Abstract
Context: Dimethyl dicarboxylate biphenyl (DDB) is a clinically used hepatoprotectant and has also been found to have chemopreventive activity.
Materials and methods: Sixteen novel analogs (5-20) were designed, synthesized, and evaluated for their cancer preventive activity. The 2,2′-bismethyl ester (5-18) and ether (19, 20) DDB analogs were synthesized by insertion of various linear alkyl, short fatty acid, polar, and aromatic groups. All synthesized analogs were evaluated in an in vitro short-term 12-O-tetradecanoylphorbol-13-acetate (TPA)-induced Epstein Barr virus early antigen (EBA-EA) activation assay. Three of the most potent compounds were also tested for inhibitory effects on skin tumor promotion in an in vivo two-stage mouse-skin carcinogenesis test using 7,12-dimethylbenz[a]anthracene (DMBA) as an initiator and TPA as a promoter.
Results: Compound 19 with bisprenyl ethers had the most significant cancer preventive activity (100% inhibition of activation at 1 × 103 mol ratio/TPA, 78.4%, 49.7%, and 10.9% inhibition at 5 × 102, 1 × 102, 1 × 10 mol ratio/TPA, respectively) in vitro. Compound 19 also exhibited a remarkable inhibitory effect on skin tumor promotion in the in vivo two-stage mouse-skin carcinogenesis test.
Discussion and conclusions: Thus, DDB analog 19 could be a valuable candidate as a cancer preventive agent or as a lead for the development of new antitumor promoter drugs.
Introduction
Carcinogenesis is a complex process involving initiation, promotion, and progression steps. The promotion step is a long and reversible process and has been widely studied (CitationItoigawa et al., 2002). Inhibition of this step, which is known as cancer prevention, should be an effective approach to control cancer, and various phytochemicals, such as carotenoids, green tea polyphenols, curcumin, glycyrrhizin and its related compounds, herbs and medicinal plants, have been reported to exhibit cancer preventive ability (CitationNishino et al., 2000). However, despite its recognized effectiveness at blocking the long process of cancer development, relatively limited numbers of studies have been reported on cancer prevention. Therefore, more efforts aimed at the discovery and development of cancer preventive agents are needed. To evaluate cancer preventive ability, a short-term in vitro assay can be applied for determining cancer preventive agents. Epstein–Barr virus (EBV) is known to be activated by tumor promoters to produce early antigens. Inhibition of EBV early antigen (EBV-EA) is used to evaluate antitumor promoting ability (CitationIto et al., 1981).
Dimethyl dicarboxylate biphenyl (DDB, 1), a synthetic analog of schizandrin C () isolated from Fructus Schizandrae chinensis, is a hepatoprotective agent used to treat hepatitis B in China and to treat HBV and HCV in many Asian countries (CitationSun & Liu, 2005; CitationJin et al., 2007). In addition, DDB was shown to reverse multidrug resistant cancer cells, breast carcinoma MCF-7/Adr, KBv200, and Bel7402 in vitro and increase antitumor activity of vincristine to KBv200 xenografts in vivo (CitationJin et al., 2007). DDB also prevented the oncogenic transformation of WB-F344 rat liver epithelial cells induced by 3-methylcholanthrene and 12-O-tetradecanoyl phorbal 13-acetate (TPA) at the doses of 1, 2, and 4 µmol/L (CitationSun & Liu, 2005). In a soft-agar colony formation assay, colony numbers were reduced in transformed cells treated with DDB. Furthermore, DDB could inhibit TPA-induced down-regulation of the gap junctional intercellular communication (GJIC). These findings suggest that DDB has chemopreventive potential.
