Abstract
Context: Long pepper, Piper longum Linn. (Piperaceae), is widely used in traditional Mongolian medicine for treating hyperlipidemia and coronary heart disease.
Objective: To investigate the hypolipidemic effects of a new piperine derivative GB-N isolated from long pepper in high-fat diet-fed rats.
Methods: The levels of serum total cholesterol, triacylglycerols (TG), low-density lipoprotein cholesterol (LDL-C) and high-density lipoprotein cholesterol (HDL-C) were determined by enzymatic colorimetric method. The levels of 3-hydroxy-3-methylglutaryl coenzyme A (HMG-CoA), CYP7A1, lecithin cholesterol acyltransferase (LCAT) and LDL receptor (LDLR) mRNA and protein expression were detected by real-time polymerase chain reaction and western blot analysis.
Results and discussion: Compared with model rats, oral administration of GB-N at doses of 2.5–10 mg/kg to hyperlipidemic rats could significantly decrease the levels of serum TG from 1.54 mmol/L in hyperlipidemic rats to 0.94–1.02 mmol/L, with an increase in serum HDL-C levels from 0.40 mmol/L in hyperlipidemic rats to 1.21–2.26 mmol/L. Treatment with GB-N (10 mg/kg) could also significantly upregulate levels of hepatic HMG-CoA reductase, CYP7A1, LCAT and LDLR mRNA and protein expression.
Conclusion: GB-N had hypolipidemic activity via regulating lipid metabolism pathways in liver of hyperlipidemic rats and could be explored as a potential agent for the prevention of hyperlipidemia diseases.
Introduction
Cholesterol and lipids such as phospholipids and triglycerides (TG) play critical roles in the maintenance of internal homeostasis of the organisms and the histological organization and intermediary metabolism of eukaryotes. However, abnormalities in lipid metabolism are attributed to many disease states, including atherosclerosis, which is a main nosogenesis of many cardiovascular and cerebrovascular disorders. The identification and treatment of dyslipidemia is a high clinical priority in cardiovascular medicine in both the primary and secondary prevention settings (CitationRubenfire et al., 2010). Although statins are effective for treating hyperlipidemia, the cost is still high, which may impede adequate treatment in developing countries with lower income (CitationAlsheikh-Ali et al., 2004). By comparison, plant materials and those extracts are characterized by minimal adverse effects and multiple targets in preventing and curing hyperlipidemia (CitationJi & Gong, 2008; CitationDaisy et al., 2009; CitationFeng et al., 2011; CitationPatel & Mishra, 2011). Therefore, developing new and cost-effective herbal medications for dyslipidemia treatment is welcome in the clinic.
Long pepper [Piper longum Linn. (Piperaceae)], the grown ear-fruit, is widely used in traditional Mongolian medicine for treating hyperlipidemia and coronary heart disease. Increasingly, considerable attention has been given to the antioxidant, antiinflammatory, antiallergic, immunomodulatory and antitumor substances contained in the fruit. The primary constituents isolated from the herb are piperine, piperlongumine, sylvatin, sesamin, diaeudesmin piperlonguminine, pipermonaline and piperundecalidine (CitationSunila & Kuttan, 2004; CitationSunila & Kuttan, 2005; CitationPullela et al., 2006; CitationKumar et al., 2011). GB-N, a new N-isobutylamine product of piperine derivative, N-isobutyl-5-(3,4-methylenedioxyphenyl) -2E,4E-pentadienamine, was isolated from the material and has in vivo hypolipidemic potential according to our previous reports (published in Chinese). The structure of the compound as shown in was determined using spectroscopic techniques including UV, mass spectrometry, and 1H and 13C nuclear magnetic resonance (NMR) by comparison of the spectral data in the previous reports (CitationLee et al., 2008; CitationEe et al., 2009). These interesting findings motivated us to prepare a new agent that might hold promising antidyslipidemic and antiatherosclerotic benefits. Therefore, the aim of this study was to study the potential of such modifications on the hypolipidemic activity and its regulating mechanisms on the lipid metabolic-related factors [3-hydroxy-3-methylglutaryl coenzyme A reductase (HMG-CoA reductase), cytochrome P450 7A1 (CYP7A1), lecithin cholesterol acyltransferase (LCAT) and low-density lipoprotein receptor (LDLR)] in the high-fat diet-fed-induced hyperlipidemic rats.
