Abstract
Context: Diabetes mellitus (DM), a chronic disease, has been increasing and subsequently devastates the quality of life and economic status of the patients. Oxidative stress participates in development and progression of diabetes, in which levels of glutathione peroxidase (GPx) and superoxide dismutase (SOD) were changed in diabetic mice. Berberine has been widely used as an alternative medicine and proved to be effective for treatment of DM and dyslipidemia.
Objective: Impacts of berberine on regulation of GPx and SOD messenger RNAs (mRNAs), and glutathione (GSH) content were examined in diabetic mice to clarify its antioxidative stress potential.
Materials and methods: Noninsulin-dependent diabetes was induced in mice by a single intraperitoneal streptozotocin injection. Diabetic mice were daily treated with metformin (100 mg/kg/d) or berberine (200 mg/kg/d) for 2 weeks. The fasting blood glucose and GSH content were monitored. GPx and SOD mRNA expression were semi-quantified by reverse transcription-polymerase chain reaction.
Results: Berberine showed the same hypoglycemic potential as metformin, a hypoglycemic drug. Interestingly, berberine did not change levels of GPx, copper-zinc SOD (CuZn-SOD), and manganese SOD (Mn-SOD) mRNA in the normal mice but significantly recovered these levels in the diabetic mice to nearly the same levels as the normal. The GSH contents, including total GSH and reduced/oxidized GSH contents, were restored to the normal level by berberine, corresponded to GPx levels.
Discussion and conclusion: Berberine conveyed antioxidative effect via down- and up-regulation of GPx and CuZn-SOD expression, respectively. Therefore, use of berberine as a hypoglycemic compound for alternative treatment of DM could bring extra-beneficent consequence according to its antioxidative stress.
Introduction
The number of patients with diabetes mellitus (DM), one of the most costly and worrying chronic diseases, has been daily increasing and becoming a serious health care problem worldwide. The number of individuals with diabetes is increasing alarmingly owing to the aging of the population, obesity, and lack of exercise. Estimates indicate that by the year 2030 diabetes will affect 366 million people worldwide (CitationWild et al., 2004). Prevalence of DM in adults worldwide was estimated to be 4.0% in 1995 and to rise to 5.4% by the year 2025, and in the future, DM will be increasingly concentrated in urban areas (CitationKing et al., 1998). DM brings in negative quality of life and growing up in economic and social problems. Chronic DM results in many complications such as hypertension, heart attack, kidney failure, and finally morbidity or premature death. Therefore, advance researches for searching new strategies to improve DM status and quality of life in patients are still required.
Oxidative stress is a widely accepted participant in the development and progression of diabetes (CitationMaritim et al., 2003). Possible sources of oxidative stress and damage to proteins in diabetes include free radicals generated by autoxidation reactions of sugars and sugar adducts to protein and by autoxidation of unsaturated lipids in plasma and membrane proteins (CitationBaynes, 1991). The oxidative stress in diabetes may be amplified by a continuing cycle of metabolic stress, tissue damage, and cell death, leading to increase in free radical production (CitationBaynes, 1991). Moreover, high blood glucose level determines overproduction of reactive oxygen species (ROS) by mitochondrial electron transport chain (CitationPiconi et al., 2003). Such studies may lead to therapeutic approaches for limiting the damage from glycation and oxidation reactions and for complementing existing therapy for treatment of the complications of diabetes (CitationWest, 2000).
The free radical tissue-defense mechanisms, such as superoxide dismutase (SOD), glutathione peroxidase (GPx), and glutathione (GSH) are the important antioxidative mechanisms, against ROS and particularly superoxide anion radicals in mammals (CitationZelko et al., 2002; CitationMorel & Barouki, 1999). The isoform of SOD has copper (Cu) and zinc (Zn) in their catalytic center and is localized in intracellular cytoplasmic compartments (CuZn-SOD or SOD1). Another isoform of SOD has manganese (Mn) as a co-factor and has been localized to mitochondria of aerobic cells (Mn-SOD or SOD2) (CitationZelko et al., 2002). GPx exists in the cytosol as a homotetramer with each subunit containing a selenium atom incorporated within a catalytically active selenocysteine residue (CitationSukenaga et al., 1987). This amino acid is sterically exposed at a flat lipophilic impression on the protein allowing it to become oxidized by hydroperoxide approach (CitationLi et al., 1996). GSH has been described for a long time as a defensive agent against the action of toxic xenobiotics including drugs, pollutants, and carcinogens. As a prototype antioxidant, GSH has been involved in cell protection from the noxious effect by excess oxidant stress (CitationPompella et al., 2003).
