis welwitschianus; ) were evaluated since both bioactivities are important features in neurodegenerative disease protection.
Figure 1. Studied plants from the region of Comporta in the Southwest of Portugal in their natural habitat. A- A. calcarea, B- A. rouyana, C- T. capitellatus, D- S. impressa, and E- U. australis welwitschianus.
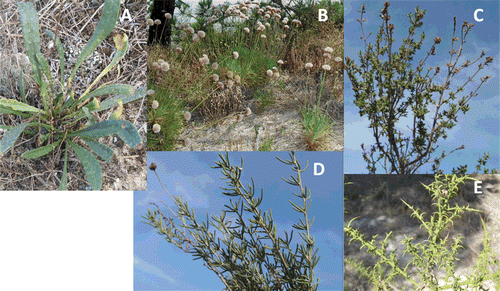
Total phenolic quantification of crude extracts () identified A. rouyana as the richest species, followed by T. capitellatus, U. australis welwitschianus, S. impressa, and, finally, A. calcarea. The total flavonoid content was also determined since flavonoids are a class of polyphenols strongly associated with antioxidant capacity ().
Table 1. Total phenol content, total flavonoid content and antioxidant capacity (peroxyl radical scavenging and hydroxyl radical scavenging capacities) for the hydroethanolic extracts of the five endemic plants from the Southwest of Portugal under study.
Peroxyl (ROO·) and hydroxyl (·OH) are two of the most important free radicals for the human body. These plants’ capacity to scavenge ROO· and ·OH was measured by the Oxygen Radical Absorbance Capacity (ORAC) method and Electron Paramagnetic Resonance (EPR), respectively (). A. rouyana was the most effective scavenger of both radicals; S. impressa presented a lower antioxidant capacity for peroxyl radical than A. rouyana. However, its hydroxyl radical scavenging capacity was similar to that of the most efficient scavenger, A. rouyana.
High Performance Liquid Chromatography with Diode Array Detection and Electrochemical Detection (HPLC-DAD-ED) was used to better understand the antioxidant capacity of these leaf extracts. The resulting phytochemical profile () identified some of the major compounds by way of a study of their UV spectra and comparison with standards, such as those proposed by CitationRobards and Antolovich (1997) for classes of flavonoids. Flavones typically show maximum absorption rates in an intense Band II (310–350 nm) with a shoulder or low intensity Band I (250–280 nm). Flavonols absorb at 250–280 nm (Band II) and 350–385 nm (Band I), while hydroxycinnamic acids demonstrate no Band I and absorb at 227–245 nm and at 310–332 (Band II). Some of the peaks identified by DAD correspond to those detected in ED (). The electrochemical detector identified peaks corresponding to reactive species with strong electron donating capacity. A positive relation between the total area of electrochemical chromatogram peaks () and the antioxidant properties of the extracts was, therefore, expected. The largest ED total peak area was found in the A. rouyana, followed by T. capitellatus, U. australis welwitschianus, S. impressa and A. calcarea (). The former case may be explained by the A. rouyana’s higher flavonoid content (). The ED peaks for U. australis welwitschianus, S. impressa and A. calcarea were hardly detectable ( and ).
Figure 2. Phytochemical analyses of extracts. A) HPLC chromatograms and respective ED profiles B) total peak electrochemical detection for A. calcarea (Ac), A. rouyana (Ar), S. impressa (Si), T. capitellatus (Tc) and U. australis welwitschianus (Uaw). Tentatively, identifications are signaled in chromatograms. Legend: 1-Chlorogenic or caffeic acid; 2-Myrcetin glucoside; 3-l-Ascorbic acid; 4-Rutin derivative; 5-Ferulic acid; 6-Ferulic acid derivative; 7-Luteolin glucoside; 8-Protocatechuic acid derivative; 9-Apigenin glucoside.