Materials and methods
Animals
A total of 60 adult male Wistar rats with initial weight of 140–160 g were provided by Laboratory Animal Research Center, University of Inner Mongolia and were housed under standard housing conditions (temperature: 24 ± 1°C; light/dark cycle: 10/14 h). Experiments reported in this study were carried out in accordance with local guidelines for the care of laboratory animals of University of Inner Mongolia and with the 1996 Guide for the Care and Use of Laboratory Animals (Institute of Laboratory Animal Resources on Life Sciences, National Research Council, National Academy of Sciences, Washington DC, USA) and were approved by the ethics committee for research on laboratory animal use of the institution [No. SCXK (meng) 2007-0001].
Drugs and chemicals
Piperine derivative (GB-N) was donated by Institute of Macromolecular Chemistry and Mongolian Medicine, Inner Mongolia University, China. The apparent content of the compound was determined quantitatively as 98.4% by high-performance liquid chromatography analysis. Simvastatin was provided by North China Pharmaceutical Co. Ltd. (Shijiazhuang, Hebei, China) with the purity >95%. All other chemicals were obtained from local sources and were of analytical grade. All compounds were prepared as suspension and administered orally with 0.5% (w/v) sodium carboxyl methyl cellulose as vehicle.
Extraction and isolation of GB-N
Air-dried herb (2 kg) was extracted with ethanol for 4 h by conventional reflux extraction to give a brown extract. Then it was subjected to a silica gel column using n-hexane:EtOAc (1.5:1) as solvent systems to give six fractions (A-F). Fraction D (1.47 g) continued silica gel chromatography elution with n-hexane: EtOAc (1.5:1) to yield compound GB-N (40 mg). The structures of the compounds were determined using spectroscopic techniques including UV, mass spectrometry, 1H and 13C NMR and distortionless enhancement by polarization transfer spectrometry ().
Experimental procedure
The rats were allowed 7 days for habituation and were fed with standard rodent chow. Starting from the initiation of the experiment, all rats were randomly divided into six groups, each group consisting of 10 rats. Except the control group, other groups were switched to high-fat rodent chow (modified from standard rodent chow, including 10% lard, 3% cholesterol, 0.5% sodium tauroglycocholate and 86.5% standard chow by weight) (CitationWang et al., 2010; CitationAissaoui et al., 2011; CitationFeng et al., 2011) and they had free access to food and water throughout the experimental period (14 days).
Rats in the GB-N treatment groups were orally administered daily at doses of 2.5, 5 and 10 mg/kg rat weight, respectively, sustaining until the end of the experiment, while the control group was treated with the same vehicle and the simvastatin treatment group was treated with simvastatin (10 mg/kg rat weight).
At the end of the experimental period and after 12 h of fasting with only free access to water, animals were anesthetized with chloral hydrate by intraabdominal way and sacrificed at the same time of the day in all groups to avoid circadian fluctuations. Blood samples were collected and centrifuged at 3500g for 15 min to obtain serum. Livers were peeled off, weighed after washing with phosphate buffered salineand then kept in −80°C refrigerator until analysis.
Biochemical analysis
The serum levels of total cholesterol (TC), total TG, low-density lipoprotein cholesterol (LDL-C) and high-density lipoprotein cholesterol (HDL-C) were determined by using a commercial kit (BioSino Co. Ltd., Beijing, China).
Quantitative real-time polymerase chain reaction
Total RNA samples were isolated from liver tissues using a QuickPrep Total RNA extraction kit (Tianzhen Biotech Co. Ltd., Beijing, China). For preparation of cDNA, each total RNA sample was reverse-transcribed using Super Script RNase H-reverse transcriptase (Invitrogen, Carlsbad, CA, USA) and oligo (dT) primers (Invitrogen). The prepared cDNA samples were then purified by using a polymerase chain reaction (PCR) purification kit (Tianzhen Biotech Co. Ltd.). Quantitative real-time PCR (RT-PCR) (Tianzhen Biotech Co. Ltd.) was performed according to the manufacturer’s instructions. β-Actin was used as an internal control. The primer sequences used for the target genes are depicted in . The cDNAs were amplified using standard PCR buffer, 0.2 mM dNTP, 1 µM specific primer set, 0.5 µM 18S primer/competimer mix, 2 mM MgCl2 and 0.75 U of Taq DNA polymerase (Invitrogen) in 25 µl of total volume for 26–32 cycles. Each cycle consisted of 3-min denaturation at 94°C, 45-s annealing at 57°C and 45-s extension at 72°C. PCR products were separated on a 2.5% agarose gel with ethidium bromide by electrophoresis. Signal density was determined by laser scanning densitometry.
