Abstract
Context: Orofacial dyskinesia (OD) is a late complication of prolonged neuroleptic treatment characterized by involuntary movements of the oral region. Chronic treatment with neuroleptics leads to development of vacuous chewing movements (VCMs). VCMs in rats are widely accepted as an animal model of OD.
Objective: To study the effect of Murraya koenigii L. (Rutaceae) leaves on haloperidol-induced OD.
Materials and methods: Effect of alcohol extract of M. koenigii leaves (EEMK) and its alkaloid fraction (AMK) on body weight, locomotor activity, behavioral parameters, such as VCMs, tongue protrusions (TPs), orofacial bursts (OBs), and biochemical parameters such as antioxidant defense enzymes levels [superoxide dismutase (SOD) and catalase (CAT)], glutathione (GSH) levels, and lipid peroxidation (LPO) in the forebrain region was studied in haloperidol-treated rats.
Results: Rats chronically treated with haloperidol (1 mg/kg, i.p., 21 days) significantly decreased locomotion and developed VCMs, OBs, and TPs. Biochemical analysis reveals that chronic haloperidol-treated rats also showed decreased levels of SOD and CAT. Chronic haloperidol treatment significantly induced LPO and decreased the forebrain GSH levels in the rats. Co-administration of EEMK (100 and 300 mg/kg, p.o.) and AMK (30 and 100 mg/kg, p.o.) along with haloperidol significantly reversed the effect on locomotion. EEMK and AMK significantly reversed the haloperidol-induced decrease in forebrain SOD and CAT levels in rats and significantly reduced the LPO and restored the decreased GSH levels by chronic haloperidol treatment.
Conclusion: The study concludes that M. koenigii could be screened as a potential drug for the prevention or treatment of neuroleptic-induced OD.
Introduction
Haloperidol is a widely used neuroleptic drug for the treatment of acute and chronic psychosis. The use of typical antipsychotics such as haloperidol is limited by their tendency to produce a range of extrapyramidal movement disorders such as Parkinson’s disease, akathesia, dystonia, and tardive dyskinesia (TD). TD occurs in 20–40% of the patient population undergoing chronic neuroleptic therapy. TD is characterized by repetitive involuntary movements, usually involving the mouth, face, and tongue, and sometimes limb and trunk musculature (CitationEgan et al., 1997; CitationCasey, 2000; CitationKulkarni & Naidu, 2001). TD may persist for months or years after drug withdrawal and, in some patients, it is irreversible. Because of its severity, high incidence, and potential irreversibility, TD is considered as a major clinical and ethical issue in psychiatry.
Oxidative stress and products of lipid peroxidation (LPO) are implicated in the etiopathology of TD (CitationCadet et al., 1986; CitationCoyle & Puttfarcken, 1993; CitationAndreassen & Jorgensen, 2000). Chronic treatment with neuroleptics is reported to increase free radical production and oxidative stress (CitationBalijepalli et al., 2001). Haloperidol increases oxidative stress in brain tissue (CitationSagara, 1998).
CitationElkashef and Wyatt (1999) reported that rats with vacuous chewing movements (VCMs) had significantly higher thiobarbituric acid reactive substances (TBARS) in the striatum, suggesting increased LPO and free radical production in these animals. The chronic use of neuroleptics is also reported to cause decreases in the activity of antioxidant defense enzymes superoxide dismutase (SOD) and catalase (CAT; CitationCadet et al., 1987). Free radicals are thought to play a role in aging process, and age is one of the risk factors for the development of TD. A positive correlation exists between increasing age and the development of TD, further supporting the role of free radicals in the pathogenesis of TD (CitationRichardson et al., 1986). Vitamin E, an antioxidant and free radical scavenger, has been reported to be effective in the treatment of TD (CitationElkashef & Wyatt, 1999; CitationGupta et al., 1999; CitationSoares & Mcgrath, 2002), suggesting the potential role of free radicals in the pathogenesis of TD.
Murraya koenigii L (Rutaceae) is a rich source of carbazole alkaloids having antioxidant activity (CitationZheng & Wang, 2001). Hence, in this study, we explored the potential of M. koenigii through the use of behavioral and biochemical models of orofacial dyskinesia (OD) in rats. In the present study, we investigated the effect of alcoholic extract of M. koenigii leaves (EEMK) and its alkaloid fraction (AMK) on haloperidol-induced OD. The effect of EEMK and AMK on locomotor activity and biochemical parameters such as protective antioxidant enzyme levels (SOD and CAT), glutathione (GSH) levels, and LPO in the forebrain region was also studied in haloperidol-treated rats.
