Abstract
Context: Dao-Tan decoction (DTD) is a Chinese herb prescription used to treat atherosclerosis or dizziness for centuries. Previous study shows that DTD could inhibit intercellular adhesion molecule-1 (ICAM-1) expression induced by tumor necrosis factor-α (TNF-α). However, its mechanism has never been clearly described.
Objective: To examine the hypothesis that DTD might inhibit TNF-α-induced ICAM-1 expression through regulating the mitogen-activated protein kinase (MAPK) pathways, involving Jun N-terminal kinase (JNK) and p38.
Materials and methods: The rats were orally administrated with DTD for 3 days (2.3 g/kg per day), then the serum was collected. Human umbilical vein endothelial cells (HUVECs) were cultured and stimulated by TNF-α with or without DTD serum. The expression of ICAM-1 mRNA was examined by reverse transcription-polymerase chain reaction and the expression of p38 and JNK was examined by Western blot analysis. Results: DTD serum significantly inhibits TNF-α-induced ICAM-1 expression by 17–41% on HUVECs. TNF-α-induced JNK and p38 activations, which were involved in ICAM-1 expression, were significantly inhibited with DTD serum treatment by 10–50% on HUVEC.
Discussion and conclusion: Based on the theory of traditional Chinese medicine (TCM), pathogenesis of atherosclerosis is caused by “blood” and “phlegm” attached on blood vessels. DTD has a function of “dissolving phlegm”, thus it is chosen for the treatment of atherosclerosis. This study demonstrated that DTD could inhibit the expression of ICAM-1, by significantly preventing the activation of JNK and p38, which are important factors of atherosclerosis. Therefore, the present study indicates the pharmacological basis for treatment of atherosclerosis with DTD.
Introduction
Atherosclerosis is known to be a chronic inflammatory disease characterized by a series of highly specific cellular and molecular responses (CitationRoss & Glomset, 1973; CitationRoss, 1993). Adhesion of circulating polymorphonuclear leukocytes to the vascular endothelium is a critical step in the inflammatory response. This interaction is mediated by inducible cell adhesion molecules, such as intercellular adhesion molecule-1 (ICAM-1), vascular cell adhesion molecule-1, and E-selectin, which are expressed on the surface of endothelial cells, through a series of complex events (CitationHuo & Ley, 2001; CitationGüray et al., 2004).
As an inducible cell surface glycoprotein, ICAM-1 belongs to the immunoglobulin superfamily, and is widely expressed at a low level, in leukocytes and endothelial cells and can be upregulated by proinflammatory cytokines (CitationStaunton et al., 1988). ICAM-1 plays a central role in a number of inflammatory and immune responses, because it serves as a counter-receptor for leukocyte β2-integrins CD11a/CD18 (lymphocyte function-associated antigen-1) and CD11b/CD18 (Mac-1) and mediates leukocytes and their migration across the vessel wall barrier (CitationSmith et al., 1989).
ICAM-1 expression is regulated primarily at the level of transcription (CitationBevilacqua, 1993), and induced by various stimuli, including tumor necrosis factor (TNF)-α, interferon (INF)-γ, IL-1β (CitationDustin et al., 1986) and thrombin (CitationKaplanski et al., 1998). A growing body of evidence implicates that the mitogen-activated protein kinase (MAPK) pathways, as c-Jun N-terminal kinase (JNK) and p38 MAPK, work as mediators of leukocyte adhesion in response to classical inflammatory stimuli, induced by TNF-α (CitationKaur et al., 2003; CitationBerk et al., 2001), which may promote adhesion molecule surface expression and result in atherosclerosis (CitationGutiérrez et al., 2007).
Dao-Tan decoction (DTD) is a safe and effective traditional Chinese medicinal formula used in the treatment of dizziness for centuries. Oral administration of DTD has benefic effects in the treatment of atherosclerosis (CitationDuan & Huang, 2011), stroke (CitationZhang, 2008), hyperlipidemia (CitationGuo, 2000), and fatty liver (CitationChen, 2001). In our previous study, we found that the DTD could inhibit ICAM-1 expression induced by TNF-α, in mouse’s cerebrovascular endothelial cells (CitationChen et al., 2008). Bearing in mind that MAPK pathways are involved in the modulation of ICAM-1 expression, we want to examine the hypothesis that the DTD might inhibit TNF-α-induced ICAM-1 expression through regulating the MAPK pathways, as JNK and p38, in cultured human umbilical vein endothelial cells (HUVEC). Serum pharmacological method was used in this study, since most of herbs contain complex components, and their pharmacokinetics remains unknown. It is difficult to investigate their pharmacological actions and mechanisms in vitro by directly adding crude extracts into cultured cells. It is therefore more reasonable to use the cell-free portion of a blood specimen, which contains the absorbed components and their metabolites, in pharmacological experiments in vitro (CitationBochu et al., 2005).