Table 1. Sequences of primers used in RT-PCR.
Western blot analysis
Total proteins were extracted from 10–30 mg aliquots of liver. Each sample was homogenized with chilled lysis buffer containing 10 mM Tris-HCl (pH7.4), 1% deoxycholic acid, 1% NP-40, 1% SDS, 150 mM NaCl, 1 mM EDTA and a protease inhibitor cocktail, and kept for 30 min on ice. After centrifugation at 140,000 rpm for 20 min at 4°C, the supernatant was collected as the total protein sample. Protein content was measured by bicinchoninic acid protein assay. Twenty milligrams of each protein sample was used in western blot analysis using rabbit polyclonal anti-LDLR, LCAT and CYP7A1 receptor antibodies and donkey anti-rabbit IgG secondary antibody (Invitrogen). The enhanced chemiluminescence plus western blotting detection system was used in visualization of the signal. For detection of GAPDH, an internal control, the membrane was reprobed with a Restore Western Blot Stripping Buffer, and then western blot analysis was performed using rabbit polyclonal anti-GAPDH antibody.
Statistical analysis
All data were expressed as mean ± standard error of the mean. The data obtained were subjected to statistical analysis using one-way analysis of variance followed by Dunnett’s post test for comparison between control and test groups using SPSS 12.0 software (SPSS Inc., Chicago, IL, USA). A p value <0.05 was considered to be significant. For quantification of the RT-PCR and western blot results, the gray scale of the pictures was quantified using Gel-Pro Analyzer. The fold changes of the mRNA and protein level were expressed relative to the readings of the control group.
Results
Characterization of GB-N
Yellow solid; mp 167–169°C; UV (methanol as solvent): 308.0 (existence of 3,4- methylene–bioxydran conjugated system), 338.5 (existence of long-chain conjugated system), 231.5 (existence of conjugated diene system); IR (KBr) vmax cm−1: 3270.33 (N-H stretching vibration), 1645.40 (carbonyl stretching vibration), 1153.80 (C-O-C asymmetrical stretching vibration), 1627.19–1440.83 (C stretching vibration of the benzene ring skeleton), 3072.53 (hydrogen stretching vibration absorption peak of benzene ring), 3007.02 (conjugated alkene CH stretching vibration absorption peak), 2960.26–2793.12 (the CH symmetric and asymmetric stretching vibration on alkyl); EI-MS m/z 273 [M]+; 1H-NMR (CDCl3, 300 MHz): δ 5.93 (d, 1H, J = 15.1, CH=CH-CH=CH), 7.33 (d, d, 1H, CH=CH-CH=CH), 6.65 (d, d, 1H, CH=CH-CH=CH), 6.73 (d, 1H, CH=CH-CH=CH), 6.92 (s, 1H, Ar-7H), 6.73 (d, 1H, J = 8.0, Ar-10H), 6.84 (d, 1H, Ar-11H), 5.93 (s, 2H, O-CH2-O), 3.16 (t, 2H, -N-CH2-), 1.81 (m, 1H, C2′-H), 0.93 (d, 6H, C3′-H, C4′-H); 13C-NMR (CDCl3, 75 MHz): δ 166.3 (-C=O), 122.54 (C-11), 140.84 (C-3), 123.46 (C-2), 124.75 (C-4), 138.71 (C-5), 130.92 (C-6), 105.73 (C-7), 148.20 (C-8), 148.2 (C-9), 108.47 (C-10), 123.2 (C-6′), 101.28 (O-CH2-O), 47.06 (-NH-CH2-), 28.66 (C-2′′), 20.18 (C-3′′, C-4′′).
Hypolipidemic effects of GB-N in hyperlipidemic rats
Fifteen days of high-fat diet feeding significantly increased the serum levels of TC, TG and LDL-C, and significantly decreased the serum level of HDL-C as shown in , indicating the model was successful in inducing hyperlipidemia in rats. The widely used hypolipidemic and HMG-CoA reductase inhibitor simvastatin significantly decreased the serum level of TC and LDL-C in hyperlipidemic rats (p < 0.05), but there were no significant differences in the serum levels of TG and HDL-C between simvastatin-treated group and model group (p > 0.05).