In our design of new DDB analogs, we found that known DDB analogs with different functional groups were previously synthesized and tested for cancer preventive ability (CitationXie et al., 1995). In addition, while many 2,2′-carboxylate ester derivatives have been covered in various patents and papers, the modification of a 2,2′-bismethylene alcohol DDB intermediate (21) appears to be a new avenue of exploration. A prenylated side chain, which has been found to be effective in cancer chemoprevention studies of other compound classes (CitationTatsuzaki et al., 2010), was a logical first choice. In addition, short unsaturated fatty acid chains, which have resulted in good chemopreventive activity in betulinic acid derivatives, were included in our modification scheme (CitationNakagawa-Goto et al., 2009). Water-solubility is an important factor for drug discovery, because it is always associated with important pharmaceutical drug indices. Thus, a hydrophilic carboxylic acid moiety was incorporated into new DDB analogs by coupling the 2,2′-bismethylene alcohol DDB intermediate with succinic and glutaric anhydrides. Accordingly, in this study, we will discuss the synthesis of new DDB analogs (), structure–activity relationship findings, and EBV-EA inhibition ability. In vivo data of the most potent compounds are also described.
Materials and methods
Chemistry
1H NMR (400 MHz) spectra were measured on a Varian Inova spectrometer with TMS as the internal standard. All chemical shifts are reported in ppm. Mass spectra were measured on a Shimadzu LCMS-2010 (ESI-MS). All reactions were monitored by thin-layer chromatography (TLC) on aluminum sheets (silica gel 60 F254 plate, 20 × 20, Merk). Melting points were recorded on a Fisher Johns melting apparatus without correction. Medium-pressure column chromatography was used in Biotage Flash and Isco companion systems with silica 40 µm columns from Grace Inc. All final compounds were > 95% pure based on high performance liquid chromatography (HPLC). Anhydrous solvents were purchased from commercial suppliers.
General procedure for compounds 5-7 and 9-14
To a solution of 21 in dichloromethane, the appropriate carboxylic acid (5 eq. mole), N-(3-dimethylaminopropyl)-N′-ethylcarbodiimide hydrochloride (5 eq. mole) and 4-dimethylamino pyridine (1 eq. mole) were added and stirred overnight. The reaction mixture was subjected to preparative TLC (hexane-ethyl acetate) without work-up.
Compound 5: Yield, 86%; Colorless prisms; mp: 109°C–110°C; 1H NMR (CDCl3) δ 5.94 (d, J = 1.2 Hz, 2H), 5.92 (d, J = 1.6 Hz, 2H), 4.97 (d, J = 1.2 Hz, 2H), 4.89 (d, J = 1.2 Hz, 2H), 4.06 (s, 6H), 2.18 (t, J = 7.6 Hz, 6H), 1.57 (sext, J = 7.6 Hz, 4H), 0.88 (t, J = 7.2 Hz, 6H); ESI-MS m/z: 678 [M+18(H2O)]+. Compound 6: Yield, 75%; Colorless prisms; mp: 127°C–128°C; 1H NMR (CDCl3) δ 7.92 (d, J = 7.6 Hz, 4H), 7.46–7.52 (m, 2H), 7.36 (t, J = 7.6 Hz, 4H), 5.88 (s, 2H), 5.70 (s, 2H), 5.31 (d, J = 11.6 Hz, 2H), 5.12 (d, J = 11.6 Hz, 2H), 4.07 (s, 3H); ESI-MS m/z: 746 [M+18(H2O)]+. Compound 7: Yield, 85%; Colorless oil; 1H NMR (CDCl3) δ 6.69 (s, 2H), 5.97 (s, 2H), 5.95 (s, 2H), 4.85 (s, 4H), 3.95 (s, 6H), 1.99 (s, 6H); ESI-MS m/z: 464 [M+18(H2O)]+. Compound 9: Yield, 97%; Colorless oil; 1H NMR (CDCl3) δ 6.68 (s, 1H), 5.96 (d, J = 1.4 Hz, 2H), 5.95 (d, J = 1.4 Hz, 2H) 4.86 (s, 4H), 3.93 (s, 6H), 2.22 (t, J = 7.2 Hz, 4H), 1.59 (m, 4H), 0.90 (t, J = 7.2 Hz, 6H); ESI-MS m/z: 520 [M+18(H2O)]+. Compound 10: Yield, 99%; Colorless oil; 1H NMR (CDCl3) δ 6.68 (s, 2H), 5.96 (d, J = 1.5 Hz, 2H), 5.94 (d, J = 1.5 Hz, 2H), 4.85 (s, 4H), 3.93 (s, 6H), 2.23 (t, J = 7.6 Hz, 4H), 1.54 (pent, J = 7.6 Hz, 4H), 1.34–1.25 (m, 4H), 0.88 (t, J = 7.6 Hz, 6H); ESI-MS m/z: 548 [M+18(H2O)]+. Compound 11: Yield, 88%; Colorless oil; 1H NMR (CDCl3) δ 6.68 (s, 2H), 5.96 (d, J = 1.5 Hz, 2H), 5.94 (d, J = 1.5 Hz, 2H), 4.85 (s, 4H), 3.93 (s, 6H), 2.24–2.20 (m, 4H), 1.57–1.19 (m), 0.87 (t, J = 6.8 Hz, 6H); ESI-MS m/z: 744 [M+18(H2O)]+. Compound 12: Yield, 8%; Colorless oil; 1H NMR (CDCl3) δ 6.96–6.87 (m), 6.7 (s, 2H), 5.94 (s, 4H), 5.78 (d, J= 15.6 Hz, 2H), 4.94 (d, J = 12.4 Hz, 2H), 4.85 (d, J = 12.4 Hz, 2H), 3.93 (s, 6H), 1.85 (d, J = 6.8 Hz, 6H); ESI-MS m/z: 544 [M+46(HCOOH)]+. Compound 13: Yield, 51%; Colorless oil; 1H NMR (CDCl3) δ 6.70 (s, 2H), 5.94 (d, J = 1.5 Hz, 2H), 5.93 (d, J = 1.5 Hz, 2H), 5.62 (s, 2H), 4.95 (d, J = 12.5 Hz, 2H), 4.82 (d, J = 12.5 Hz, 2H), 3.93 (s, 6H), 2.12 (s, 6H), 1.86 (s, 6H); ESI-MS m/z: 568 [M+42(CH3CN+H)]+. Compound 14: Yield, 55%; Colorless oil; 1H NMR (CDCl3) δ 7.19 (d, J = 9.8 Hz, 1H), 7.15 (d, J = 9.8 Hz, 1H), 6.70 (s, 2H), 6.18–6.08 (m, 4H), 5.93 (d, J = 1.5 Hz, 4H), 5.71 (s, 1H), 5.68 (s, 1H), 4.96 (d, J = 12.3 Hz, 4H), 4.87 (d, J = 12.3 Hz, 4H), 3.93 (s, 6H), 1.83 (d, J= 5.2 Hz, 6H); ESI-MS m/z: 516 [M-34]+.
General procedure for compounds 8, 17, and 18
Compound 21 and triethylamine (5-10 eq. mole) were first added to anhydrous dichloromethane, and then the appropriate acyl chloride (2.2 eq. mole) was added at 0°C under nitrogen. The reaction was warmed gradually to room temperature and stirred for 1–3 h. After the reaction was completed, water and saturated sodium carbonate solution were added and the reaction mixture was extracted with dichloromethane, dried over sodium sulfate, and concentrated. Further purification was done by combiflash (hexane-ethyl acetate gradient).
Compound 8: Yield, 99%; Colorless oil; 1H NMR (CDCl3) δ 6.68 (s, 2H), 5.95 (d, J = 6.5 Hz, 4H), 4.85 (s, 4H), 3.93 (s, 6H), 2.26 (q, J = 7.2 Hz, 4H), 1.08 (t, J = 7.6 Hz, 6H); ESI-MS m/z: 492 [M+18(H2O)]+. Compound 17: Yield, 85%; Orange amorphous solid; 1H NMR (CDCl3) δ 7.43 (s, 2H), 7.34 (d, J = 15.6Hz, 2H), 6.73 (s, 2H), 6.58 (d, J = 1.2 Hz, 2H), 6.44 (m, 2H), 6.22 (d, J = 16 Hz, 2H), 5.95 (d, J = 2.4 Hz, 4H), 5.01 (d, J = 12.4 Hz, 2H), 4.95 (d, J = 12.4 Hz, 2H), 3.93 (s, 6H); ESI-MS m/z: 620 [M+18(H2O)]+. Compound 18: Yield, 85%; Colorless amorphous solid; 1H NMR (CDCl3) δ 7.53 (m, 2H), 7.34 (s, 2H), 6.77 (s, 2H), 6.75 (s, 2H), 5.99 (s, 4H), 5.93 (s, 2H), 5.88 (s, 2H), 5.10 (d, J = 12.4 Hz, 2H), 5.02 (d, J = 12.4 Hz, 2H), 3.93 (s, 6H); ESI-MS m/z: 676 [M+18(H2O)]+.