Methods
Animals
Male albino Wistar rats (150 ± 20 g) were obtained from Bharat Serum and Vaccines Ltd., Thane. Animals were housed in five groups at ambient temperature of 25 ± 1°C. Animals had free access to water and food. They were deprived of food but not water 4 h before the experiment. The experiments were carried out between 9.00 and 14.00 h. All the experimental procedures and protocols used in this study were approved by the Institutional Animal Ethics Committee of M.G.V.’s Pharmacy College, Nashik. Ethical guidelines were strictly followed during all the experiments.
Plant material
Leaves of M. koenigii were obtained from Aushadhi Bhavan, Ayurved Seva Sangh, Nashik in August, 2010, and identified by Dr. P.G. Diwakar, Jt. Director, Botanical Survey of India, Pune, where a voucher specimen (MUKKID 1) has been retained.
Leaves of M. koenigii were dried, powdered, and defatted with the petroleum ether (60–80°C) using Soxhlet’s extractor. The petroleum ether was removed and marc was air dried. Thereafter marc was successively extracted with ethanol. The extract was concentrated and evaporated to dryness. The yield of crude alcohol extract of M. koenigii (EEMK) was found to be 5.6% w/w. EEMK was further subjected to the isolation of alkaloids as described earlier (CitationCordell, 1981). Yield of AMK was found to be 49.37% w/w.
Drugs and treatment schedule
Haloperidol (1 mg/kg, i.p.; Serenace, Searle India, India) was diluted with distilled water and administered intraperitoneally once daily in the morning (1100 h). EEMK (100 and 300 mg/kg), AMK (30 and 100 mg/kg), and vitamin E (10 mg/kg) were administered orally once per day (1000 h) for a period of 21 days in a constant volume of 0.5 mL/100 g body weight of rat. Behavioral assessments were done 24 h after the last dose of haloperidol. Drug doses were selected on the basis of previous studies conducted in our laboratory. Group I received vehicle. Group II received haloperidol (1 mg/kg) alone. Group III received EEMK (100 mg/kg) + haloperidol (1 mg/kg). Group IV received EEMK (300 mg/kg) + haloperidol (1 mg/kg). Group V received AMK (30 mg/kg) + haloperidol (1 mg/kg). Group VI received AMK (100 mg/kg) + haloperidol (1 mg/kg). Group VII received vitamin E (10 mg/kg) + haloperidol (1 mg/kg).
Assessment of OD
Behavioral parameters
Rats were placed individually in a small (22 × 22 × 22 cm) Plexiglas cage immediately after administration of haloperidol. The number of VCM, along with number of orofacial burst (OB) and tongue protrusion (TP) was scored for the period of 1 h (CitationSalamone et al., 1986).
Locomotor activity
To analyze changes in spontaneous locomotor activity caused by treatment with haloperidol and/or EEMK or AMK, the animals were placed individually in the center of an open-field arena divided into 16 equal squares, as previously described (CitationVogel & Vogel, 2002). The number of line crossings was measured over 5 min and taken as an indicator of locomotor activity.
Body weight change
Haloperidol is known to increase body weight (CitationFell et al., 2004). Effect of EEMK and AMK on body weight of rats after treatment with haloperidol was observed.
Dissection and homogenization
The animals were sacrificed by decapitation immediately after behavioral assessments. The brain was removed; forebrain was dissected out and was rinsed with isotonic saline and weighed. A 10% (w/v) tissue homogenate was prepared in 0.1 M phosphate buffer (pH = 7.4), the postnuclear fraction for CAT assay was obtained by the centrifugation of homogenate at 1000g for 20 min at 4°C, and for other enzyme assays, the postnuclear fraction was obtained by centrifugation at 12,000g for 60 min at 4°C.
Superoxide dismutase
The assay of SOD was based on the ability of SOD to inhibit spontaneous oxidation of adrenaline to adrenochrome (CitationMisra & Fridivich, 1972; CitationSaggu et al., 1989). To 0.05 mL supernatant, 2.0 mL of carbonate buffer and 0.5 mL of EDTA were added. The reaction was initiated by the addition of 0.5 mL of epinephrine, and the auto-oxidation of adrenaline (3 × 10−4 M) to adrenochrome at pH 10.2 was measured by following change in OD at 480 nm. The change in OD every minute was measured at 480 nm against reagent blank. The results were expressed as units of SOD activity (mg/protein). One unit of SOD activity induced ˜50% inhibition of adrenaline. The results were expressed as nmol SOD U/mg wet tissue.