Figure 2. Effects of simvastatin, GB-N on the serum level of (A) total cholesterol (TC), (B) triglycerides (TG), (C) low-density lipoprotein cholesterol (LDL-C), and (D) high-density lipoprotein cholesterol (HDL-C). Compared with normal group, Δp < 0.05, ΔΔp < 0.01; compared with model group *p < 0.05, **p < 0.01.
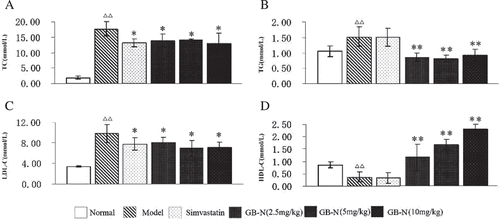
Over a period of 14 days of treatment, compared with model group, GB-N could significantly decrease the serum levels of TC, TG and LDL-C in a similar magnitude (p < 0.05, p < 0.01 and p < 0.05, respectively). Compared with the model group, repeated treatment with GB-N at the doses of 2.5–10 mg/kg also significantly increased the serum levels of HDL-C in a dose-dependent manner (). The serum level of HDL-C in GB-N-treated group (10 mg/kg) was significantly higher than that in the model group (p < 0.01).
Regulating effects of GB-N on lipid metabolic-related factors
RT-PCR analysis indicated that treatment with GB-N at the doses of 2.5, 5.0 and 10 mg/kg could significantly increase levels of LCAT mRNA (), while western blotting analysis also revealed significant increases in the protein expression of LCAT. These results were also consistent with the findings in hyperlipidemic rats treated with simvastatin.
Figure 3. Effects of GB-N on levels of 3-hydroxy-3-methylglutaryl coenzyme A (HMG-CoA), lecithin cholesterol acyltransferase (LCAT) and low-density lipoprotein receptor (LDLR) mRNA determined by real-time polymerase chain reaction (RT-PCR). Compared with model group, *p < 0.05, **p < 0.01.
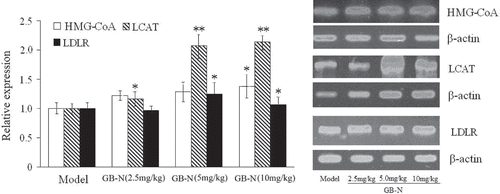
The hyperlipidemic rats treated with GB-N at the dose of 10 mg/kg also had a significant increase in the level of HMG-CoA mRNA compared with that of model rats (p < 0.05), while treatment at the doses of 2.5 and 5.0 mg/kg showed no significant changes (). On the other hand, treatment with GB-N (5 and 10 mg/kg) appeared to increase the levels of LDLR mRNA (both p < 0.05). In corresponding to this change, western blotting revealed significant increases of the levels of LDLR protein expression in rats treated with GB-N at the three doses (all p < 0.05). Interestingly, treatment with GB-N (2.5 and 10 mg/kg) also increased the levels of CYP7A1 protein expression, which is the rate-limiting enzyme in the synthesis of bile acid from cholesterol (). However, simvastatin could not impact the levels of LDLR protein expression in the liver of model control group.
Discussion
In the present study, piperine derivative GB-N showed obvious hypolipidemic effects on high-fat diet-fed-induced hyperlipidemia in rats, and the hypolipidemic effects of GB-N were correlated with the increase of lipid metabolic-related genes and proteins believed to modulate lipid metabolism.
To avoid the interference of estrogen on lipid metabolism (CitationTikkanen, 1996), only male rats were selected in this study. Animals fed with enriched high-fat diet could increase serum lipids, which has been widely used as an experimental model of hyperlipidemia for evaluating the pathophysiology of dyslipidemia-related disorders and the development of hypolipidemic drug. Consistent with literatures reported previously (CitationLü et al., 2009; CitationSolanki & Jain, 2010; CitationWang et al., 2010; CitationLin et al., 2011), in this study, rats fed with the high-fat diet used for 14 days significantly increased the serum levels of TC, TG and LDL-C but decreased the serum level of HDL-C, four critical biomarkers of hyperlipidemia. These results also demonstrated the reliability and robustness of this preclinical animal model of hyperlipidemia.
Simvastatin is one of the most widely used HMG-CoA reductase inhibitors for treating hyperlipidemia (CitationAdameova et al., 2009). At the dose of 10 mg/kg daily for 14 days, simvastatin markedly decreased the levels of TC and LDL-C but did not impact the serum level of TG and HDL-C.
