Abstract
Context: Eugenol is known for its analgesic, local anesthetic, anti-inflammatory, antibacterial, and hair growing effects, the application of which, however, is limited by its low solubility, liability of sublimating, and its pungent smell. Compared to eugenol, its glycosylated derivate [eugenol α-glucoside (α-EG)] has more advantages in application.
Objective: The biosynthesis of α-EG by Xanthomonas maltophilia Hugh (Xanthomonadaceae) BT-112 and the optimum conditions for α-EG production are investigated here.
Materials and methods: The α-EG was obtained by fermentation using Xanthomonas maltophilia BT-112 and purified by macroporous absorption resin. The identity of α-EG is confirmed by high performance liquid chromatography (HPLC) and nuclear magnetic resonance (NMR).
Results: The maximum yield of α-EG reached 10.62 g/L broth when the suspension of Xanthomonas maltophilia strain was incubated at 30°C with 70 mM eugenol and 1.0 M maltose.
Discussion and conclusion: Bio-fermentation was applied in this work to get α-EG with a high mole conversion, which is a potentially efficient and highly promising approach to modify phenolic compounds into glucosides.
Introduction
Eugenol (2-allyl-4-methoxyphenol), the principal chemical component of clove oil from Eugenia caryophyllata Thunb. (Myrtaceae), has been well known for its analgesic, local anesthetic, anti-inflammatory, antibacterial, and hair growing effects (CitationHamada & Ikemoto, 2001). It belongs to the class of essential oil that is generally recognized as safe (GRAS) by Food and Drug Administration (CitationFujisawa et al., 2002; CitationHemaiswarya & Doble, 2009). Owing to numerous pharmacological benefits of eugenol, application of eugenol and its derivatives have been increasingly reported. Eugenol was found efficient in inhibition of 5-lipoxygenase (5-LO) enzyme activity and TEI (tongue edema index) (CitationDip et al., 2004; CitationRaghavenra et al., 2006). The application of eugenol in therapy, cosmetics, and research, however, was limited by its low solubility, liability of sublimating, and its pungent smell. It is, therefore, crucial to have the structure of eugenol modified.
Glucosylation has been considered to be an important method for the structural modification of phenolic compounds that contain a hydroquinone moiety (CitationHiroyuki et al., 1996, Citation2002). It can improve the biological activities of the phenolic compounds, since it increases water solubility and ameliorates pharmacological properties, and even endows the phenolic glucosides with new biological functions (CitationKurosu et al., 2002; CitationChen, 2006 a,Citationb). Compared with eugenol, for the reason, it would be useful if a eugenol glucoside could be synthesized using a simple method.
In our research for production of some polysaccharides via microbial fermentation, Xanthomonas maltophilia Hugh (Xanthomonadaceae) was occasionally found to modify phenolic compounds by glycosylation. And then, X. maltophilia BT-112 strain was successfully gained by screening and mutation to realize the glucosylation of hydroquinone and get α-arbutin as described before (CitationLiu et al., 2006). It also seemed to be practicable and meaningful to transform eugenol to eugenol α-glucoside (α-EG) by using this strain.
In this work, we developed the α-anomer-selective glucosylation of eugenol by fermentation using X. maltophilia BT-112 strain. This enzyme-catalyzed reaction was conducted regioselectively and stereoselectively without laborious protection and deprotection processes. The method described herein is efficient, simple, and selective and can be easier to be applied in industrial production.
Materials and methods
Chemicals
Eugenol and maltose of analytical reagent were purchased from Sigma Chemicals Co. (Shanghai, China). All other chemicals used were of chemically pure grades and purchased from Sinopharm Chemical Reagent Co., Ltd (Beijing, China).
Strain and medium
The strain we used was Xanthomonas maltophilia BT-112, which was described before (CitationLiu et al., 2006). The medium contained 20 g maltose, 6 g peptone, 3 g yeast extract, 0.5 g MgSO4, 2 g K2HPO4·3H2O, 0.05 g NaHCO3 per liter of distilled water. The initial pH was adjusted to 8.0.
