Abstract
Context: Cecropia glaziovii Snethl. (Cecropiaceae), commonly known as “embaúba-vermelha”, is widely distributed throughout Latin America and has been reported in Brazilian folk medicine to treat cough, asthma, high blood pressure and inflammation.
Objective: Investigate the hepatoprotective properties of crude hydroethanolic extract of C. glaziovii as well as its in vitro antioxidant and antiviral (HSV-1 acyclovir resistant strain) activities.
Materials and methods: The hepatoprotective effect, the antioxidant properties and antiviral activity of crude hydroethanol extract (RCE40) from C. glaziovii leaves were evaluated by carbon-tetrachloride (CCl4)-induced hepatotoxicity, by TBARS (thiobarbituric acid reactive species) and MTT [3-(4,5-dimethylthiazol-2-yl)-2,5-diphenyl tetrazolium bromide] assays, respectively.
Results: The RCE40 extract (20 mg/kg) inhibited lipid peroxidation on liver in post injury treatment and decreased serum levels of alanine aminotransferase (ALT) and aspartate aminotransferase (AST). In addition, in this protocol the RCE40 (20 mg/kg) enhanced the activity of hepatic enzymes (SOD/CAT) which are involved in combating reactive oxygen species (ROS), suggesting that it possesses the capacity to attenuate the CCl4-induced liver damage. Moreover the RCE40 (20 mg/kg) inhibited TBARS formation induced by several different inductors of oxidative stress showing significant antioxidant activity, including physiologically relevant concentration, as low as 2 µg/mL. Concerning antiviral activity, the RCE40 was effective against herpes simplex virus type 1 replication (29R acyclovir resistant strain) with EC50 = 40 µg/mL and selective index (SI) = 50.
Discussion and conclusion: These results indicate that C. glaziovii could be a good source of antioxidant and anti-HSV-1 lead compounds.
Introduction
Cecropia glaziovii Snethl. (Cecropiaceae), commonly known as “embaúba-vermelha”, is widely distributed throughout Latin America and in the Southeast and South of Brazil (CitationBerg & Rosseli, 2005). It has been reported in Brazilian folk medicine to treat cough, asthma, high blood pressure, inflammation, and is used as a diuretic (CitationLorenzi & Matos, 2008; CitationPio Correa, 1978).
Several biological effects were described in the literature for C. glaziovii such as antihypertensive (CitationLima-Landmann et al., 2007; CitationNinahuaman et al., 2007), anxiolytic and antidepressant (CitationRocha et al., 2002; Citation2007), bronchodilator (CitationDelarcina et al., 2007), antiacid secretion and antiulcer (CitationSouccar et al., 2008).
Concerning their chemical composition, previous studies have reported phenolic compounds such as C-glycosylflavonoids, proanthocyanidins and phenolic acids in Cecropia species (CitationAndrade-Cetto & Wiedenfeld, 2001; CitationTanae et al., 2007; CitationCosta et al., 2011). These polyphenolic compounds are well known as inhibitors of herpes simplex virus type 1 (HSV-1) replication (CitationFortin et al., 2002; CitationBettega et al., 2004), antibacterial agents (CitationEsquenazi et al., 2002) and as free radical scavengers and inhibitors of lipid peroxidation (CitationRice-Evans et al., 1996; CitationDe Oliveira et al., 2003).
As far as we are aware, there are no reports in the literature investigating the hepatoprotective effects and antioxidant activity of C. glaziovii with the exception of one anti HSV-1 research for this species (CitationSilva et al., 2010). Thus, the aim of this study was to investigate the hepatoprotective properties of crude hydroethanolic extract of C. glaziovii as well as its in vitro antioxidant and antiviral (HSV-1 acyclovir resistant strain) activities.
Materials and methods
Plant material
The leaves of C. glaziovii Snethl. (Cecropiaceae) were collected in Criciúma, State of Santa Catarina, Brazil in August 2003. The plant material was identified by Dr. Vanilde C. Zanette and a voucher specimen (CRI 7371) was deposited in the Herbarium Pe Dr. Raulino Reitz (Universidade do Extremo Sul Catarinense).
Preparation of the extracts
Air-dried leaves (50 g) of C. glaziovii were crushed and extracted under reflux (90°C) with 500 ml of ethanol 40° GL (Gay-Lussac - alcohol concentration unit) for 30 min. After cooling, the extract was filtered and then evaporated under reduced pressure to dryness yielding the crude hydroethanolic extract (RCE40).