Piperine is the main active ingredient of long pepper (Piper longum), the grown ear-fruit that is widely used in traditional Mongolian medicine for treating hyperlipidemia and coronary heart disease. A recent study indicated that piperine possesses robust hypolipidemic activity in rats (CitationVijayakumar & Nalini, 2006). As an effort to develop piperine-related hypolipidemic agents, we recently identified a new piperine derivative GB-N. Results in this study showed that the piperine derivative GB-N had great hypolipidemic activity in rats. In order to understand the molecular mechanisms that are responsible for the hypolipidemic effects of GB-N, the mRNA and protein levels of lipid metabolic-related genes involved in lipid metabolism were examined by using RT-PCR and western blotting techniques, respectively. It was found that GB-N could significantly increase the levels of LCAT mRNA and protein expression. LCAT is an important enzyme for HDL metabolism, which catalyzes the free cholesterol into esterification. Gene mutation at LCAT markedly reduces HDL-C level in humans (CitationHovingh et al., 2005). On the other hand, hyperlipidemia downregulates the LCAT mRNA level and decreases the activity of LCAT in blood plasma, which impairs the capability of HDL-C to remove cholesterol and leads to cholesterol accumulation in the blood. Our data suggested that GB-N could increase LCAT activity, although the causal or consequential relationship remained to be determined.
CYP7A1 is the rate-limiting enzyme for converting cholesterol into bile acid. Increased level of CYP7A1 is correlated to a decreased TC level in blood plasma (CitationGoodwin et al., 2003). Cholesterol and bile acid can induce and suppress CYP7A1 activity (CitationCheema et al., 1997; CitationMarrapodi & Chiang, 2000). In the current study, a significantly increased CYP7A1 protein level was observed in rats treated with GB-N, suggesting that the increased CYP7A1 activity could attribute to the decreased TC level.
Moreover, GB-N also could consistently increase the levels of LDLR protein expression. Increased LDLR level will increase the efficiency of reducing LDL from the blood and thus may be the reason of decreased LDL in the serum. Interestingly, GB-N did not consistently impact the mRNA or protein levels of HMG-CoA, the drug target of simvastatin. These results suggested that GB-N produced hypolipidemic effects by regulating LCAT, LDLR and CYP7A1, which was different from statins by downregulating HMG-CoA.
In conclusion, our study showed that GB-N could improve serum lipid profile in high-lipid diet-induced hyperlipidemic rats by regulating LCAT, LDLR and CYP7A1, the three pivotal factors in cholesterol metabolism. Efficacy of GB-N provided evidence that this functional plant compound could be developed as a valuable agent for prevention of hyperlipidemia.
Declaration of interest
This study was supported by the Natural Sciences Foundation of Inner Mongolia (2011), Importance Project of Science and Technology of China (2009ZX0913-103) and Caoyuan Yingcai Project of Inner Mongolian Government (2010).
References
- Adameova A, Harcarova A, Matejikova J, Pancza D, Kuzelova M, Carnicka S, Svec P, Bartekova M, Styk J, Ravingerová T. (2009). Simvastatin alleviates myocardial contractile dysfunction and lethal ischemic injury in rat heart independent of cholesterol-lowering effects. Physiol Res, 58, 449–454.
- Aissaoui A, Zizi S, Israili ZH, Lyoussi B. (2011). Hypoglycemic and hypolipidemic effects of Coriandrum sativum L. in Meriones shawi rats. J Ethnopharmacol, 137, 652–661.
- Alsheikh-Ali AA, Kuvin JT, Karas RH. (2004). Risk of adverse events with fibrates. Am J Cardiol, 94, 935–938.
- Cheema SK, Cikaluk D, Agellon LB. (1997). Dietary fats modulate the regulatory potential of dietary cholesterol on cholesterol 7 alpha-hydroxylase gene expression. J Lipid Res, 38, 315–323.
- Daisy P, Eliza J, Mohamed Farook KA. (2009). A novel dihydroxy gymnemic triacetate isolated from Gymnema sylvestre possessing normoglycemic and hypolipidemic activity on STZ-induced diabetic rats. J Ethnopharmacol, 126, 339–344.
- Ee GC, Lim CM, Lim CK, Rahmani M, Shaari K, Bong CF. (2009). Alkaloids from Piper sarmentosum and Piper nigrum. Nat Prod Res, 23, 1416–1423.