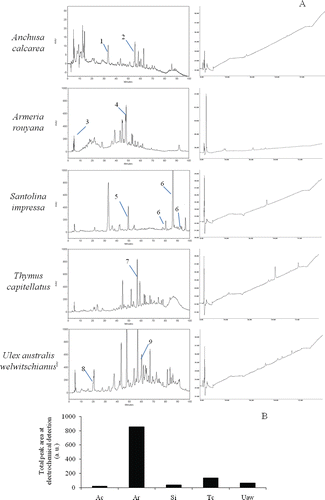
Besides the higher flavonoid content in A. rouyana () which contributes to antioxidant capacity, l-ascorbic acid (, Peak 3) also play an important role in A. rouyana’s antioxidant capacity due to its redox reactivity (ED signals). An enzymatic method, using the K-asco kit from Megazyme (1.98 ± 0.33 mg g−1 dw) confirmed l-ascorbic acid presence and subsequently quantified it by a HPLC validated method (3.27 ± 0.26 mg g−1dw). Rutin derivative (, Peak 4), a flavonol, is also an abundant compound in this plant extract.
T. capitellatus demonstrated high levels of the flavone luteoline glucoside (, Peak 7) which is one of the main compounds corresponding to the reactive peak in ED detection and may therefore constitute an antioxidant. Hydroxycinnamic acid (Peak 1, ) and myrcetin glucoside (Peak 2, ) displayed peaks in A. calcarea. Ferulic acid (Peak 5, ) and its derivatives (Peak 6, ) were the principal components of S. impressa. Finally, only a protocatechuic acid derivative (Peak 8, ) and an apigenin glucoside (Peak 9, ) were identified in U. australis welwitschianus.
The AChE inhibitory activity of hydroethanolic extracts, using a fixed amount of extract (2 mg mL−1) and using AChE from electric eel (type VI-S; lyophilized powder) is presented in , as a percentage of AChE inhibition. This screening revealed that two of the extracts, A. rouyana and T. capitellatus, inhibited the enzyme markedly (>60–70%). Further assays for a range of concentrations (0–4000 µg mL−1) allowed us to estimate the IC50 for both extracts (); similar IC50 ( and ) arose. We performed a SPE fractionation for A. rouyana and T. capitellatus. This provided a polyphenols-enriched fraction (bound fraction) capable of discarding free sugars and organic acids such as ascorbic acid in the unbound fraction. These polyphenol-enriched fractions were identified as the cause of the AChE inhibition in both extracts (), while compounds appearing in unbound fractions did not present inhibitory capacity.
Table 2. Acetylcholinesterase inhibitory activity of hydroethanolic extracts of the five endemic plants from the Southwest of Portugal under study and AChE inhibition is presented as percentage of inhibition using 2 mg mL−1 of extract and for the most effective extracts was calculated the IC50 (μg mL−1). Physostigmine was used as positive control.
Table 3. Acetylcholinesterase inhibitory activity of fractions obtained from SPE fractioning of hydroethanolic extracts of A. rouyana and T. capitellatus. Unbound fraction, that includes sugars and organic acids (including l-ascorbic acid), and Bound fraction, that includes the polyphenols enriched fraction. AChE inhibition is presented as percentage of inhibition using 2 mg mL−1 of fraction.
Figure 3. Nonlinear regression of acetylcholinesterase inhibition profile obtained with Origin Pro 6.1 software (OriginLab©, USA) for plant hydroethanolic extracts. A- A. rouyana, B- T. capitellatus. AChE inhibition was determined based on Ellman’s reaction. Using AChE from electric eel as described in methods. Effect on AChE activity was calculated as an inhibition percentage (%) of the maximum activity (control without inhibitor).