Growth curve of bacteria
The BT-112 was incubated in the culture medium above for 48 h at 30°C with the initial density of 107 c.f.u./mL. During this process, the plate assay was performed through extraction of culture every 2 h and calculated the colony forming units (c.f.u.) later. Thus the bacterial growth curves were made and the maximum concentration of bacteria was determined.
Biosynthesis of α-EG
Cells of X. maltophilia BT-112 were grown in flask under aerobic conditions with shaking in a DHZ-25A incubator shaker (TaiCang, China) at 30°C and 150 rpm for 36 h. Then maltose and eugenol were added to the broth, with the amount up to 1 M and 70 mM, respectively, and reacted for 72 h. The reaction formula was shown in . The 1 mL of the reaction mixture was diluted with 3 mL methanol and then centrifuged at 6000 rpm for 20 min. The supernatant was analyzed by high performance liquid chromatography (HPLC).
Identification of α-EG
The amount of α-EG was measured by HPLC using a Shimadzu LC-10ATVP system with UV-VIS detector at 280 nm under the following conditions: column, YWG-C18 (4.6 × 250 mm); solvent, methanol-water (6:4, v/v); flow rate, 1 mL/min; and temperature 40°C. To confirm the product, nuclear magnetic resonance (NMR) analysis was carried out. The 13C-NMR, 1H-NMR data were obtained by an AV 600 spectrometer (Bruker, Germany) operated at 150 and 600 MHz, respectively, using dimethyl sulfoxide (DMSO) as an internal standard.
Conditions optimization of α-EG biosynthesis
To get a maximum α-EG production, the fermentation conditions were optimized. The impact of factors, such as temperature, shaking speed, pH, amount of eugenol added, the concentration of maltose, and the reaction time in sequence on the production of α-EG was examined. The conversion rate of eugenol was based on the following formula:
Conversion rate = (n1−n2)/n1 × 100%;
n1− the amount of eugenol added; n2− the amount of eugenol left.
The amount of eugenol was calculated by HPLC data. A series of eugenol-methanol solutions were prepared with different concentrations, namely 0.5, 1.0, 2.0, 3.0, 5.0, 7.5, and 10 mM. The amount of eugenol was then measured by HPLC under the conditions above. The concentration of eugenol and the peak area of eugenol were used as X and Y axes, respectively, to get a standard curve of eugenol and equation of linear regression. Thus, the amount of eugenol in the broth can be determined by its peak area from the standard curve.
Purification of α-EG
ethanol (400 ml) was added to 200 mL reaction mixture (v/v) to deposit xanthan gum (a polysaccharide produced by Xanthomonas). The mixture was centrifuged at 6000 rpm for 20 min. The supernatant obtained by filtration was then evaporated at 50°C for 50 min to remove ethanol and diluted with distilled water to 1000 mL. Macroporous adsorption resin has large capacities and high recovery yield for preparative separation of many biological products, such as phenols and glycoside compounds, due to its huge specific surface area and appropriate micropore volumes (CitationWang et al., 2005). The resin-based column chromatography was performed on a low-pressure glass chromatographic column filled with H-103 macroporous adsorption resin. Such resin-based column needed to be pretreated prior to use as described before. The crude extracts containing α-EG was subjected to the column at the velocity of 1 mL/min and eluted with 10% and 40% ethanol. The fractions obtained by elution with 40% ethanol were used as the sample for the further separation of pure α-EG. The crystal of α-EG formed by evaporation was dried in vacuum to get some white powder, namely, purified α-EG. The yield was calculated according to the following equation:
The purity meant a percentage of α-EG to total powder products and was assessed by analytical HPLC.
Statistical analysis
All the experiments were conducted at least three times. Results are expressed as means ± SD.
Results and discussion
Identification of α-EG
The growth curves of the BT-112 reached the maximum density approximately at 36 h, and then maltose and eugenol were added to the broth to biosynthesize α-EG.
The HPLC chromatograms of the reaction mixture were shown in . The single peak identified by HPLC was isolated. The identity of this compound was confirmed to be α-EG by 13C-NMR and 1H-NMR (Suppl. and and ). According to 1H-NMR, coupling constant of anomeric proton was 1.86 Hz, which is consistent with α-orientation at the anomeric center of d-glucose as reported previously (CitationLohith et al., 2006).