Catalase
The CAT activity assay was based on the ability of CAT to induce the disappearance of hydrogen peroxide (CitationBeers & Sizer, 1952). The reaction mixture consisted of 2 mL phosphate buffer (pH = 7.0), 0.95 mL of hydrogen peroxide (0.019 M), and 0.05 mL supernatant in final volume of 3 mL. Absorbance was recorded at 240 nm every 10 s for 1 min. One unit of CAT was defined as the amount of enzyme required to decompose 1 µmol of peroxide per min, at 25°C and pH = 7.0. The results were expressed as units of CAT activity (mg protein). Units of activity were determined from the standard graph of H2O2. The results were expressed as CAT U/mg wet tissue.
Reduced glutathione
Reduced GSH (S-glutamyl cisteinyl glycine) plays an important role in the protection of cells against damage from endogenous and exogenous free radicals and oxidants. Reduced GSH was determined by the method of CitationEllman (1959). To the homogenate, 10% TCA was added, centrifuged, and treated with 1.0 mL of Ellman reagent [19.8 mg of 5,5′-dithiobisnitro benzoic acid in 100 mL of 1.0% sodium citrate and 3 mL of phosphate buffer (pH = 8.0)]. The color developed was measured at 412 nm. The results were expressed as nmol GSH/mg wet tissue
Lipid peroxidation
LPO as evidenced by the formation of TBARS was measured by the method of CitationNiehaus and Samuelson (1968). In brief, 0.1 mL of homogenate (Tris-HCl buffer, pH 7.5) was treated with 2 mL of (1:1:1 ratio) TBA-TCA-HCl reagent (thiobarbituric acid 0.37%, 0.25 N HCl, and 15% TCA) and placed in water bath for 15 min, cooled, and centrifuged at room temperature for 10 min at 1000 rpm. The absorbance of clear supernatant was measured against reference blank at 535 nm. The results were expressed as LPO nmol/mg wet tissue.
Statistical analysis
The mean ± SEM values were calculated for each group. One-way ANOVA followed by Dunnett’s multiple comparison tests were used for statistical analysis. Values of p < 0.05 were considered statistically significant.
Results
Behavioral effects of chronic haloperidol or M. koenigii treatment in rats
Haloperidol (1 mg/kg) treatment significantly increased the frequency of VCMs, OBs, and TPs in rats compared with vehicle-treated controls. EEMK (100 and 300 mg/kg) and AMK (30 and 100 mg/kg) significantly attenuated haloperidol-induced VCM (), OB (), or TP ().
Figure 1. Effect of Murraya koenigii on haloperidol-induced VCMs in rats. n = 5. The observations are mean ± SEM. # p < 0.05 compared with vehicle-treated group. * p < 0.05 compared with haloperidol-treated group. One-way ANOVA followed by Dunnett’s test. HPL: Haloperidol, EEMK: alcohol extract of Murraya koenigii leaves, AMK: alkaloids isolated from EEMK.
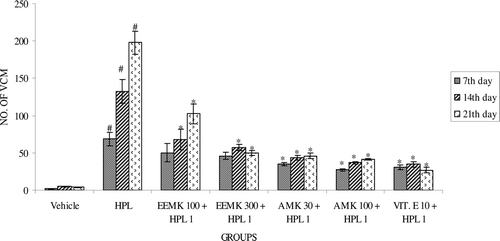
Figure 2. Effect of Murraya koenigii on haloperidol-induced OB in rats. n = 5. The observations are mean ± SEM. # p < 0.05 compared with vehicle-treated group. * p < 0.05 compared with haloperidol-treated group. One-way ANOVA followed by Dunnett’s test. HPL: Haloperidol, EEMK: alcohol extract of Murraya koenigii leaves, AMK: alkaloids isolated from EEMK.