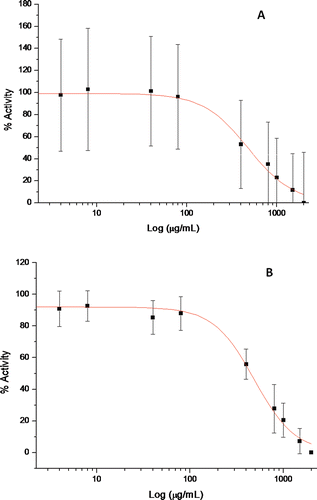
Discussion
In general, plant extracts present a positive relationship between total phenols content, flavonoids content and antioxidant capacity, with higher phenols and flavonoid levels reflecting greater antioxidant capacity. However, some points must be discussed.
S. impressa demonstrated low levels of phenolic compounds and flavonoids as well as limited peroxyl radical antioxidant capacity; however, its hydroxyl radical scavenging capacity was similar to that of A. rouyana, the extract with the highest value (). These results suggest either the existence of polyphenols displaying differential antioxidant potencies for the two radicals or a possible combination of compounds interfering with antioxidant capacity and/or the presence of additional antioxidant molecules other than polyphenols. Such has already been reported for other plant extracts (CitationTavares et al., 2010a). No data are yet available regarding the phenolic and flavonoid leaf content or antioxidant capacity of these species.
Analyses of the phytochemical composition of extracts () reveal ED peaks, corresponding to identified compounds in HPLC-DAD. This suggests that detected compounds are associated with antioxidant properties. l-Ascorbic acid is already recognized as an antioxidant molecule (CitationPadayatty et al., 2003) contributing greatly to the antioxidant capacity of A. rouyana (). A. rouyana and T. capitellatus were found to be the most efficient AChE inhibitors displaying higher rates of inhibition than those plants in the literature (CitationMukherjee et al., 2007a). They also belong to families of species known to be AChE inhibitors (CitationAdewusi et al., 2010). A. rouyana demonstrated an AChE inhibition level of 72% using 1 mg mL−1 of extract, whereas that of T. capitellatus was 75.9%. These extracts were definitely stronger inhibitors than those described in the literature (CitationAdewusi et al., 2010). Among others they surpass the inhibition levels of the following: Albizia adianthifolia (Schumach.) W.F. Wight. (Fabaceae; 51.00 ± 3.40%) (CitationEldeen et al., 2005); Trichilia dregeana Sond. (Meliaceae; 55.00 ± 4.40%; CitationEldeen et al., 2005); Buxus sempervirens L. (Buxaceae; 61.76 ± 0.76%; CitationOrhan et al., 2004); and Lavandula augustifolia Miller (Lamiaceae; 64.30 ± 9.00%; CitationOrhan et al., 2007).
Physostigmine, one of the AChE inhibitors used in Alzheimer’s treatments (CitationMohs et al., 1985), was used in this work as a positive control. As expected, the plant extracts were less effective than this pure compound.
Although well-known AChE inhibitors include alkaloids such as physostigmine and galantamine other sources have been described, including ursolic acid (CitationChung et al., 2001), lignans (CitationEl-Hassan et al., 2003) flavonoids, terpenoids and coumarins (CitationWilliams et al., 2011). We performed a SPE fractionation on A. rouyana and T. capitellatus. The unbound fraction corresponding to sugars and organic acids (including ascorbic acid) and a bound fraction corresponding to the polyphenols were tested for AChE inhibition. shows that polyphenols-enriched fractions demonstrated inhibitory capacity. However, these polyphenols-enriched fractions had a lower AChE inhibitory activity than the total extracts, suggesting an absence of compounds which potentiate the AChE inhibitory capacity of polyphenols.
Polyphenols were abundant in both extracts and associated with part of the antioxidant capacity and with the AChE inhibitory capacity. l-Ascorbic acid also seemed to make an important contribution to antioxidant capacity in A. rouyana, although irrespective of AChE inhibition. In summary, these results highlight the potential of these compounds as candidates for neuroprotective natural products and reinforce the need for their further study.