Table 1. 13C-NMR and 1H-NMR data of α-EG in DMSOa.
Conditions optimization of α-EG biosynthesis
The following factors, temperature, shaking speed, pH, the amount of eugenol provided, the maltose concentration, and reaction time, were selected to determine the optimum conditions for the reaction. The results showed in illustrated the effects of temperature on the synthesis of α-EG, and at 30°C a maximum molar conversion rate of 62.5% based on the eugenol supplied was reached. As shown in , the effects of shaking speed were determined. The conversion rate touched the top at 150 rpm, below or above which the conversion decreased. On the one hand, when the speed slowed down, mass transfer coefficient was low; on the other hand, shear force would enhance so much with the increasing of the speed that the cells would be destroyed. As shown in , the effects of pH were determined. The conversion rate came to a head at pH 8.0. Below pH 7.5 and above pH 8.5, the conversion decreased, probably due to the lower enzyme activity under these conditions. showed the impact of the amount of eugenol added. When the concentration of eugenol reached 70 mM, the theoretical amount of α-EG reached a maximum of 44.3 mM with a conversion rate of 63.4%. and showed the impact of the amount of maltose and the reaction time. When the concentration of maltose reached 1.0 M and the reaction lasted for 72 h, the highest molar conversion rate was gained.
Figure 3. Effects of reaction temperature (A), shaking speed (B), pH (C), eugenol concentration (D), reactant composition (E), and reaction time (F) on eugenol conversion.
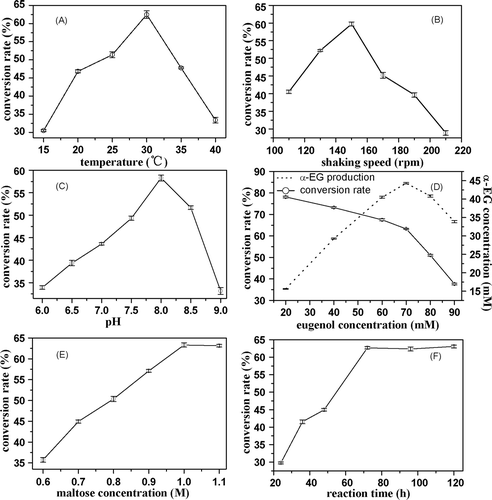
Thus, the optimum conditions for the biosynthesis of α-EG were determined as the following: maltose concentration 1.0 M; eugenol concentration 70 mM; pH 8.0; temperature 30°C; shaking speed 150 rpm, and reaction time 72 h. At 72 h, the conversion seemed to stop, and after that, the conversion rate remained constant and α-EG was not hydrolyzed.
Preparation of α-EG
A maximum molar conversion rate of 63.4% based on the amount of eugenol supplied was achieved after 72 h reaction under the optimum conditions as shown in . After purification, the α-EG yield reached 10.62 g/L according to the formula above. Since α-EG was not commercially available, purity assessment could not be made by comparison. While clear NMR spectra were obtained and a single peak was shown by HPLC analysis (). That corresponded to previously purified α-EG. Finally, the purity of α-EG was equal to 97.1%. A higher yield was obtained by a more effective and simpler method compared to the work of CitationSato et al. (2003).
In conclusion, eugenol was efficiently modified with glucosyl group by the X. maltophilia BT-112 in this research. In our previous study, we used this strain to form α-arbutin(hydroquinone α-d-glucoside) with sucrose as glucosyl donor (CitationLiu et al., 2006). While in this study, BT-112 could not catalyze the reaction between sucrose and eugenol. The mechanism still remains unknown. It was supposed that the enzyme catalyzing this reaction was a regio- and stereo-selective glycosidase which had glucose transfer activity involved in xanthan gum metabolic pathway based on our previous study (CitationLu & Xiao, 2007). Further studies on the specific enzyme and its catalytic properties on this reaction are in progress in our laboratory.