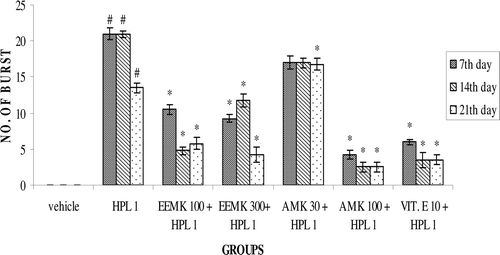
Figure 3. Effect of Murraya koenigii on haloperidol-induced TPs in rats. n = 5. The observations are mean ± SEM. # p < 0.05 compared with vehicle-treated group. * p < 0.05 compared with haloperidol-treated group. One-way ANOVA followed by Dunnett’s test. HPL: Haloperidol, EEMK: alcohol extract of Murraya koenigii leaves, AMK: alkaloids isolated from EEMK.
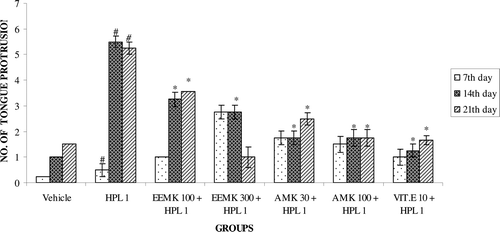
Effect of M. koenigii on locomotor activity
Haloperidol treatment decreases the number of squares traversed by rats. Co-administration of EEMK (100 and 300 mg/kg) and AMK (30 and 100 mg/kg) significantly increased the number of squares traversed compared with haloperidol-treated group (). Haloperidol treatment exhibited significant increase in the number of grooming in rats. Treatment with EEMK (100 and 300 mg/kg) and AMK (30 and 100 mg/kg) significantly reduced the number of grooming ().
Figure 4. Effect of Murraya koenigii on number of squares traveled in haloperidol-treated rats. n = 5. The observations are mean ± SEM. # p < 0.05 compared with vehicle-treated group. * p < 0.05 compared with haloperidol-treated group. One-way ANOVA followed by Dunnett’s test. HPL: Haloperidol, EEMK: alcohol extract of Murraya koenigii leaves, AMK: alkaloids isolated from EEMK.
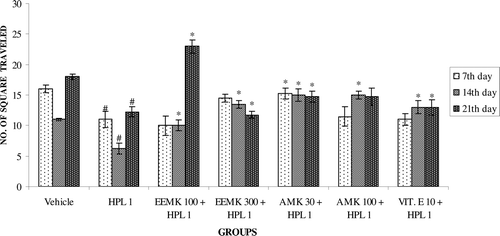
Figure 5. Effect of Murraya koenigii on grooming in haloperidol-treated rats. n = 5. The observations are mean ± SEM. # p < 0.05 compared with vehicle-treated group. * p < 0.05 compared with haloperidol-treated group. One-way ANOVA followed by Dunnett’s test. HPL: Haloperidol, EEMK: alcohol extract of Murraya koenigii leaves, AMK: alkaloids isolated from EEMK.
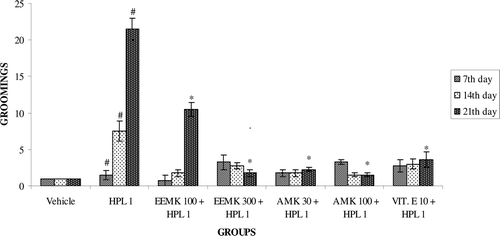
Effect of M. koenigii on body weight
Haloperidol treatment exhibited significant increase in body weight compared with vehicle-treated animals. Treatment with EEMK (100 and 300 mg/kg) in haloperidol-treated animals significantly restored the body weight compared with the haloperidol-treated group ().
Figure 6. Effect of Murraya koenigii on body weight in haloperidol-treated rats. n = 5. The observations are mean ± SEM. # p < 0.05 compared with vehicle-treated group. * p < 0.05 compared with haloperidol-treated group. One-way ANOVA followed by Dunnett’s test. HPL: Haloperidol, EEMK: alcohol extract of Murraya koenigii leaves, AMK: alkaloids isolated from EEMK.
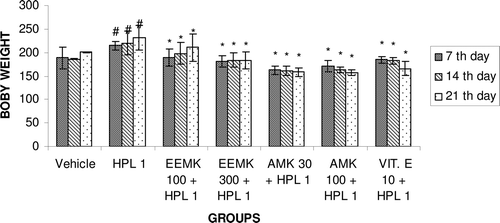
Biochemical changes after chronic haloperidol or M. koenigii treatment in rats
Effect of M. koenigii on SOD
Haloperidol-treated rats showed decreased levels of antioxidant enzyme, SOD, in forebrain homogenates. Administration of EEMK (100 and 300 mg/kg) and AMK (30 and 100 mg/kg) significantly reversed the haloperidol-induced decrease in forebrain SOD levels compared with haloperidol-treated rats ().