Conclusions
Due to their antioxidant and AChE inhibitory capacities, A. rouyana and T. capitellatus are considered promising sources of neuroprotective phytomolecules, either as alternative neuroprotective drugs or as leads for synthesizing more effective molecules. The extracts, therefore, deserve to be the targets of bioguided fractionation and further characterization.
Acknowledgments
The authors wish to thank the Fundação para a Ciência e Tecnologia for financial support of C. Santos (SFRH/BPD/26562/2006), L. Tavares (SFRH/BD/37382/2007) and A. Serra (SFRH/BD/19238/2004).
Declaration of interest
The authors report no conflicts of interest. The authors alone are responsible for the content and writing of the paper.
References
- Adewusi EA, Moodley N, Steenkamp V. (2010). Medicinal plants with cholinesterase inhibitory activity: A review. Afr J Biotechnol, 9, 8257–8276.
- Andersen JK. (2004). Oxidative stress in neurodegeneration: cause or consequence? Nat Med, 10 Suppl, S18–S25.
- Bachurin SO. (2003). Medicinal chemistry approaches for the treatment and prevention of Alzheimer’s disease. Med Res Rev, 23, 48–88.
- Beking K, Vieira A. (2010). Flavonoid intake and disability-adjusted life years due to Alzheimer’s and related dementias: a population-based study involving twenty-three developed countries. Public Health Nutr, 13, 1403–1409.
- Chung YK, Heo HJ, Kim EK, Kim HK, Huh TL, Lim Y, Kim SK, Shin DH. (2001). Inhibitory effect of ursolic acid purified from Origanum majorana L on the acetylcholinesterase. Mol Cells, 11, 137–143.
- Eldeen IM, Elgorashi EE, van Staden J. (2005). Antibacterial, anti-inflammatory, anti-cholinesterase and mutagenic effects of extracts obtained from some trees used in South African traditional medicine. J Ethnopharmacol, 102, 457–464.
- El-Hassan A, El-Sayed M, Hamed AI, Rhee IK, Ahmed AA, Zeller KP, Verpoorte R. (2003). Bioactive constituents of Leptadenia arborea. Fitoterapia, 74, 184–187.
- Ellman GL, Courtney KD, Andres V Jr, Feather-Stone RM. (1961). A new and rapid colorimetric determination of acetylcholinesterase activity. Biochem Pharmacol, 7, 88–95.
- Fang L, Kraus B, Lehmann J, Heilmann J, Zhang Y, Decker M. (2008). Design and synthesis of tacrine-ferulic acid hybrids as multi-potent anti-Alzheimer drug candidates. Bioorg Med Chem Lett, 18, 2905–2909.
- Fortalezas S, Tavares L, Pimpão R, Tyagi M, Pontes V, Alves PM, McDougall G, Stewart D, Ferreira RB, Santos CN. (2010). Antioxidant properties and neuroprotective capacity of strawberry tree fruit (Arbutus unedo). Nutrients, 2, 214–229.
- Joyner PM, Cichewicz RH. (2011). Bringing natural products into the fold - exploring the therapeutic lead potential of secondary metabolites for the treatment of protein-misfolding-related neurodegenerative diseases. Nat Prod Rep, 28, 26–47.
- Mandel S, Amit T, Reznichenko L, Weinreb O, Youdim MB. (2006). Green tea catechins as brain-permeable, natural iron chelators-antioxidants for the treatment of neurodegenerative disorders. Mol Nutr Food Res, 50, 229–234.
- Mandel SA, Amit T, Weinreb O, Reznichenko L, Youdim MB. (2008). Simultaneous manipulation of multiple brain targets by green tea catechins: a potential neuroprotective strategy for Alzheimer and Parkinson diseases. CNS Neurosci Ther, 14, 352–365.
- Mohs RC, Davis BM, Johns CA, Mathé AA, Greenwald BS, Horvath TB, Davis KL. (1985). Oral physostigmine treatment of patients with Alzheimer’s disease. Am J Psychiatry, 142, 28–33.