SOD, GPx, and GSH level were changed in diabetic rats. GPx was increased, while SOD was decreased in the third or fourth week of diabetic rats (CitationKakkar et al., 1998). In chronic (12 weeks) diabetic rats, diabetes was associated with significantly increased activities of CuZn-SOD in the pancreas. On the other hand, the liver of diabetic rats showed a generalized decrease in GPx and SOD, as well as levels of reduced GSH (CitationWohaieb & Godin, 1987). Hence, potency of herbs or drugs to stabilize free radical defense mechanism enzymes, recovering to normal status, should be concerned in DM treatments.
Berberine () is a quaternary isoquinoline alkaloid found in many medicinal plants such as Berberis aristata (De.), Coptis chinensis (Franch.), Coptis japonica (Thunb.), and Coscinium fenestratum (Geartn.) (CitationRojsanga et al., 2006). Berberine extracts was employed as a remedy for treatment of several illness and abnormal symptoms. In 2008, CitationZhang and colleagues (2011) reported that berberine at a daily dose of 1 g for 3 months was effective and safe for treatment of DM type II and dyslipidemia. Though many studies have reported potential of berberine to reduce blood glucose level, its impact on expression of genes-related oxidative stress pathway in diabetes is still less. Therefore, it is worth to examine how berberine modulates regulatory expression of gene-related oxidative stress including GPx, copper-zinc SOD (CuZn-SOD), and manganese SOD (Mn-SOD) in streptozotocin-induced diabetes mouse liver. In addition, the fasting blood glucose (FBG) profile was carried out, and the level of GSH content in mouse liver was monitored.
Materials and methods
Chemicals
Berberine chloride (Ber) was supplied by Wako Pure Chemical Ind. (Osaka, Japan). Metformin (Met) and β-naphthoflavone was purchased from Sigma Chemical Co. (St. Louis, MO). Trizol® reagent and deoxynucleotide triphosphate mixture were supplied by Invitrogen® (Carlsbad, CA). ReverTraAce® and HelixAmp™ Taq DNA polymerase for reverse transcription and polymerase chain reaction (RT-PCR) were purchased from Toyobo Co., Ltd. (Osaka, Japan) and NanoHelix RM (Daejeon, South Korea), respectively. Random primers and RNase inhibitor were obtained from Takara Bio Inc. (Shiga, Japan). Forward and reverse primers of GPx, CuZn-SOD, Mn-SOD, and GAPDH genes were synthesized by Bio Basic, Inc. (Markham, Ontario, Canada). The primers of each gene are shown in previous studies (CitationMouatassim et al., 1999; CitationKondo et al., 2011); the product sizes of each gene were 197, 241, 246, and 145 bp, respectively. HT GPx assay kit was a product of Trevigen® (Gaithersbura, MD). All other laboratory chemicals were of the highest available purity from commercial suppliers.
Animals
Male ddY mice at 6 weeks of age were supplied by Sankyo Experimental Animals, Tokyo, Japan. All mice were housed in the University of Toyama’s Animal Center facility under the supervision of certified laboratory veterinarians and were treated according to a research protocol approved by the Toyama University’s Institutional Animal Care and Use Committee, Toyama, Japan, and the Animal Ethics Committee of Khon Kaen University, Khon Kaen, Thailand (Ref. No. AEKKU 06/2553). At all times, the mice were housed on paper chip bedding in plastic cages, with water and a commercial mouse diet supplied ad libitum. The mice quarters were air conditioned (20°C–23°C) and had a 12-h light/dark cycle.