Chemical characterization of the RCE40 extract by high-performance liquid chromatography (HPLC)
The qualitative analysis of phenolic compounds was performed on methodology previously described by CitationCosta et al. (2011). Briefly, a PerkinElmer Brownlee Choice C18 column (150 × 4.6 mm i.d.; 5 mm) was employed and a gradient combining solvent A (acetonitrile) and solvent B (acetic acid 1%, adjusted to pH 3.0) as follows: 0–30 min, linear change from A–B (5:95 v/v) to A–B (20:80 v/v); 30–40 min, isocratic A–B (20:80 v/v), with a flow rate of 1.0 mL/min, detection at 340 nm and UV spectra monitored over a range of 450–200 nm. The injection volume was 10 µL and the peaks were characterized by comparing the retention time, UV spectra and by the co-injection of the sample with the reference standards.
Antioxidant activity
In vitro assay
The antioxidant activity of C. glaziovii crude extract (RCE40) was estimated by the inhibition of thiobarbituric acid reactive substances (TBARS) formation induced by three different free radical generators in a lipid rich substrate (CitationPolydoro et al., 2004). Briefly, 500 µl of 0.1% (w/v) lipid homogenate in phosphate buffer (pH 7.4) was mixed with 500 µl of trichloroacetic acid (10%) and centrifuged at 1,200g (10 min). The RCE40 extract at different concentrations (2, 20, 200 µg/mL) was added to a test tube together with 2,2-azobis (2-amidino-propane) dichloride (AAPH-0.5 M), iron sulfate (FeSO4-0.145 mM) or hydrogen peroxide (H2O2-0.4 M) solutions to induce lipid peroxidation. The tubes were incubated at room temperature for 15 min and 500 µL of thiobarbituric acid (TBA-0.67%) was added and heated at 100°C for 30 min. After cooling, the absorbances were measured by a spectrophotometer (Micronal®) at 532 nm, and the results were expressed as nmol malondialdehyde equivalents (MDA)/mL of substrate. Controls were run simultaneously.
In vivo assay
Animals.
Male Wistar rats (250–350 g, 2–3 months old) from our own breeding colony were housed in five per cage under 12-h light/dark cycle (lights on 7:00 a.m.) at constant temperature of 23° ± 1°C. Animals (n = 60) were maintained with food (Nuvilab® chow) and water ad libitum. In vivo studies were performed in accordance with the National Institutes of Health guidelines and with the approval of the local ethics committee.
Treatment and liver injury induction.
In the post injury protocol an acute liver injury was induced by an i.p. administration of CCl4 (5 mg/kg, dissolved in soy oil 1:1, v/v %) as previously described (CitationLee et al., 2003). The animals were divided into five groups, in six animals per group: 1) control (ethanol 1% p.o.); 2) CCl4 (5 mg/kg i.p.); 3) CCl4 + RCE40 (20 mg/kg in ethanol 1%, p.o.); 4) CCl4 + RCE40 (40 mg/kg in ethanol 1%, p.o.); 5) CCl4 + NAC (N-acetylcysteine) (100 mg/kg i.p.). All treatments were administered for 3 h after CCl4 administration and continued every 12 h for 2 consecutive days. Plasma obtained from the tail vein was collected from each rat, 12 h after CCl4 administration for the determination of enzymes ALT and AST. 12 h after, rats were killed by decapitation, the liver was isolated and fixed in a 4% formalin solution for histological analyses or immediately stored at −70°C until TBARS assay. In the prophylactic protocol (CitationIlavarasan et al., 2003) rats were divided into five groups as described above. In this protocol all groups were treated once, during seven days, and on the seventh day, CCl4 (5 mg/kg, dissolved in soy oil 1:1, v/v % i.p.) was administered except in the group 1. Plasma obtained from the tail vein was collected from each rat, 6 h after CCl4 administration for the determination of serum ALT and AST. After 24 h, rats were killed by decapitation, the liver isolated and fixed in a 4% formalin solution for histological analyses or immediately stored at −70°C until TBARS assay.
Determination of hepatic lipid peroxidation.
TBARS in liver homogenate was determined by reaction with TBA and by an index of lipid peroxidation (CitationDraper & Hadley 1990). Briefly, the samples were mixed with 1 ml of trichloroacetic acid 10% and 1 ml of TBA 0.67%; this mixture was then heated in a boiling water bath for 30 min and the malondialdehyde (MDA) equivalents were determined by the absorbance at 532 nm using 1,1,3,3-tetramethoxypropane as an external standard. The results were expressed as nmol MDA equivalents per milligram of protein (nmol/mg) (CitationLowry et al., 1951).
Antioxidant enzyme activities.
Superoxide dismutase (SOD) activity was measured spectrophotometrically by the inhibition rate of autocatalytic adrenochrome formation in a reaction buffer containing 1 mM adrenaline/50 mM glycine-NaOH (pH = 10.2) as previously described (CitationBannister & Calaberese 1987). The method of CitationAebi (1984) was used to analyse catalase (CAT) activity. Homogenates were sonicated in 50 mM phosphate buffer (pH 7.0) and the resulting suspension was centrifuged at 3000 × g for 10 min. The supernatant was used for enzyme assay. CAT activity was assayed by measuring the rate of decrease in H2O2 absorbance at 240 nm. Enzyme activities were reported as units/mg of protein.