Compounds 15 and 16
Succinic anhydride (for 15) or glutaric anhydride (for 16) (1.2 eq. mole) and DMAP (5% w/w) was added to a flask containing 21 in anhydrous tetrahydrofuran. The solution was refluxed under nitrogen overnight. A solution of 1N hydrochloric acid was added to acidify the reaction mixture and ethyl acetate was used three times successively for extraction of the aqueous layer. Preparative TLC (dichloromethane–methanol) was applied to purify the desired compound from the combined aqueous extracts.
Compound 15: Yield, 28%; Colorless oil; 1H NMR (CDCl3) δ 6.67 (s, 2H), 5.96 (d, J = 16.8 Hz, 4H), 4.94 (d, J = 12.6 Hz, 2H), 4.89 (d, J = 12.6 Hz, 2H), 3.93 (s, 6H), 2.59–2.54 (m, 8H); ESI-MS m/z: 580 [M+18(H2O)]+. Compound 16: Yield, 7%; Colorless oil; 1H NMR (CDCl3) δ 6.68 (s, 2H), 5.96 (d, J = 13.6 Hz, 4H), 4.90 (d, J = 12.5 Hz, 2H), 4.84 (d, J = 12.5 Hz, 2H), 2.38–2.31 (m, 8H), 1.93–1.84 (m, 4H); ESI-MS m/z: 608 [M+18(H2O)]+.
Compounds 19 and 20
Compound 21 in anhydrous tetrahydrofuran was added slowly to a flask with sodium hydride (5 eq. mole) in anhydrous tetrahydrofuran under nitrogen at 0°C. After 10 min, 3,3-dimethylallyl bromide (3 eq. mole for 19) or geranyl bromide (3 eq. mole for 20) was added. When the starting material disappeared, water was added to quench the reaction. The aqueous solution was partitioned with ethyl acetate. The organic layer was washed with sodium bicarbonate solution and then dried over sodium sulfate. Desired compounds were purified by preparative TLC with a hexane-ethyl acetate system.
Compound 19: Yield, 43%; Colorless oil; 1H NMR (CDCl3) δ 6.78 (s, 2H), 5.92 (s, 4H), 5.23 (m, 2H), 4.23 (d, J = 12.1 Hz, 2H), 4.16 (d, J = 12.1 Hz, 2H), 3.94 (s, 6H), 3.82 (d, J = 6.9 Hz, 4H), 1.70 (s, 6H), 1.59 (s, 6H); ESI-MS m/z: 521 [M+23(Na)]+. Compound 20: Yield, 47%; Colorless oil; 1H NMR (CDCl3) δ 6.76 (s, 1H), 6.69 (s, 1H), 5.69–5.93(m, 4H), 5.11–5.05 (m, 2H), 4.39–4.11 (m, 4H), 3.94 (s, 6H), 3.84 (m, 2H), 2.10–1.95 (m, 4H), 1.67 (s, 3H), 1.58 (s, 3H), 1.57 (s, 3H); ESI-MS m/z: 481 [M-17(OH)]+.
In vitro EBV-EA activation experiment
Raji cells (106 cells/mL) were incubated at 37°C for 48 h in RPMI-1640 medium with 10% fetal calf serum (FCS), n-butyric acid (4 mmol), TPA (32 pmol), and test compounds. Smears were made from the cell suspension, and the EBV-EA inducing cells were stained by an indirect immunofluorescence technique. In each assay, at least 500 cells were counted and the number of stained cells (positive cells) was recorded. Each assay was repeated three times for one test compound. The EBV-EA-inhibiting activity of the test compound was estimated on the basis of the percentage of the number of positive cells compared with that of the control without the test compound. The viability of the cells was assayed by the Trypan Blue staining method. For the determination of cytotoxicity, the cell viability was required to be more than 60% (CitationIwase et al., 2000).