Induction of diabetes in mice
Diabetes (noninsulin-dependent diabetes or DM type II) was induced by a single intraperitoneal injection of streptozotocin (STZ) in 0.05 M sodium citrate, pH 4.5, at a dose of 100 mg/kg (CitationHayashi et al., 2006; CitationSrinivasan & Ramarao, 2007). Two weeks after STZ injection, the tail vein blood was collected to determine FBG level. Only mice with the FBG level over 250 mg/dL were considered diabetic and included in the experiment.
Experimental design
Mice were mainly divided into two groups including normal and diabetic mice (n = 15). Each major group was randomly divided into three groups (n = 5) including control, metformin-treated, and berberine-treated mice, respectively. Metformin (100 mg/kg/d) and berberine (200 mg/kg/d) were dissolved in phosphate-buffer saline (PBS) and intragastrically given to mice daily for 2 weeks. The control group was daily intragastrically given 0.1 mL of PBS to assure nonsignificant change in the messenger RNA (mRNA) expression of the investigated genes from the vehicle. The FBG level of all mice were weekly monitored before and after 2 weeks of the treatment using a glucometer (Accu-Check® Advantage II Performa kits, Roche Diagnostics, Manheim, Germany), and the mouse urine was checked using Pretest 7aII strips (Wako®, Osaka, Japan) to confirm diabetic condition (CitationSakuma et al., 2001). During fasting, mice were deprived of food for 12 h but had free access to water. The mice were sacrificed at 24 h after the last treatment and the liver was excised immediately for preparation of total RNA as described elsewhere (CitationKondo et al., 2011).
Expression of GPx and SOD mRNA expression
Mouse GPx, CuZn-SOD, Mn-SOD, and glyceraldehyde 3-phosphate dehydrogenase (GAPDH) mRNAs were semi-quantified by RT-PCR. Hepatic total RNA was reverse-transcribed using ReverTraAce® reverse transcriptase (Toyobo Co., Ltd.), and then complementary DNA (cDNA) was amplified under the conditions recommended by the supplier of NanoHelix RM (Daejeon, South Korea). The conditions of PCR cycle were followed by the method of CitationMouatassim et al. (1999) and CitationKondo et al. (2011). After separation of the PCR products by 2% agarose gel electrophoresis, the target cDNA were detected under ultraviolet (UV) light in the presence of ethidium bromide and semi-quantified by Syngene® gel documentation (InGenius L, Cambridge, UK) and the GeneTools match program (Syngene®). The mRNA levels of the targets were normalized to that of GAPDH.
Measurement of GSH/GSSG content in mouse liver
Levels of reduced/oxidized GSH (GSH/GSSG) contents in mouse livers were determined using an HT GSH assay kit, according to the manufacturer’s instructions. Briefly, small pieces of liver were homogenated in distilled water. An aliquot of homogenate liver was determined for protein concentration by the Bradford method using bovine serum albumin as a standard. The homogenate liver was mixed with 5% metaphosphoric acid to precipitate the protein. After centrifugation at 12,000 × g for 10 min, the supernatant was collected and 50 µL of each sample was added to a 96-well plate, followed by the further addition of 150 µL of the reaction mixture. Coloring of the reaction was immediately measured at 405 nm by a UV-microplate reader (Biorad® Model-680, Philadelphia, PA) at 2 min intervals over a 10-min period. For the measurement of GSSG content, the homogenate liver was treated with 4-vinylpiridine before addition of the reaction mixture, followed by incubation at room temperature for 1 h. Total GSH, GSH, or GSSG content was determined by comparing the net slopes of the samples with the slope of the standard GSSG curve (CitationKondo et al., 2011).
Statistic analysis
The results were analyzed by one-way analysis of variance (ANOVA) followed by Tukey post-hoc test (SPSS ver. 17.0; SPSS Inc., Chicago, IL). Difference with p < 0.05 was considered to be statistically significant.