Determination of serum biochemical parameters.
Serum alanine aminotransferase (ALT) and aspartate aminotransferase (AST) levels were determined routinely by commercial available kits (Labtest, Brazil).
Histological examination of liver.
For histopathological analyses after fixation, excised liver tissues were soaked in paraffin and then routinely stained with hematoxylin and eosin. A blinded experienced pathologist performed histopathological analyses.
Antiviral assay and
cytotoxicity evaluation–Cell viability test. The cytotoxicity evaluation was performed by MTT [3-(4,5-dimethylthiazol-2-yl)-2,5-diphenyl tetrazolium bromide] method, according to CitationTakeuchi et al. (1991) and CitationSieuwerts et al. (1995) with minor modifications. Briefly, VERO cell cultures (2 × 105 cells/mL) were prepared in 96-well tissue culture plates (Corning®). After a 24 h period of incubation at 37°C under a humidified 5% CO2 atmosphere, the cell monolayers were confluent, the medium was removed from the wells, and 200 μL of each extract/fraction dilutions (1:2 – ranging from 2000 to 15.6 μg/mL prepared in cell culture medium) was added to each well. As a cell control, only 200 μL of medium was added to the cells. The plates were incubated under the same conditions cited above. After 4 days, the medium was removed by suction from all wells and 50 μL of MTT (Sigma®, 1 mg/mL) solution prepared in cell culture medium was added to each well and the plates were incubated once more for 4 h. After the MTT solution was removed without disturbing the cells, 100 μL of DMSO was added to each well to dissolve the formazan crystals. After gently shaking the plates, the crystals were completely dissolved, and the absorbances were read on a multiwell spectrophotometer (Bio-Tek®, Elx 800) at 540 nm. The CC50 was defined as the cytotoxic concentration of sample that reduced the absorbance of treated cells to 50% when compared with that of the cell control.
Antiherpes assay. VERO cell cultures (2 × 105 cells/mL) were prepared in the same way as described above and, when the cell monolayers were confluent, the medium was removed from the wells and 100 μL/well of non-cytotoxic concentrations (≤ CC50 values) of the extracts and 100 μL/well of HSV-1 (29-R acyclovir resistant strain – University of Rennes, France) at 0.5 of multiplicity of infection (MOI) were added simultaneously to the cells. Cell and viral controls were performed by adding only 200 μL of minimum essential media (MEM) or 200 μL of viral suspension, respectively. The plates were incubated for 96 h. The same MTT method used to evaluate cell viability was followed. The percentages of protection were calculated as [(A−B) × 100/(C−B)], where A, B and C indicate the absorbances of the extracts, virus and cell controls, respectively. Each obtained EC50 value was defined as the effective concentration that reduced the absorbance of infected cells to 50% when compared with cell and virus controls. The selective index was calculated as CC50/EC50.
Statistical analysis. For antioxidant activity, the results were expressed as means ± S.D. and p values were considered significant when p < 0.05. Differences in experimental groups were determined by ANOVA. Comparison between means was carried out using a Newman–Keuls test. For antiviral activity the 50% cytotoxic (CC50) and 50% effective (EC50) concentrations were calculated from concentration-effect curves after linear regression analysis. The results represent the mean ± standard error (S.E.) of the mean values of three different experiments.
Results and discussion
The antioxidant properties, hepatoprotective effect and antiviral activity of crude hydroethanol extract (RCE40) from C. glaziovii leaves were evaluated, respectively, by using TBARS, carbon-tetrachloride (CCl4) induced hepatotoxicity and MTT assays.
Antioxidant activity
In vitro assay
The in vitro antioxidant activity assay employed three different oxidant systems to induce TBARS formation [AAPH (0.5 M), FeSO4 (0.145 mM) or H2O2 (0.4 M) solutions]. The extract (RCE40) in all concentrations tested (2, 20 and 200 µg/mL, respectively) inhibited TBARS formation induced by all inductors of oxidative stress, showing a significant antioxidant activity, including physiologically relevant concentration (as low as 2 µg/mL) (). In addition, these results showed that RCE40 could act as a lipid peroxidation chain breaker, direct scavengers of peroxyl/hydroxyl/hydrogen peroxide and as an iron chelator.
Table 1. Inhibition of lipid peroxidation by hydroethanolic extracts from C. glaziovii leaves induced by H2O2, FeSO4, AAPH.
The most valuable exogenous reactive oxygen species (ROS) generator in biological systems is H2O2. In particular, H2O2 is a potential source for hydroxyl radical (·OH), one of the most dangerous radicals formed through the Fenton reaction in the presence of transition metal ions, as iron. Additionally, azo-initiators like AAPH induce the formation of peroxyl radicals, capable of initiating the chain reaction of lipid peroxidation (CitationCao et al., 1997; CitationMartini & Termini 1997; CitationKanno et al., 2003).