- Mukherjee PK, Kumar V, Mal M, Houghton PJ. (2007a). Acetylcholinesterase inhibitors from plants. Phytomedicine, 14, 289–300.
- Mukherjee PK, Kumar V, Mal M, Houghton PJ. (2007b). In vitro acetylcholinesterase inhibitory activity of the essential oil from Acorus calamus and its main constituents. Planta Med, 73, 283–285.
- Newman DJ, Cragg GM. (2007). Natural products as sources of new drugs over the last 25 years. J Nat Prod, 70, 461–477.
- Nordberg A, Svensson AL. (1998). Cholinesterase inhibitors in the treatment of Alzheimer’s disease: a comparison of tolerability and pharmacology. Drug Saf, 19, 465–480.
- Orhan I, Kartal M, Naz Q, Ejaz A, Yilmaz G, Kan Y, Konuklugil B, Sener B, Choudhary, MI. (2007). Antioxidant and anticholinesterase evaluation of selected Turkish Salvia species. Food Chem, 103, 1247–12254.
- Orhan I, Sener B, Choudhary MI, Khalid A. (2004). Acetylcholinesterase and butyrylcholinesterase inhibitory activity of some Turkish medicinal plants. J Ethnopharmacol, 91, 57–60.
- Padayatty SJ, Katz A, Wang Y, Eck P, Kwon O, Lee JH, Chen S, Corpe C, Dutta A, Dutta SK, Levine M. (2003). Vitamin C as an antioxidant: evaluation of its role in disease prevention. J Am Coll Nutr, 22, 18–35.
- Perry NS, Bollen C, Perry EK, Ballard C. (2003). Salvia for dementia therapy: review of pharmacological activity and pilot tolerability clinical trial. Pharmacol Biochem Behav, 75, 651–659.
- Popova M, Bankova V, Butovska D, Petkov V, Nikolova-Damyanova B, Sabatini AG, Marcazzan GL, Bogdanov S. (2004). Validated methods for the quantification of biologically active constituents of poplar-type propolis. Phytochem Anal, 15, 235–240.
- Ramassamy C. (2006). Emerging role of polyphenolic compounds in the treatment of neurodegenerative diseases: a review of their intracellular targets. Eur J Pharmacol, 545, 51–64.
- Robards K, Antolovich M. (1997). Analytical chemistry of fruit bioflavonoids—a review. Analyst, 122, R11–R34.
- Soreq H, Seidman S. (2001). Acetylcholinesterase–new roles for an old actor. Nat Rev Neurosci, 2, 294–302.
- Tavares L, Carrilho D, Tyagi M, Barata D, Serra AT, Duarte CM, Duarte RO, Feliciano RP, Bronze MR, Chicau P, Espírito-Santo MD, Ferreira RB, dos Santos CN. (2010a). Antioxidant capacity of Macaronesian traditional medicinal plants. Molecules, 15, 2576–2592.
- Tavares L, Fortalezas S, Carrilho C, McDougall GJ, Stewart D, Ferreira RB, Santos CN. (2010b). Antioxidant and antiproliferative properties of strawberry tree tissues. J Berry Res, 1, 3–12.
- Thanvi BR, Lo TC. (2004). Update on myasthenia gravis. Postgrad Med J, 80, 690–700.
- Williams P, Sorribas A, Howes MJ. (2011). Natural products as a source of Alzheimer’s drug leads. Nat Prod Rep, 28, 48–77.
- Wimo A, Jonsson L, Gustavsson A, McDaid D, Ersek K, Georges J, Gulacsi L, Karpati K, Kenigsberg P, Valtonen H.(2011). The economic impact of dementia in Europe in 2008-cost estimates from the eurocode project. Int J Geriatr Psych, DOI: 10. 1002/gps. 2610.