In vivo two-stage mouse skin carcinogenesis test
A total of 30 female imprinting control region (ICR) mice (6 weeks old, purchased from SLC Co. Ltd., Shizouka, Japan) were used. Two groups, with each group consisting of 15 animals, housed at five/cage, were painted with 390 nmol of 7,12-dimethylbenz[a]anthracene (DMBA) in acetone, 0.1 mL/mouse, on a shaved region of skin on the back. After 1 week, the mice were treated topically with 1.7 nmol of TPA in acetone (0.1 mol) twice a week for 20 weeks. One hour prior to TPA treatment, the animals in group I were treated with acetone (0.1 mL) alone, serving as a promotion-positive control. The animals in group II were treated with the test compound (85 nmol) in acetone (0.1 mL). The incidence of papilloma was observed weekly for 15 weeks. The differences in the occurrence of mouse skin papillomas between the control and treatment groups were analyzed by means of the Student’s t-test after 15 weeks of promotion.
Results and discussion
Chemistry
DDB analogs () were synthesized following literature methods (CitationXie et al., 1995). Reduction of 1 and 3,3′-dibromo-DDB (2) with diisobutylaluminum hydride (DIBAL) resulted in the related 2,2′-methylene alcohols 21 and 3, respectively (). Diols 21 and 3 were then converted to various ester and ether analogs as shown in . Esterifications of 21 and 3 were carried out in the presence of either excess carboxylic acid, 1-ethyl-3-(3-dimethylaminopropyl)carbodiimide (EDCI), and 4-dimethylaminopyridine (DMAP) or acyl chloride and triethylamine at 0°C. A hydrophilic group was introduced by coupling with succinic anhydride or glutaric anhydride. Bisprenyl ether 19 and mono-geranyl ether 20 were obtained by Williamson ether synthesis of 21 with prenylbromide and geranylbromide, respectively, in the presence of sodium hydride. It should be noted that the bisgeranyl ether was not stable and decomposed easily after purification. Therefore, only the mono-geranyl ether 20 was obtained.
Table 1. DDB analogs and their EBV-EA inhibition ability.
In vitro EBV-EA inhibition of DDB analogs
All analogs were evaluated in a short-term in vitro EBV-EA inhibition assay to determine their cancer prevention potential, and the results are shown in . All tested compounds showed relatively potent inhibition of EBV activation. The analogs with unsaturated alkyl side chains and terminal carboxylic acids, such as 12–16, 19, and 20, significantly inhibited EBV-EA activation, showing 95.9%–100% inhibition at the highest tested concentration, and showed greater inhibitory effects than the parent compound 1. In particular, the most potent compound 19 displayed 100% inhibition at 1 × 103 mol ratio/TPA, and 78.4, 49.7, and 10.9% inhibition at 5 × 102, 1 × 102, 1 × 10 mol ratio/TPA, respectively, with an IC50 value of 252 mol ratio/TPA. At the higher concentrations of 1 × 103 and 5 × 102 mol ratio/TPA, the inhibition values with 19 were comparable to those of curcumin, which is a known potent cancer preventive agent. Moreover, even at low concentrations, 19 inhibited EBV-EA activation and the inhibitory effects of 19 were notably greater than those of curcumin at 1 × 102 and 1 × 10 mol ratio/TPA. The analogs with prenyl-like unsaturated alkyl groups, such as 12–14, 19, and 20 exhibited relatively high activity. This finding is consistent with other reports that a prenyl-like group tends to enhance the inhibitory effect on EBA activation (CitationTatsuzaki et al., 2010). The presence of an aromatic ring on the C-2,2′ side chain, as found in 6, 17, and 18, reduced the inhibitory effect on EBV-EA activation. The effect of bromide depended on the functional group at C-2 and -2′. With 2,2′-biscarbomethoxy substitution, the 3,3′-dibromo analog 2 showed lower potency than the parent compound 1, while with 2,2′-bisbutyryloxymethyl substitution, the 3,3′-dibromo analog 5 showed higher potency than the related nonbrominated compound 9. The esters 7–10 with linear saturated fatty acids of varying lengths demonstrated almost similar potency, indicating that the length of the alkyl chain is not crucial for the activity; however, the activity decreased with a chain length of 12 carbons (analog 11). Compounds 15 and 16 with terminal carboxylic acids on the 2,2′-fanctional groups exhibited better activity than the parent compound 1. In a direct comparison, the succinate side chain (15) was better than glutarate side chain (16) in terms of potency.