In vivo assay
In this work, we showed the effect of RCE40 on hepatic oxidative damage through four parameters: lipid peroxidation (TBARS), hepatic antioxidant enzymes (SOD/CAT), serum levels of alanine aminotransferase (ALT) and aspartate aminotransferase (AST) activity and by the degree of inflammatory response and hepatic necrosis. These treatments with RCE40 (20 and 40 mg/kg) on CCl4-induced liver injury were compared to the effect of N-acetylcysteine (NAC) as the antioxidant standard. Additionally, we used two protocols: injury protocol (after CCl4 administration) and prophylactic protocol (before CCl4 administration).
In order to determine the antioxidant effects of RCE40, we determined TBARS levels in liver homogenate. Administration of CCl4 increased TBARS content in comparison to the control group as expected. All treatments in the post injury, inhibited lipid peroxidation on liver (). On the other hand, in the prophylactic protocol liver protection was not detected against oxidative damage induced by CCl4. In contrast RCE40 (20 mg/kg) potentiated the oxidative damage induced by CCl4 administration ().
Figure 1. (A) TBARS values in carbon tetrachloride intoxicated rat liver treated with an RCE40 extract of C. glaziovii orally and NAC intraperitoneally in two protocols. In the post injury protocol, rats were pretreated with carbon tetrachloride (CCl4) (5 mg/kg). Three hours later, rats were treated with RCE40 (20 and 40 mg/kg p.o.) or NAC (100 mg/kg i.p.) twice daily. Normal rats were given in ethanol 1%.Data are mean ± SD *Different from control, p < 0.05; **different from rats receiving CCl4 alone, p < 0.05. (B) TBARS values in carbon tetrachloride intoxicated rat liver treated with an RCE40 extract of C. glaziovii orally and NAC intraperitoneally in two protocols. In the prophylactic protocol rats were pretreated with ethanol 1%, RCE40 or NAC in the same doses that the post injury protocol, once a day, for seven days, on the seventh day, a single dose of the mixture of CCl4 (5 mg/kg) and soy oil (1:1, v/v%,5 ml/kg i.p.) was administered. Data are mean ± SD *Different from control, p < 0.05; **different from rats receiving CCl4 alone, p < 0.05.
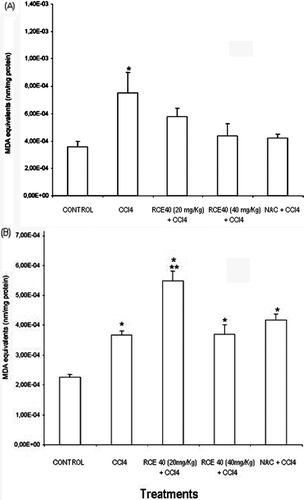
The thiobarbituric acid reactive substances (TBARS) is an indirect measurement of lipid peroxidation (CitationNan et al., 2003). Lipid peroxide levels in a liver tissue were found to be significant in CCl4-challenged rats (CitationHalliwell et al., 1995). These free radicals trigger cell damage through two mechanisms namely covalent binding to cellular macromolecules and lipid peroxidation, which affect the ionic permeability of the membrane structure (CitationBasu, 2003). The RCE40 effect in the post injury protocol may be attributed to the antioxidant activity of the extract which is exhibited by scavenging the CCl3 radical generated due to the metabolic transformation of CCl4 in the liver or by the interruption of the lipid peroxidation progression (CitationAhmed & Urooj, 2010). This protection is of similar magnitude to the effects of NAC, a well-known and potent antioxidant. The higher protection exhibited by the post injury when compared to the prophylactic protocol could be associated to different factors. Probably the antioxidant compounds do not accumulate or are metabolized to inactive, non- toxic compounds (CitationSkibola & Smith 2000; CitationGalat & O’Brien 2004), and these are in accordance with our results.
Concerning to hepatic antioxidant enzyme activities, there was a significant decrease of the SOD/CAT activity with CCl4 administration in the post injury protocols. The decrease in antioxidant enzymes activities induced by CCl4 in post injury protocols may be secondary to the inactivation caused by free radicals (Silk-Lesiuk et al., 2003). The superoxide dismutase enzyme (SOD) is considered the most effective antioxidant defense that the body has against superoxide free radicals. However, in the process of removing superoxide free radicals, SOD rarely operates alone, and requires the catalase enzyme (CAT) to remove hydrogen peroxide molecules which are by-products of the reactions created by SOD (CitationHalliwell & Gutteridge, 1999). In this protocol, RCE40 (20 and 40 mg/kg) increased the activity of antioxidant liver enzymes (), as well as increased SOD/CAT relation in the prophylactic protocols ().