- Feng LJ, Yu CH, Ying KJ, Hua J, Dai XY. (2011). Hypolipidemic and antioxidant effects of total flavonoids of Perilla frutescens leaves in hyperlipidemia rats induced by high-fat diet. Food Res Int, 44, 404–409.
- Goodwin B, Watson MA, Kim H, Miao J, Kemper JK, Kliewer SA. (2003). Differential regulation of rat and human CYP7A1 by the nuclear oxysterol receptor liver X receptor-alpha. Mol Endocrinol, 17, 386–394.
- Hovingh GK, de Groot E, van der Steeg W, Boekholdt SM, Hutten BA, Kuivenhoven JA, Kastelein JJ. (2005). Inherited disorders of HDL metabolism and atherosclerosis. Curr Opin Lipidol, 16, 139–145.
- Huang L, Wen K, Gao X, Liu Y. (2010). Hypolipidemic effect of fucoidan from Laminaria japonica in hyperlipidemic rats. Pharm Biol, 48, 422–426.
- Ji W, Gong BQ. (2008). Hypolipidemic activity and mechanism of purified herbal extract of Salvia miltiorrhiza in hyperlipidemic rats. J Ethnopharmacol, 119, 291–298.
- Kumar S, Kamboj J, Suman, Sharma S. (2011). Overview for various aspects of the health benefits of Piper longum Linn. fruit. J Acupunct Meridian Stud, 4, 134–140.
- Lee SA, Hwang JS, Han XH, Lee C, Lee MH, Choe SG, Hong SS, Lee D, Lee MK, Hwang BY. (2008). Methylpiperate derivatives from Piper longum and their inhibition of monoamine oxidase. Arch Pharm Res, 31, 679–683.
- Lin LY, Ker YB, Chang CH, Chen KC, Peng RY. (2011). Arabinogalactan present in the mountain celery seed extract potentiated hypolipidemic bioactivity of coexisting polyphenols in hamsters. Pharm Biol, 49, 319–326.
- Lü H, Chen J, Li WL, Ren BR, Wu JL, Kang HY, Zhang HQ, Adams A, De Kimpe N. (2009). Hypoglycemic and hypolipidemic effects of the total triterpene acid fraction from Folium Eriobotryae. J Ethnopharmacol, 122, 486–491.
- Marrapodi M, Chiang JY. (2000). Peroxisome proliferator-activated receptor alpha (PPARalpha) and agonist inhibit cholesterol 7alpha-hydroxylase gene (CYP7A1) transcription. J Lipid Res, 41, 514–520.
- Patel MB, Mishra SH. (2011). Hypoglycemic activity of C-glycosyl flavonoid from Enicostemma hyssopifolium. Pharm Biol, 49, 383–391.
- Pullela SV, Tiwari AK, Vanka US, Vummenthula A, Tatipaka HB, Dasari KR, Khan IA, Janaswamy MR. (2006). HPLC assisted chemobiological standardization of alpha-glucosidase-I enzyme inhibitory constituents from Piper longum Linn-An Indian medicinal plant. J Ethnopharmacol, 108, 445–449.
- Rubenfire M, Brook RD, Rosenson RS. (2010). Treating mixed hyperlipidemia and the atherogenic lipid phenotype for prevention of cardiovascular events. Am J Med, 123, 892–898.
- Solanki YB, Jain SM. (2010). Antihyperlipidemic activity of Clitoria ternatea and Vigna mungo in rats. Pharm Biol, 48, 915–923.
- Sunila ES, Kuttan G. (2004). Immunomodulatory and antitumor activity of Piper longum Linn. and piperine. J Ethnopharmacol, 90, 339–346.
- Sunila ES, Kuttan G. (2005). Protective effect of Piper longum fruit ethanolic extract on radiation induced damages in mice: a preliminary study. Fitoterapia, 76, 649–655.
- Tikkanen MJ. (1996). Estrogens, progestins and lipid metabolism. Maturitas, 23 Suppl, S51–S55.
- Vijayakumar RS, Nalini N. (2006). Piperine, an active principle from Piper nigrum, modulates hormonal and apo lipoprotein profiles in hyperlipidemic rats. J Basic Clin Physiol Pharmacol, 17, 71–86.
- Wang TC, Lin CC, Lee HI, Yang C, Yang CC. (2010). Anti-hyperlipidemic activity of spider brake (Pteris multifida) with rats fed a high cholesterol diet. Pharm Biol, 48, 221–226.