Results
Effect of berberine on FBG level
To confirm the potential of berberine on lowering blood glucose level of DM type II, all mice were monitored FBG level before and weekly after 2 weeks of the treatment of berberine. In accord with CitationHayashi et al. (2006), mice intraperitoneally treated with single dose of STZ (100 mg/kg) became diabetes with FBG over 250 mg/dL, while the control mice that received normal saline showed normal level of FBG (). Berberine lowered the FBG level in the STZ-induced diabetic mice to the level comparable to that did by metformin, a typical antiglycemic drug. The FBG level was not significantly changed by either berberine or metformin in the normal mice.
Effect of berberine on expression of genes-related oxidative stress in diabetic mice
To examine how berberine participated in regulatory expression of gene-related oxidative stress, the expression of GPx, CuZn-SOD, and Mn-SOD mRNA were determined in mouse livers. Metformin and berberine did not significantly change level of GPx, CuZn-SOD, and Mn-SOD expression in normal mice (). Expression of GPx mRNA was significantly elevated in diabetic mice (). Though metformin expressed strong anti-glycemic potential in diabetic mice, it did not lower GPx mRNA expression in the mice. Interestingly, berberine, besides effectively lowering the FBG level, significantly reduced the expression level of GPx mRNA back to the same level as the normal mice. Diabetic condition extensively down-regulated the expression of CuZn-SOD mRNA (). The level of CuZn-SOD mRNA was not inducible by metformin, while berberine recovered the expression of CuZn-SOD to nearly the same level as the normal ones. Expression of Mn-SOD mRNA was not significantly changed in either normal or diabetic mice (). Metformin and berberine did not change the level of Mn-SOD expression in both normal and diabetic mice.
Figure 3. Expression of glutathione peroxidase (GPx), copper zinc superoxide dismutase (CuZn-SOD), and manganese superoxide dismutase (Mn-SOD) messenger RNA (mRNA) in mouse liver. Both normal and diabetic mice were daily intragastrically given metformin (Met) (100 mg/kg/d), berberine (Ber) (200 mg/kg/d), or phosphate-buffer saline, respectively, for 2 weeks. Mice were decapitated 24 h after the last treatment and liver was immediately excised to prepare total RNA. Semi-quantitative determination of mRNA expression was performed using a pairs of primers specific to the investigated genes as described. The data are presented as the mean ± standard deviation (n = 5) from triplicate independent experiments. A significant difference was determined by one-way analysis of variance followed by Tukey post-hoc test. *p < 0.01, **p < 0.001 versus nontreatment (NT).
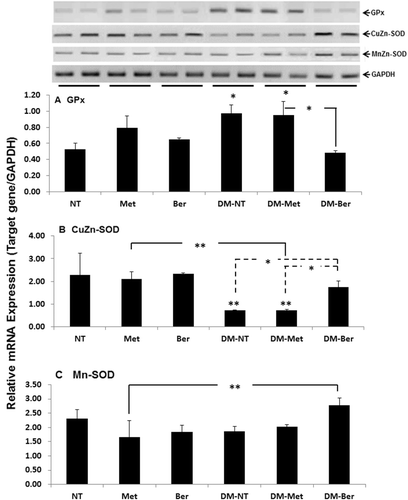
Effect of berberine on level of GSH in diabetic mice
To obtain information of a major antioxidant level in mouse liver, assessment of total GSH and reduced/oxidized GSH (GSH/GSSG) contents were carried out. Berberine, itself, did not modify the level of all types of GSH contents (). Level of total GSH and GSH/GSSG contents corresponded with those of GPx mRNA expression, namely they were significantly inducible by metformin and diabetic condition, while berberine itself did not change, but interestingly reduced, these all levels in diabetic mice to nearly the same level as those of the normal mice.
Figure 4. Total glutathione (GSH), reduced glutathione (GSH), and oxidized glutathione (GSSG) contents in mouse liver. Both normal and diabetic mice were daily intragastrically given metformin (Met) (100 mg/kg/d), berberine (Ber) (200 mg/kg/d), or phosphate-buffer saline, respectively, for 2 weeks. Mice were decapitated 24 h after the last treatment and liver was immediately excised to prepare liver homogenate. Levels of total and oxidized/reduced glutathione (GSH/GSSG) contents in mouse livers were determined using the HT glutathione assay kit. The data are presented as the mean ± standard deviation (n = 5) from duplicate independent experiments. A significant difference was determined by one-way analysis of variance followed by Tukey post-hoc test. *p < 0.05, **p < 0.001 versus nontreatment (NT).