The analyses of serum biochemical parameters showed that the serum levels of ALT and AST in both prophylactic and post injury protocols were significantly increased in comparison to the control, suggesting hepatocellular death in this model. In the post injury protocol, RCE40 (20 and 40 mg/kg) decreased serum levels of ALT and AST, while in the prophylactic treatment these protective effects were less pronounced and the high doses of RCE40 (40 mg/kg) increased ALT and AST plasmatic levels ().
Table 2. Effect of RCE40 extract in plasmatic ALT and AST levels in rats with liver injury induced by carbon tetrachloride.
Figure 2. (A) SOD/CAT values in carbon tetrachloride intoxicated rat liver treated with a RCE40 extract of C. glaziovii orally and NAC intraperitoneally in two protocols. In the post injury protocol, rats were pretreated with carbon tetrachloride (5 mg/kg). Three hours later, rats were treated with RCE40 (20 and 40 mg/kg p.o.) or NAC (100 mg/kg i.p.) twice daily. Normal rats were given in ethanol 1%. Data are mean ± SD *Different from control, p < 0.05; **different from rats receiving carbon tetrachloride alone, p < 0.05. (B) SOD/CAT values in carbon tetrachloride intoxicated rat liver treated with a RCE40 extract of C. glaziovii orally and NAC intraperitoneally in two protocols. In the prophylactic protocol rats were pretreated with ethanol 1%, RCE40 or NAC in the same doses that the post injury protocol, once a day, for seven days, on the seventh day, a single dose of the mixture of CCl4 and soy oil (1:1, v/v%, 5 ml/kg i.p.) was administered. Data are mean ± SD *Different from control, p < 0.05; **different from rats receivingcarbon tetrachloride alone, p < 0.05.
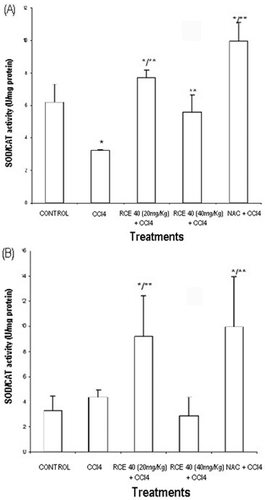
In relation to the effect of RCE40 on histological changes in the liver, we observed that liver histopathology after CCl4 administration showed lobular disarray, fatty degeneration, potent inflammatory cell infiltration, and severe necrosis of hepatocytes (). In the post injury protocol, RCE 40 mg/kg () reduced CCl4-induced necrosis and inflammation. In the prophylactic treatment the lower doses minimally reduced the damage induced by CCl4 (data not shown). In contrast, the highest dose of both extracts increased the hepatotoxicity of CCl4 in the prophylactic protocol ().
Figure 3. (A) Effects of C. glaziovii in post injury or prophylactic protocols on CCl4-induced liver damage in rats. Liver tissue sections from rat receiving CCl4. HE × 400, representative of three animals/group. (B) Effects of C. glaziovii in post injury or prophylactic protocols on CCl4-induced liver damage in rats. Liver tissue sections from rat with CCl4 and RCE40 (40 mg/kg) post injury protocol. HE × 400,representative of three animals/group. (C) Effects of C. glaziovii in post injury or prophylactic protocols on CCl4-induced liver damage in rats. Liver tissue sections from rat with CCl4 and RCE (40 mg/kg) prophylactic protocol. HE × 400, representative of three animals/group
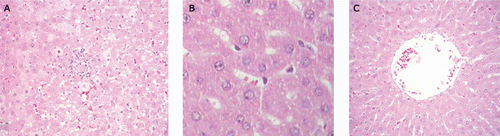
In the present study, we found that the post injury treatment with the RCE40 inhibits CCl4-induced damage as evidenced by decreased serum activities of AST and ALT, decreased hepatic lipid peroxidation, and by the histological observations. The decrease in antioxidant enzymes activities induced by CCl4 administration may be also resulting from inactivation caused by lipid peroxides or enhanced free radical concentration in oxidative stress conditions (Silk-Lesiuk et al., 2003).
The chemical analysis by HPLC-UV/DAD, RCE40 showed isovitexin (rt = 28.4 min) as the major compound. Furthermore chlorogenic acid (rt = 13.7 min), isoorientin (rt = 24.7 min) and orientin (rt = 25.3 min) () were also identified. Since the ability of plant extract to scavenge ROS and/or metal chelating seems to be related to the chemical structure of phenolic compounds (CitationRice-Evans et al., 1996; CitationHalliwell & Gutteridge, 1999), the biological effects herein described could be related to flavonoids present in the RCE40 extract, which are involved in free radical-scavenging and metal ion-chelating (Heras et al., 1998; CitationHigdon & Frei 2003; CitationKaneko et al., 2003; CitationVelázquez et al., 2003).