In vivo mouse skin carcinogenesis inhibition
The in vitro inhibitory effects determined in the EBV-EA assay generally have been found to correlate well with in vivo inhibitory effects on tumor promotion as reported in many studies (CitationKonoshima et al., 1994; CitationIshida et al., 2000, Citation2002; CitationSakurai et al., 2003; CitationWang et al., 2006). Therefore, based on the in vitro data, only three of the most potent compounds (13, 15, and 19) were examined in a two-stage in vivo skin carcinogenesis test evaluating mouse skin papilloma induced by DMBA as an initiator and TPA as a promoter (). The compounds’ activities were determined by both the percentage of papilloma-bearing mice () and the average number of papillomas/mouse (), compared with the positive control. All three compounds delayed the appearance of the first tumor for 2 weeks compared with the positive control. In the positive control group, 6.6, 40, and 100% of the mice bore papillomas after 6, 8, and 11 weeks of promotion, respectively, and 6.3 papillomas were formed/mouse after 15 weeks. However, in the groups treated with compounds 13 and 19, 0, 7, and 33% of the mice bore papillomas at weeks 6, 8, and 11, respectively, and 4.4–5.1 papillomas/mouse were found with all three tested compounds, even after 15 weeks of promotion.
Table 2. In vivo inhibitory effects of 13, 15, and 19 on two-stage mouse carcinogenesis.
Figure 3. Inhibitory effects of compounds 13, 15, and 19 on DMBA–TPA mouse skin carcinogenesis. Tumor formation in all mice was initiated with DMBA (390 nmol) and promoted with TPA (1.7 nmol) twice weekly beginning 1 week after initiation. (A) Papilloma percentage in mice. (B) Average number of papillomas/mouse. (♦) Control TPA alone; (▪) TPA + compound 13 (85 nmol); (×) TPA+ compound 15 (85 nmol); (▴) TPA +compound 19 (85 nmol). After 15 weeks of promotion, a significant difference in the number of papillomas/mouse between the treated groups and the control group was evident (p <0.05). In , the trace for compound 19 is superimposed with that for compound 13.
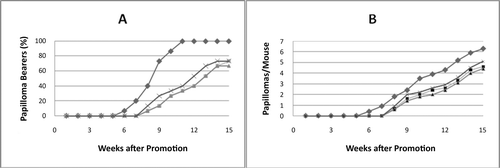
Conclusions
Several 2,2′-bismethyl ester and ether DDB analogs were designed and synthesized. All analogs showed potent EBV-EA inhibition in vitro. Among them, analogs 12–16, 19, and 20 with unsaturated side chains or terminal carboxylic acids significantly inhibited the EBV-EA activation. In particular, prenyl derivative 19 showed the highest inhibitory effects (100%, 78.4%, 49.7% and 10.9% inhibition at 1 × 103, 5 × 102, 1 × 102, 1 × 10 mol ratio/TPA, respectively), which were greater than those of curcumin at the low concentrations. In an in vivo assay, DDB analogs 13, 15, and 19 also delayed the formation of mouse skin papillomas after initiation and promotion by a cancer promoting substance. The DDB has been used clinically, which implies that DDB analogs have good probability to be further developed as potent cancer preventive agents for clinical use. Thus, DDB analog 19 could be a valuable candidate as a cancer preventive agent or as a lead for the development of new antitumor promoter drugs.
Declaration of interest
This study was supported in part by NIH grant CA17625-32 from the National Cancer Institute awarded to K. H. Lee, and also in part by the Department of Health Cancer Research Center of Excellence (DOH-100-TD-C-111-05). The authors alone are responsible for the content and writing of the paper.
References
- Ishida J, Kozuka M, Wang H, Konoshima T, Tokuda H, Okuda M, Yang Mou X, Nishino H, Sakurai N, Lee KH, Nagai M. (2000). Antitumor-promoting effects of cyclic diarylheptanoids on Epstein-Barr virus activation and two-stage mouse skin carcinogenesis. Cancer Lett, 159, 135–140.