Table 1. Effect of Murraya koenigii on biochemical parameters in haloperidol-treated rats.
Effect of M. koenigii on CAT
Haloperidol-treated rats showed decreased levels of antioxidant enzymes CAT in forebrain homogenates. Administration of EEMK (100 and 300 mg/kg) and AMK (30 and 100 mg/kg) significantly reversed the haloperidol-induced decrease in forebrain CAT levels compared with haloperidol-treated rats ().
Effect of M. koenigii on LPO
Haloperidol treatment for 21 days induced LPO as indicated by significant raise in forebrain MDA levels compared with control rats. Administration of EEMK (100 and 300 mg/kg) and AMK (30 and 100 mg/kg) along with haloperidol significantly reversed the extent of LPO compared with haloperidol-treated rats ().
Effect of M. koenigii on GSH
Administration of haloperidol significantly decreased the forebrain GSH levels. Administration of EEMK (100 and 300 mg/kg) and AMK (30 and 100 mg/kg) significantly reversed the haloperidol-induced decrease in the forebrain GSH level compared with haloperidol-treated rats ().
Discussion
In the present study, chronic haloperidol-treated animals showed increased frequencies of VCMs and TPs compared with vehicle-treated control animals. Chronic treatment with EEMK and AMK significantly reversed the induced VCMs and TPs.
Numerous reports indicate that an excessive production of free radicals is associated with chronic neuroleptic use and might contribute to the onset of TD and other movement disorders, such as dystonia and Parkinson’s disease (CitationCadet et al., 1986). This effect may be related to a reduction in specific endogenous antioxidant mechanisms, such as a decrease in GSH levels (CitationShivakumar & Ravindranath, 1993) and low levels of antioxidant defense enzymes such as SOD and CAT (CitationElkashef & Wyatt, 1999).
The molecular mechanisms by which neuroleptics increase oxygen free radical production are unknown. Neuroleptics act by blocking dopamine receptors (CitationCreese et al., 1976). Such blockade results in increased dopamine turnover, which in turn could conceivably lead to an increased production of hydrogen peroxide, resulting in oxidative stress (CitationCohen & Spina, 1988; CitationElkashef & Wyatt, 1999). Oxygen free radicals are also reported to diminish the dopamine. However, this does not seem to be the only mechanism responsible for the GSH/ATP depletion observed during haloperidol treatment (CitationVairetti et al., 1999). CitationSagara (1998) showed that haloperidol causes a sequence of cellular alterations that leads to cell death, and the production of reactive oxygen species (from the mitochondria but not from the metabolism of catecholamines) is an integral part of this cascade. Thus, the possibility of a direct interaction with specific membrane components exists. CitationCohen and Zubenko (1985) demonstrated that striatal cell membranes of rats chronically treated with neuroleptics exhibit abnormal physicochemical properties. It is conceivable that the changes in membrane properties may be related to free radical production. Another possibility is that neuroleptics suppress the activity of certain detoxifying enzymes, leaving cells unprotected especially if basal enzyme activity is low or the free radical scavenging mechanisms are less effective. Free radicals are highly reactive with specific cellular components and have cytotoxic properties (CitationRavindranath & Reed, 1990), and neuronal loss in the striatum has been reported in animals treated chronically with neuroleptics (CitationNielsen & Lyon, 1978).
Neuroleptics may also have a direct cytotoxic effect via the production of toxic metabolites (CitationGorrod & Fang, 1993; CitationWright et al., 1998). CitationGalili et al. (2000) reported that the direct neurotoxic effects of haloperidol and its metabolites on mouse neuronal cultures and PC-12 cells were reversed by antioxidants. CitationBurkhard et al. (1993) reported that, like MPP+, the metabolites of haloperidol, chlorpromazine, and thioxanthine inhibited Complex I of the electron transport chain; clozapine was also found to inhibit Complex I, but at a much higher concentrations—this might be one of the possible mechanisms for the development of TD.