Antiviral activity
The RCE40 extract from the leaves of C. glaziovii showed low cytotoxicity against VERO cell cultures (CC50 > 2.000 μg/mL) and inhibited significantly the replication of HSV-1 (29R strain), (EC50 = 40 μg/mL) yielding a good selectivity index (SI = 50). Recently, our research group showed the in vitro anti HSV-1 (KOS strain–acyclovir sensitive) and HSV-2 (333 strain) of the crude extract obtained from C. glaziovii leaves and their C-glycosylflavonoid-enriched fraction (CitationSilva et al., 2010). Herein, we demonstrate that RCE40 extract is active against HSV-1 (29R strain–acyclovir resistant).
There are several reports of antiviral activity of phenolic compounds (CitationOkuda et al, 2005; CitationOrhan et al., 2010). Chlorogenic acid and its related compounds are abundant plant polyphenols that have anti HSV-1 activity (CitationChiang et al., 2002; CitationWang et al., 2009; CitationNolkemper et al., 2010). In the same way, the anti-HSV activity of naturally occurring flavonoids has also been described (CitationLyu et al, 2005; CitationSchnitzler et al., 2010). Therefore, our findings suggest that at least part of the anti HSV-1 effects of the RCE40 extract might be attributable to its phenolic composition.
Since the efficacy of acyclovir is limited by the recent increase in the resistance of virus and recurrence of latent virus, particularly from immunocompromised patients (CitationCheng et al., 2002), the data presented herein indicate that the C. glaziovii leaf extracts have HSV-1 activity against acyclovir resistant strain and should be considered as a potential new sources of antiviral agents. However, further studies to elucidate the active principles and the underlying mechanism responsible for the antiherpes effects of phenolic compounds present in this extract as well as the mechanism responsible for the protective effects are needed.
Conclusion
The results showed that C. glaziovii leaves RCE40 extract possess in vitro and in vivo capacities of inhibiting lipid peroxidation and attenuating the CCl4-induced hepatic damage respectively. This extract was also effective against herpes simplex virus type 1 replication (29R strain, acyclovir-resistant). Finally, the HPLC fingerprint of RCE40 showed the presence of isoorientin, orientin, isovitexin and chlorogenic acid as major compounds. Further studies are in progress to determine the exact mechanism associated with pharmacological activities detected, their possible synergistic effect, as well as the toxic effects observed in the prophylactic treatment group.
Acknowledgements
The authors SQ Oliveira, JCF Moreira, CMO Simões, F Dal-Pizzol and FH Reginatto are grateful to CNPq for their research fellowships.
Declaration of interest
This work was performed with financial support from CNPq, CAPES, FAPERGS and FAPESC.
References
- Aebi H. (1984). Catalase in vitro. Meth Enzymol, 105, 121–126.
- Ahmed F, Urooj A. (2010). Hepatoprotective effects of Ficus racemosa stem bark against carbon tetrachloride-induced hepatic damage in albino rats. Pharm Biol, 48, 210–216.
- Andrade-Cetto A, Wiedenfeld H. (2001). Hypoglycemic effect of Cecropia obtusifolia on streptozotocin diabetic rats. J Ethnopharmacol, 78, 145–149.
- Bannister JV, Calabrese L. (1987). Assays for superoxide dismutase. Methods Biochem Anal, 32, 279–312.
- Basu S. (2003). Carbon tetrachloride-induced lipid peroxidation: eicosanoid formation and their regulation by antioxidant nutrients. Toxicology, 189, 113–127.
- Berg CC, Roselli PF. (2005). “Cecropia” Flora Neotropica. New York, USA: The New York Botanical Garden Press.
- Bettega JM, Teixeira H, Bassani VL, Barardi CR, Simões CM. (2004). Evaluation of the antiherpetic activity of standardized extracts of Achyrocline satureioides. Phytother Res, 18, 819–823.
- Cao G, Sofic E, Prior RL. (1997). Antioxidant and prooxidant behavior of flavonoids: structure-activity relationships. Free Radic Biol Med, 22, 749–760.
- Cheng HY, Lin CC, Lin TC. (2002). Antiherpes simplex virus type 2 activity of casuarinin from the bark of Terminalia arjuna Linn. Antiviral Res, 55, 447–455.
- Chiang LC, Chiang W, Chang MY, Ng LT, Lin CC. (2002). Antiviral activity of Plantago major extracts and related compounds in vitro. Antiviral Res, 55, 53–62.
- Costa GM, Ortmann CF, Schenkel EP, Reginatto FH. (2011). An HPLC-DAD method to quantification of main phenolic compounds from leaves of Cecropia species. J Braz Chem Soc, 22, 1089–1095.