- Ishida J, Kozuka M, Tokuda H, Nishino H, Nagumo S, Lee KH, Nagai M. (2002). Chemopreventive potential of cyclic diarylheptanoids. Bioorg Med Chem, 10, 3361–3365.
- Ito Y, Yanase S, Fujita J, Harayama T, Takashima M, Imanaka H. (1981). A short-term in vitro assay for promoter substances using human lymphoblastoid cells latently infected with Epstein-Barr virus. Cancer Lett, 13, 29–37.
- Itoigawa M, Ito C, Ju-ichi M, Nobukuni T, Ichiishi E, Tokuda H, Nishino H, Furukawa H. (2002). Cancer chemopreventive activity of flavanones on Epstein-Barr virus activation and two-stage mouse skin carcinogenesis. Cancer Lett, 176, 25–29.
- Iwase Y, Takemura Y, Ju-ichi M, Ito C, Furukawa H, Kawaii S, Yano M, Mou XY, Takayasu J, Tokuda H, Nishino H. (2000). Inhibitory effect of flavonoids from citrus plants on Epstein-Barr virus activation and two-stage carcinogenesis of skin tumors. Cancer Lett, 154, 101–105.
- Jin J, Sun H, Wei H, Liu G. (2007). The anti-hepatitis drug DDB chemosensitizes multidrug resistant cancer cells in vitro and in vivo by inhibiting P-gp and enhancing apoptosis. Invest New Drugs, 25, 95–105.
- Konoshima T, Takasaki M, Tatsumoto T, Kozuka M, Kasai R, Tanaka O, Nie RL, Tokuda H, Nishino H, Iwashima A. (1994). Inhibitory effects of cucurbitane triterpenoids on Epstein-Barr virus activation and two-stage carcinogenesis of skin tumors. Biol Pharm Bull, 17, 668–671.
- Nakagawa-Goto K, Yamada K, Taniguchi M, Tokuda H, Lee KH. (2009). Cancer preventive agents 9. Betulinic acid derivatives as potent cancer chemopreventive agents. Bioorg Med Chem Lett, 19, 3378–3381.
- Nishino H, Tokuda H, Satomi Y, Masuda M, Onozuka M, Yamaguchi S, Takayasu J, Tsuruta J, Takemura M, Ii T, Ichiishi E, Kuchide S, Okuda M, Murakoshi M. (2000). Cancer Chemoprevention by Phytochemicals and their Related Compounds. Asian Pac J Cancer Prev, 1, 49–55.
- Sakurai N, Kozuka M, Tokuda H, Nobukuni Y, Takayasu J, Nishino H, Kusano A, Kusano G, Nagai M, Sakurai Y, Lee KH. (2003). Antitumor agents 220. Antitumor-promoting effects of cimigenol and related compounds on Epstein-Barr virus activation and two-stage mouse skin carcinogenesis. Bioorg Med Chem, 11, 1137–1140.
- Sun H, Liu GT. (2005). Chemopreventive effect of dimethyl dicarboxylate biphenyl on malignant transformation of WB-F344 rat liver epithelial cells. Acta Pharmacol Sin, 26, 1339–1344.
- Tatsuzaki J, Nakagawa-Goto K, Tokuda H, Lee KH. (2010). Cancer preventive agents 10. Prenylated dehydrozingerone analogs as potent chemopreventive agents. J Asian Nat Prod Res, 12, 227–232.
- Wang X, Nakagawa-Goto K, Kozuka M, Tokuda H, Nishino H, Lee KH. (2006). Cancer preventive agents. Part 6: Chemopreventive potential of furanocoumarins and related compounds. Pharmaceut Biol, 44, 116–120.
- Xie L, Xie JX, Kashiwada Y, Cosentino LM, Liu SH, Pai RB, Cheng YC, Lee KH. (1995). Anti-AIDS (acquired immune deficiency syndrome) agents. 17. New brominated hexahydroxybiphenyl derivatives as potent anti-HIV agents. J Med Chem, 38, 3003–3008.