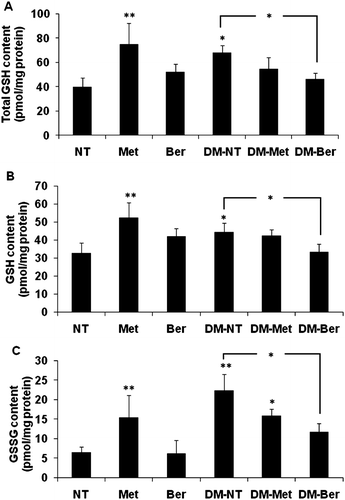
Discussion
DM is a metabolic disorder affecting metabolism of nutrients including carbohydrate, fat, and protein, subsequently leading to complication and regression of multiorgans (CitationMaritim et al., 2003). STZ induces diabetes by inhibition of insulin secretion and direct cytotoxic action on pancreatic β cell (CitationSzkudelski, 2001). In this study, a single intraperitoneal injection of STZ at a dose of 100 mg/kg to ddY mice resulted in gradual increase in FBG, and finally developed to DM type II within 2 weeks, confirmed by increase of the FBG level over than 250 mg/dL at 2 weeks after the treatment (CitationZhang et al., 2011; CitationHayashi et al., 2006). In addition, STZ generates ROS and leads to induction of oxidative stress (CitationLenzen, 2007), in which an increase of oxidative stress is widely known to be participant in development and progression of diabetes (CitationMaritim et al., 2003).
Berberine has been reported to improve glucose and lipid metabolic disorders by lowering FBG level (CitationTang et al., 2006). Intragastric administration of berberine at a dose of 100 mg/kg/d for 2 weeks, or 250 mg/kg/d (orally) for 4 weeks, decreased level of FBG in mice (CitationZhang et al., 2008; CitationWang et al., 2010; CitationTang et al., 2006). Correspondingly, the diabetic mice that daily intragastric administered berberine at a dose of 200 mg/kg/d for 2 weeks showed lower level of FBG than those of the nontreated diabetic mice, and equivalent to those of the metformin-treated ones. These observations assured antihyperglycemic potential of berberine.
Endogenous ROS at the physiological concentration helps to maintain homeostasis. However, accumulation of excess ROS for long periods causes chronic oxidative stress and adverse effects. This is particularly relevant and harmful for the islet cells, which are among those tissues having the lowest levels of intrinsic antioxidant defenses (CitationRobertson, 2004). Hyperglycemic-induced oxidative stress mechanisms are regulated via several pathways such as autooxidation of glyceraldehyde (CitationWolff & Dean, 1987) or activation of protein kinase C (CitationBrownlee, 2001). Moreover, consequence of oxidative stress on islet cells causes lipotoxicity and increase of islet apoptosis (CitationRobertson, 2004). Hence, several antioxidant compounds have been evaluated as protectors against oxidative stress for diabetes such as N-acetylcysteine (CitationTanaka et al., 1999) and nicanartine (CitationHammes et al., 1997).