The method we used in this work was a potentially efficient and highly promising approach to modify phenolic compounds into glucosides. The results demonstrated that the reaction methodology yielded highly purified α-EG which was well suited for biochemical and pharmacological research. Although CitationSato et al. (2003) had made the similar effort to get α-EG by crude enzyme catalysis, they could hardly scale it up owing to its tiny reaction volume (2 mL) and laborious purification processes. Whereas we used free cells to catalyze the reaction by fermentation, xanthan gum as a byproduct could protect cells from eugenol toxicity, for which the enzymic reaction was characterized with high tolerance of phenolic substrate. Moreover, it would be feasible to scale-up this method which may be practical for industrial applications in future.
Acknowledgment
Chang Chen and Min Xiao contributed equally to this work.
Declaration of interest
The authors have declared no conflict of interest. This work was supported by State Key Laboratory of Microbial Technology of China.
References
- Chen C, Shi Y, Li S, Qi Q, Gu L, Song J, Wang PG. (2006). A glycosylated nitric oxide donor, beta-Gal-NONOate, and its site-specific antitumor activity. Arch Pharm (Weinheim), 339, 366–371.
- Chen C, Shi YQ, Song J, Qi QS, Gu L, Wang PG. (2006). Delivery of nitric oxide released from beta-Gal-NONOate activation by beta-galactosidase and its activity against Escherichia coli. Biol Pharm Bull, 29, 1239–1241.
- Dip EC, Pereira NA, Fernandes PD. (2004). Ability of eugenol to reduce tongue edema induced by Dieffenbachia picta Schott in mice. Toxicon, 43, 729–735.
- Fujisawa S, Atsumi T, Kadoma Y, Sakagami H. (2002). Antioxidant and prooxidant action of eugenol-related compounds and their cytotoxicity. Toxicology, 177, 39–54.
- Hamada K, Ikemoto T. (2001). Effects of clove bud oil related eugenyl glucoside on hair growth. Fragrance J, 29, 47–52.
- Hemaiswarya S, Doble M. (2009). Synergistic interaction of eugenol with antibiotics against Gram negative bacteria. Phytomedicine, 16, 997–1005.
- Hiroyuki D, Toshiyuki S, Kohtaro K. (2002). Enzymatic synthesis of l-menthyl alpha-maltoside and l-menthyl alpha-maltooligosides from l-menthyl alpha-glucoside by cyclodextrin glucanotransferase. J Biosci Bioeng, 94, 119–123.
- Hiroyuki N, Masaaki Y, Susumu S. (1996). Anomer selective formation of l-menthyl α-d-glucopyranoside by α-glucosidase-catalyzed reaction. Biosci Biotech Biochem, 60, 1914–15.
- Kurosu J, Sato T, Yoshida K, Tsugane T, Shimura S, Kirimura K, Kino K, Usami S. (2002). Enzymatic synthesis of alpha-arbutin by alpha-anomer-selective glucosylation of hydroquinone using lyophilized cells of Xanthomonas campestris WU-9701. J Biosci Bioeng, 93, 328–330.
- Liu CQ, Zhang SR, Zhang P. (2006). Biocatalytic synthesis of alpha-arbutin by Xanthomonas BT-112. Chinese J Catal, 27, 361–364.
- Lohith K, Vijayakumar GR, Somashekar BR, Sivakumar R, Divakar S. (2006). Glycosides and amino acyl esters of carbohydrates as potent inhibitors of angiotensin converting enzyme. Eur J Med Chem, 41, 1059–1072.
- Lu LL, Xiao M. (2007). Enzymes in glucosylation. Chem Life, 27, 45–48.
- Raghavenra H, Diwakr BT, Lokesh BR, Naidu KA. (2006). Eugenol–the active principle from cloves inhibits 5-lipoxygenase activity and leukotriene-C4 in human PMNL cells. Prostaglandins Leukot Essent Fatty Acids, 74, 23–27.
- Sato T, Takeuchi H, Takahashi K, Kurosu J, Yoshida K, Tsugane T, Shimura S, King K, Kirimura K. (2003). Selective alpha-glucosylation of eugenol by alpha-glucosyl transfer enzyme of Xanthomonas campestris WU-9701. J Biosci Bioeng, 96, 199–202.
- Wang X, Li F, Sun Q, Yuan J, Jiang T, Zheng C. (2005). Application of preparative high-speed counter-current chromatography for separation and purification of arctiin from Fructus Arctii. J Chromatogr A, 1063, 247–251.