In the present study, chronic haloperidol-treated animals showed low levels of detoxifying enzymes such as SOD and CAT compared with vehicle-treated control animals, suggesting a possible induction of free radical generation by chronic haloperidol treatment. Chronic haloperidol-treated animals showed decreased levels of GSH and increased levels of LPO products compared with vehicle-treated control animals. EEMK and AMK induced the production of SOD and CAT, decreased the elevated level of LPO products in haloperidol-treated animals, also elevated the cellular defense mechanisms such as GSH, and also further suggesting the role of free radical in the pathophysiology of haloperidol-induced OD and possible antioxidant action of M. koenigii.
The mechanism by which M. koenigii prevents haloperidol-induced oxidative damage and OD is unclear. M. koenigii has been reported to activate antioxidant defensive enzymes, including increasing levels of SOD, GSH reductase, and CAT (CitationSathaye et al., 2011; CitationYankuzo et al., 2011). Another reported mechanism of M. koenigii action involves free radical scavenging. M. koenigii is a highly effective OH− scavenger (CitationRao et al., 2007). Accordingly, one or several of these actions of M. koenigii may contribute to the suppression of haloperidol-induced oxidative injury and OD.
Conclusion
The findings of this study strongly suggest that free radical toxicity and oxidative stress play an important role in the pathogenesis of OD, and that M. koenigii could be used for the prevention or treatment of neuroleptic-induced OD.
Declaration of interest
The authors report no conflict of interest.
References
- Andreassen OA, Jørgensen HA. (2000). Neurotoxicity associated with neuroleptic-induced oral dyskinesias in rats. Implications for tardive dyskinesia? Prog Neurobiol, 61, 525–541.
- Balijepalli S, Kenchappa RS, Boyd MR, Ravindranath V. (2001). Protein thiol oxidation by haloperidol results in inhibition of mitochondrial complex I in brain regions: Comparison with atypical antipsychotics. Neurochem Int, 38, 425–435.
- Beers RF Jr, Sizer IW. (1952). A spectrophotometric method for measuring the breakdown of hydrogen peroxide by catalase. J Biol Chem, 195, 133–140.
- Burkhardt C, Kelly JP, Lim YH, Filley CM, Parker WD Jr. (1993). Neuroleptic medications inhibit complex I of the electron transport chain. Ann Neurol, 33, 512–517.
- Cadet JL, Lohr JB, Jeste DV. (1986). Free radicals and tardive dyskinesia. Trends Neurosci, 9, 107–08.
- Cadet JL, Lohr JB, Jeste DV. (1987). Tardive dyskinesia and schizophrenic burnout: The possible involvement of cytotoxic free radicals. In: Henn FA & DeLisi LE, ed. Handbook of Schizophrenia: The Neurochemistry & Pharmacology of Schizophrenia. Amsterdam: Elsevier, 425–38.
- Cordell GA. (1981). Introduction to the Alkaloids: A Biogenetic Approach.New York: Wiley Interscience.
- Casey DE. (2000). Tardive dyskinesia: Pathophysiology and animal models. J Clin Psychiatry, 61 Suppl 4, 5–9.
- Cohen G, Spina MB. (1988). Hydrogen production in neurons: Implications for understanding Parkinson’s disease. In: Hefti F, Weiner WJ, editors. Progress in Parkinson’s Research. New York: Plenum, 119–126.
- Cohen BM, Zubenko GS. (1985). In vivo effects of psychotropic agents on the physical properties of cell membranes in the rat brain. Psychopharmacology (Berl), 86, 365–368.
- Coyle JT, Puttfarcken P. (1993). Oxidative stress, glutamate, and neurodegenerative disorders. Science, 262, 689–695.
- Creese I, Burt DR, Snyder SH. (1976). Dopamine receptor binding predicts clinical and pharmacological potencies of antischizophrenic drugs. Science, 192, 481–483.
- Egan MF, Apud J, Wyatt RJ. (1997). Treatment of tardive dyskinesia. Schizophr Bull, 23, 583–609.
- Elkashef AM, Wyatt RJ. (1999). Tardive dyskinesia: Possible involvement of free radicals and treatment with vitamin E. Schizophr Bull, 25, 731–740.
- Ellman GL. (1959). Tissue sulfhydryl groups. Arch Biochem Biophys, 82, 70–77.
- Galili R, Mosberg, Gil-Ad I, Weizman A, Melamed E, Offen D. (2000). Haloperidol-induced neurotoxicity–possible implications for tardive dyskinesia. J Neural Transm, 107, 479–490.