In this study, the impact of berberine on the pathway of oxidative stress, including regulatory expression of gene-related oxidative stress and level of the GSH content, were determined in STZ-induced diabetic mouse liver. As expected, GPx expression was elevated against ROS production in oxidative stress, which highly occurred in the acute stage of DM (CitationTang et al., 2006), while decrease of CuZn-SOD directly involved in the pathogenic process of diabetes (CitationLikidlilid et al., 2007). Moreover, CitationWohaieb and Godin (1987) reported that the liver of diabetic rats showed a generalized decrease in SOD activity, corresponded to our results (). Diabetic mice receiving berberine (200 mg/kg/d), equivalent to the daily recommended regimen in human, up to 2 weeks significantly recovered the level of hepatic GPx and CuZn-SOD expression to those of the normal mice, while metformin did not. GSH is the most abundant nonprotein thiol and has many functions in vivo. The major role of GSH is maintenance of cellular redox balance. It plays a role as a substrate of GPx, an antioxidative enzyme that scavenges various peroxides (CitationNaso et al., 2011; CitationUeno et al., 2002). Level of liver GSH was reduced in chronic (12 week) diabetic rats (CitationWohaieb & Godin, 1987). Since our study was investigated in acute (2 weeks) diabetic mice, increase in GSH, oxidized GSH (GSSG), and total GSH contents were noted. These observations suggested that GSH level in diabetes might due to severity and/or progression of the disease. Likewise, berberine, not metformin, showed its potential to preserve the level of total GSH and oxidized GSH contents to the level comparable to the normal one. Finally, potential of berberine to recover the level of GPx, SOD, and GSH levels to the normal might be an extra-benefit for balancing between the endogenous ROS and diabetic-induced oxidative stress, leading to delay progression of diabetes.
Conclusion
These findings supported an approach of using berberine as an antihypoglycemic compound, partly in acute DM type II, according to its dual extra-beneficent potentials to recover expression level of oxidative stress-related genes and to maintain the level of GSH content.
Acknowledgement
Thinnakorn Lao-ong wish to thank the National Research University-Khon Kaen University, Thailand, for providing the scholarship.
Declaration of interest
The authors declare no conflicts of interest.
References
- Baynes JW. (1991). Role of oxidative stress in development of complications in diabetes. Diabetes, 40, 405–412.
- Brownlee M. (2001). Biochemistry and molecular cell biology of diabetic complications. Nature, 414, 813–820.
- Hammes HP, Bartmann A, Engel L, Wulfroth P. (1997). Antioxidant treatment of experimental diabetic retinopathy in rats with nicanartine. Diabetologia, 40, 629–634.
- Hayashi K, Kojima R, Ito M. (2006). Strain differences in the diabetogenic activity of streptozotocin in mice. Biol Pharm Bull, 29, 1110–1119.
- Kakkar A, Mantha SV, RadhiJ, Prasad K. (1998). Increased oxidative stress in rat liver and pancreas during progression of streptozotocin-induced diabetes. Clin Sci, 94, 623–632.
- King H, Aubert RE, Herman WH. (1998). Global burden of diabetes, 1995-2025: Prevalence, numerical estimates, and projections. Diabetes Care, 21, 1414–1431.
- Kondo S, Chatuphonprasert W, Jaruchotikamol A, Sakuma T, Nemoto N. (2011). Cellular glutathione content modulates the effect of andrographolide on β-naphthoflavone-induced CYP1A1 mRNA expression in mouse hepatocytes. Toxicology, 280, 11–23.
- Lenzen S. (2007). The mechanisms of alloxan- and streptozotocin-induced diabetes. Diabetologia, 51, 216–226.
- Likidlilid A, Patchanans N, Poldee S, Peerapatdit T. (2007). Glutathione and glutathione peroxidase in type 1 diabetic patients. J Med Assoc Thailand, 90, 1759–1767.
- Li R, Coway DB, Mickle DAG, Weisel RD, Burton GW. (1996). Effect of vitamin E on human glutathione peroxidase (GSH-px1) expression in cardiomyocytes. Free Radical Biol Med, 21, 419–426.
- Maritim AC, Sanders RA, Watkins JB. (2003). Diabetes, oxidative stress, and antioxidants: A review. Biochem Mol Toxicol, 17, 24–38.
- Morel Y, Barouki R. (1999). Repression of gene expression by oxidative stress. Biochem J, 342, 481–496.
- Mouatassim S, Guerin P, Menezo Y. (1999). Expression of genes encoding antioxidant enzymes in human and mouse oocytes during the final stages of maturation. Mol Human Reprod, 5, 720–725.
- Naso F, Dias AS, Porawski M, Marroni NAP. (2011). Exogenous superoxide dismutase: Action on liver oxidative stress in animals with streptozotocin-induced diabetes. Exper Diabetes Res, Article ID 754132, 1–6.