- De Oliveira SQ, Dal-Pizzol F, Gosmann G, Guillaume D, Moreira JC, Schenkel EP. (2003). Antioxidant activity of Baccharis articulata extracts: Isolation of a new compound with antioxidant activity. Free Radic Res, 37, 555–559.
- Delarcina S Jr, Lima-Landman MTR, Souccar C, Cysneiros RM, Tanae MM, Lapa AJ. (2007). Inhibition of histamine-induced bronchospasm in guinea pigs treated with Cecropia glaziovi Sneth and correlation with the in vitro activity in tracheal muscles. Phytomedicine, 14, 328–332.
- Draper HH, Hadley M. (1990). Malondialdehyde determination as index of lipid peroxidation. Meth Enzymol, 186, 421–431.
- Esquenazi D, Wigg MD, Miranda MM, Rodrigues HM, Tostes JB, Rozental S, da Silva AJ, Alviano CS. (2002). Antimicrobial and antiviral activities of polyphenolics from Cocos nucifera Linn. (Palmae) husk fiber extract. Res Microbiol, 153, 647–652.
- Fortin H, Vigor C, Lohézic-Le Dévéhat F, Robin V, Le Bossé B, Boustie J, Amoros M. (2002). In vitro antiviral activity of thirty-six plants from La Réunion Island. Fitoterapia, 73, 346–350.
- Galati G, O’Brien PJ. (2004). Potential toxicity of flavonoids and other dietary phenolics: significance for their chemopreventive and anticancer properties. Free Radic Biol Med, 37, 287–303.
- Halliwell B, Aeschbach R, Löliger J, Aruoma OI. (1995). The characterization of antioxidants. Food Chem Toxicol, 33, 601–617.
- Halliwell B, Zhao K, Whiteman M. (1999). Nitric oxide and peroxynitrite. The ugly, the uglier and the not so good: A personal view of recent controversies. Free Radic Res, 31, 651–669.
- de las Heras B, Slowing K, Benedí J, Carretero E, Ortega T, Toledo C, Bermejo P, Iglesias I, Abad MJ, Gómez-Serranillos P, Liso PA, Villar A, Chiriboga X. (1998). Antiinflammatory and antioxidant activity of plants used in traditional medicine in Ecuador. J Ethnopharmacol, 61, 161–166.
- Higdon JV, Frei B. (2003). Tea catechins and polyphenols: Health effects, metabolism, and antioxidant functions. Crit Rev Food Sci Nutr, 43, 89–143.
- Ilavarasan R, Vasudevan M, Anbazhagan S, Venkataraman S. (2003). Antioxidant activity of Thespesia populnea bark extracts against carbon tetrachloride-induced liver injury in rats. J Ethnopharmacol, 87, 227–230.
- Kaneko T, Tahara S, Baba N. (2003). Inhibition of linoleic acid hydroperoxide-induced toxicity in cultured human fibroblasts by anthocyanidins. Biosci Biotechnol Biochem, 67, 1391–1393.
- Kanno S, Shouji A, Asou K, Ishikawa M. (2003). Effects of naringin on hydrogen peroxide-induced cytotoxicity and apoptosis in P388 cells. J Pharmacol Sci, 92, 166–170.
- Lee TY, Mai LM, Wang GJ, Chiu JH, Lin YL, Lin HC. (2003). Protective mechanism of Salvia miltiorrhiza on carbon tetrachloride-induced acute hepatotoxicity in rats. J Pharmacol Sci, 91, 202–210.
- Lima-Landman MT, Borges AC, Cysneiros RM, De Lima TC, Souccar C, Lapa AJ. (2007). Antihypertensive effect of a standardized aqueous extract of Cecropia glaziovii Sneth in rats: An in vivo approach to the hypotensive mechanism. Phytomedicine, 14, 314–320.
- Lorenzi H, Thiagarajan M, Haas B, Wortman J, Hall N, Caler E. (2008). Genome wide survey, discovery and evolution of repetitive elements in three Entamoeba species. BMC Genomics, 9, 595.
- Lowry OH, Rosebrough NJ, Farr AL, Randall RJ. (1951). Protein measurement with the Folin phenol reagent. J Biol Chem, 193, 265–275.
- Lyu SY, Rhim JY, Park WB. (2005). Antiherpetic activities of flavonoids against herpes simplex virus type 1 (HSV-1) and type 2 (HSV-2) in vitro. Arch Pharm Res, 28, 1293–1301.
- Martini M, Termini J. (1997). Peroxy radical oxidation of thymidine. Chem Res Toxicol, 10, 234–241.
- Nan JX, Jiang YZ, Park EJ, Ko G, Kim YC, Sohn DH. (2003). Protective effect of Rhodiola sachalinensis extract on carbon tetrachloride-induced liver injury in rats. J Ethnopharmacol, 84, 143–148.