- Gorrod JW, Fang J. (1993). On the metabolism of haloperidol. Xenobiotica, 23, 495–508.
- Gupta S, Mosnik D, Black DW, Berry S, Masand PS. (1999). Tardive dyskinesia: Review of treatments past, present, and future. Ann Clin Psychiatry, 11, 257–266.
- Kulkarni SK, Naidu PS. (2001). Tardive dyskinesia: An update. Drugs Today, 37, 97–119.
- Fell MJ, Neill JC, Marshall KM. (2004). Effects of the classical antipsychotic haloperidol and atypical antipsychotic risperidone on weight gain, the oestrous cycle and uterine weight in female rats. Eur Neuropsychopharmacol, 14, 385–392.
- Misra HP, Fridovich I. (1972). The generation of superoxide radical during the autoxidation of hemoglobin. J Biol Chem, 247, 6960–6962.
- Niehaus WG, Samuelson B. (1968). Formation of malon-dialdehyde from phospholipid arachidonate during microsomal lipid peroxidation. Eur J Pharmacol, 6, 126–130.
- Nielsen EB, Lyon M. (1978). Evidence for cell loss in corpus striatum after long-term treatment with a neuroleptic drug (flupenithixol) in rats. Psychopharmacology (Berl), 59, 85–89.
- Rao LJM, Ramalakshmi K. (2007). Antioxidant and radical scavenging carbazole alkaloids from the oleoresin of curry leaves (Murraya koenigii Spreng). Food Chem, 100, 742–747.
- Ravindranath V, Reed DJ. (1990). Glutathione depletion and formation of glutathione-protein mixed disulfide following exposure of brain mitochondria to oxidative stress. Biochem Biophys Res Commun, 169, 1075–1079.
- Richardson MA, Haugland G, Pass R, Craig TJ. (1986). The prevalence of tardive dyskinesia in a mentally retarded population. Psychopharmacol Bull, 22, 243–249.
- Sathaye S, Bagul Y, Gupta S, Kaur H, Redkar R. (2011). Hepatoprotective effects of aqueous leaf extract and crude isolates of Murraya koenigii against in vitro ethanol-induced hepatotoxicity model. Exp Toxicol Pathol, 63, 587–591.
- Sagara Y. (1998). Induction of reactive oxygen species in neurons by haloperidol. J Neurochem, 71, 1002–1012.
- Saggu H, Cooksey J, Dexter D, Wells FR, Lees A, Jenner P, Marsden CD. (1989). A selective increase in particulate superoxide dismutase activity in parkinsonian substantia nigra. J Neurochem, 53, 692–697.
- Salamone JD, Lalies MD, Channell SL, Iversen SD. (1986). Behavioural and pharmacological characterization of the mouth movements induced by muscarinic agonists in the rat. Psychopharmacology (Berl), 88, 467–471.
- Shivakumar BR, Ravindranath V. (1993). Oxidative stress and thiol modification induced by chronic administration of haloperidol. J Pharmacol Exp Ther, 265, 1137–1141.
- Soares KV, Mcgrath JJ. (2002). Vitamin E for neuroleptic-induced tardive dyskinesia. Cochrane Database Syst Rev, 2.
- Vairetti M, Feletti F, Battaglia A, Pamparana F, Canonico PL, Richelmi P, Bertè F. (1999). Haloperidol-induced changes in glutathione and energy metabolism: Effect of nicergoline. Eur J Pharmacol, 367, 67–72.
- Vogel HG, Vogel WH. (2002). Drug Discovery & Evaluation. 2nd edition, New York, NY: Springer, 643.
- Wright AM, Bempong J, Kirby ML, Barlow RL, Bloomquist JR. (1998). Effects of haloperidol metabolites on neurotransmitter uptake and release: Possible role in neurotoxicity and tardive dyskinesia. Brain Res, 788, 215–222.
- Yankuzo H, Ahmed QU, Santosa RI, Akter SF, Talib NA. (2011). Beneficial effect of the leaves of Murraya koenigii (Linn.) Spreng (Rutaceae) on diabetes-induced renal damage in vivo. J Ethnopharmacol, 135, 88–94.
- Zheng W, Wang SY. (2001). Antioxidant activity and phenolic compounds in selected herbs. J Agric Food Chem, 49, 5165–5170.