- Piconi L, Quagliaro L, Ceriello A. (2003). Oxidative stress in diabetes. Clin Chem Lab Med, 41, 1144–1149.
- Pompella A, Visvikis A, Paolicchi A, Tata VD, Casini AF. (2003). The changing faces of glutathione, a cellular protagonist. Biochem Pharmacol, 66, 1499–1503.
- Robertson RP. (2004). Chronic oxidative stress as a central mechanism for glucose toxicity in pancreatic islet beta cells in diabetes. J Biol Chem, 279, 42351–42354.
- Rojsanga P, Gritsanapan W, Suntornsuk L. (2006). Determination of berberine content in the stem extracts of Coscinium fenestratum by densitometry. Med Princ Pract, 15, 373–378.
- Sakuma T, Honma R, Maguchi S, Tamaki H, Nemoto N. (2001). Different expression of hepatic and renal cytochrorne P450s between the streptozotocin-induced diabetic mouse and rat. Xenobiotica, 31, 223–237.
- Srinivasan K, Ramarao P. (2007). Animal models in type 2 diabetes research: An overview. Indian J Med Res, 125, 451–472.
- Sukenaga Y, Ishida K, Takeda T, Takagi K. (1987). Nucleotide sequence of a human gene for glutathione peroxidase. Nucl Acids Res, 15, 71–78.
- Szkudelski T. (2001). The mechanism of alloxan and streptozotocin action in B cells of the rat pancreas. Physiol Res, 50, 536–546
- Tanaka Y, Gleason CE, Tran PO, Harmon JS, Robertson RP. (1999). Prevention of glucose toxicity in HIT-T15 cells and Zucker diabetic fatty rats by antioxidants. Proc Natl Acad Sci USA, 96, 10857–10862.
- Tang LQ, Wei W, Chen LM, Liu S. (2006). Effects of berberine on diabetes induced by alloxan and a high-fat/high-cholesterol diet in rats. J Ethnopharmacol, 108, 109–115.
- Ueno Y, Kizaki M, Nakagiri R, Kamiya T, Sumi H, Osawa T. (2002). Dietary glutathione protects rats from diabetic nephropathy and neuropathy. J Nutr, 32, 897–900.
- Wang Y, Campbell T, Perry B, Beaurepaire C, Qin L. (2010). Hypoglycemic and insulin-sensitizing effects of berberine in high-fat diet- and streptozotocin-induced diabetic rats. Metabolism, 60, 298–305.
- West IC. (2000). Radicals and oxidative stress in diabetes. Diabetes Med, 17, 171–180.
- Wild S, Roglic G, Green A, Sicree R, King H. (2004). Global prevalence of diabetes: Estimates for the year 2000 and projections for 2030. Diabetes Care, 27, 1047–1053.
- Wohaieb SA, Godin DV. (1987). Alterations in free radical tissue-defense mechanisms in streptozocin-induced diabetes in rat, effects of insulin treatment. Diabetes, 36, 1014–1018.
- Wolff SP, Dean RT. (1987). Glucose autoxidation and protein modification. The potential role of ‘autoxidative glycosylation’ in diabetes. Biochem J, 245, 243–250.
- Zelko IN, Mariani TJ, Folz RJ. (2002). Superoxide dismutase multigene family: A comparison of the Cuzn-Sod (Sod1), Mn-Sod (Sod2), and Ec-Sod (Sod3) gene structures, evolution, and expression. Free Radical Biol Med, 33, 337–349.
- Zhang Q, Xiao X, Feng K, Wang T, Li W, Yuan T, Sun X, Sun Q, Xiang H, Wang H. (2011). Berberine moderates glucose and lipid metabolism through multi pathway mechanism. Evid-Based Complement Alternat Med, 2011, 1–10.
- Zhang Y, Li X, Zou D, Liu W, Yang J, Zhu N, Huo L, Wang M, Hong J, Wu P, Ren G, Ning G. (2008). Treatment of type 2 diabetes and dyslipidemia with the natural plant alkaloid berberine. J Clin Endocrinol Metab, 93, 2559–2565.