- Ninahuaman MFML, Souccar C, Lapa AJ, Lima-Landman MTR. (2007). ACE activity during the hypotension produced by standardized aqueous extract of Cecropia glaziovii Sneth: A comparative study to captopril effects in rats. Phytomedicine, 4, 321–327.
- Nolkemper S, Reichling J, Sensch KH, Schnitzler P. (2010). Mechanism of herpes simplex virus type 2 suppression by propolis extracts. Phytomedicine, 17, 132–138.
- Okuda T. (2005). Systematics and health effects of chemically distinct tannins in medicinal plants. Phytochemistry, 66, 2012–2031.
- Orhan DD, Ozçelik B, Ozgen S, Ergun F. (2010). Antibacterial, antifungal, and antiviral activities of some flavonoids. Microbiol Res, 165, 496–504.
- Pio Correa M. (1978). Dicionário de plantas úteis do Brasil e das plantas exóticas cultivadas. Rio de Janeiro: Imprensa Nacional.
- Polydoro M, de Souza KC, Andrades ME, Da Silva EG, Bonatto F, Heydrich J, Dal-Pizzol F, Schapoval EE, Bassani VL, Moreira JC. (2004). Antioxidant, a pro-oxidant and cytotoxic effects of Achyrocline satureioides extracts. Life Sci, 74, 2815–2826.
- Rice-Evans CA, Miller NJ, Paganga G. (1996). Structure-antioxidant activity relationships of flavonoids and phenolic acids. Free Radic Biol Med, 20, 933–956.
- Rocha FF, Lapa AJ, De Lima TC. (2002). Evaluation of the anxiolytic-like effects of Cecropia glazioui Sneth in mice. Pharmacol Biochem Behav, 71, 183–190.
- Rocha FF, Lima-Landman MT, Souccar C, Tanae MM, De Lima TC, Lapa AJ. (2007). Antidepressant-like effect of Cecropia glazioui Sneth and its constituents - in vivo and in vitro characterization of the underlying mechanism. Phytomedicine, 14, 396–402.
- Schnitzler P, Neuner A, Nolkemper S, Zundel C, Nowack H, Sensch KH, Reichling J. (2010). Antiviral activity and mode of action of propolis extracts and selected compounds. Phytother Res, 24 Suppl 1, S20–S28.
- Sieuwerts AM, Klijn JG, Peters HA, Foekens JA. (1995). The MTT tetrazolium salt assay scrutinized: how to use this assay reliably to measure metabolic activity of cell cultures in vitro for the assessment of growth characteristics, IC50-values and cell survival. Eur J Clin Chem Clin Biochem, 33, 813–823.
- Szymonik-Lesiuk S, Czechowska G, Stryjecka-Zimmer M, Slomka M, Madro A, Celinski K, Wielosz M. (2003). Catalase, superoxide dismutase, and glutathione peroxidase activities in various rat tissues after carbon tetrachloride intoxication. J Hepatobiliary Pancreat Surg, 10, 309–315.
- Silva IT, Costa GM, Stoco PH, Schenkel EP, Reginatto FH, Simões CM. (2010). In vitro antiherpes effects of a C-glycosylflavonoid-enriched fraction of Cecropia glaziovii Sneth. Lett Appl Microbiol, 51, 143–148.
- Skibola CF, Smith MT. (2000). Potential health impacts of excessive flavonoid intake. Free Radic Biol Med, 29, 375–383.
- Souccar C, Cysneiros RM, Tanae MM, Torres LM, Lima-Landman MT, Lapa AJ. (2008). Inhibition of gastric acid secretion by a standardized aqueous extract of Cecropia glaziovii Sneth and underlying mechanism. Phytomedicine, 15, 462–469.
- Takeuchi H, Baba M, Shigeta S. (1991). An application of tetrazolium (MTT) colorimetric assay for the screening of anti-herpes simplex virus compounds. J Virol Methods, 33, 61–71.
- Tanae MM, Lima-Landman MT, De Lima TC, Souccar C, Lapa AJ. (2007). Chemical standardization of the aqueous extract of Cecropia glaziovii Sneth endowed with antihypertensive, bronchodilator, antiacid secretion and antidepressant-like activities. Phytomedicine, 14, 309–313.
- Velázquez E, Tournier HA, Mordujovich de Buschiazzo P, Saavedra G, Schinella GR. (2003). Antioxidant activity of Paraguayan plant extracts. Fitoterapia, 74, 91–97.
- Wang GF, Shi LP, Ren YD, Liu QF, Liu HF, Zhang RJ, Li Z, Zhu FH, He PL, Tang W, Tao PZ, Li C, Zhao WM, Zuo JP. (2009). Anti-hepatitis B virus activity of chlorogenic acid, quinic acid and caffeic acid in vivo and in vitro. Antiviral Res, 83, 186–190.