Materials and methods
Plant materials and preparation of DTD
The component herbs of DTD were used as follows (with voucher numbers): Pinellia Tuber (Pinellia ternata (Thunb.) Breit. Praeparatum) (091102), Arisaema cum Bile (Arisaema erubescens (Wall.) Schott) (100501), Tuckahoe (Wolfiporia cocos (Schw.) Ryv.&Gibn) (100555), Immature Bitter Orange (Citrus sinensis (L.) Osbeck) (100234), Epicarpium citri Exocarpium citri rubrum (Citrus grandis var. tomentosa Hort.) (218209101), Liquorice (Glycyrrhiza uralensis Fisch.) (100206), and Rhizoma zingiberis recens (Zingiber officinale Roscoe) (108610101) were mixed based on a dry weight ratio of 9:3:3:3:3:2:3. All the herb plants were extracted with standard methods according to Chinese Pharmacopoeia (China, 2000). All herbs were purchased from the Pharmacy Department of Xuanwu Hospital, and identified by Chinese herbalist in Pharmacy Department of Xuanwu Hospital. The authenticated voucher specimens are available in Pharmacy Department of Xuanwu Hospital.
The ingredients were decocted in 10-fold volumes of water at 100°C for 30 min twice, and then the two decocted solutions were mixed together. The solution was freeze dried under vacuum, and made into a powder. The powder was dissolved in distilled water to a final concentration of 1 g/mL (equivalent to dry weight of raw materials).
DTD serum preparation
Male Sprague–Dawley rats (Laboratory Animal Center of Xuanwu Hospital) initially weighting 200–250 g were used in this study. The animals were housed individually at 21°C with a 12-h light/12-h dark cycle. Rats had free access to a standard diet and drinking water.
Sprague–Dawley rats were randomly divided into two groups: control and DTD groups (ten animals per group). The control animals were fed with saline 5 mL/d/rat for 3 days by oral administration. The DTD treated animals were treated with DTD (2.3 g/kg body weight per day) for 3 days by oral administration. Blood was collected from the abdominal aorta at 2 h after the final administration, centrifuged at 2500 rpm for 20 min, then serum was collected, and filtered with 0.2 µm cellulose acetate membrane. The serum was treated in 56°C water bath for 30 min, and then was stored at −20°C until use.
All animals received humane care in compliance with the National Institute of Health Criteria for the Care of Laboratory Animals, and the study was approved by the Ethics Committee of Xuanwu Hospital.
Reagents and antibodies
Tissue culture medium 199 and Fetal Bovine Serum (FBS) were obtained from GIBCO (Grand Island, NY). Endothelial cells growth factor (ECGF) was acquired from Roche Inc. (Basel, Switzerland). TNF-α was obtained from Pepro Tech Inc. (Rocky Hill, NJ). Anti-p-JNK antibodies, anti-p-p38 antibodies, MAPK inhibitors SP600125 (JNK inhibitor) and SB203580 (p38 MAPK inhibitor) were obtained from Santa Cruz Biotechnology (Santa Cruz, CA). TRIzol and electrophoresis reagents were from Invitrogen (Carlsbad, CA). TaKaRa RNA PCR Kits (AMV) Version 3.0 was from Takara Bio (Tokyo, Japan). All reagents for cell culture were of tissue culture grade, and for RNA extraction, reagents were of molecular biology grade. All other materials were purchased from Sigma Co. (St. Louis, MO) except where indicated, and were of analytical grade.
Cell culture
HUVECs were isolated and cultured based on previously described methods (CitationJaffe et al., 1973). HUVEC was cultured in M199 media supplemented with 20% FBS, 100 µg/mL penicillin, 100 µg/mL streptomycin, 2 mmol/L glutamine, 20 µg/mL ECGF and 95 µg/mL heparin. Cells were grown in 75 cm2 flasks and maintained at 37°C in a 95%/5% humidified air/CO2 atmosphere. The cells were split 1:4 every 3 days; experiments were performed on cells at passage 4 to passage 8 when at 80–90% confluence.
The cells were divided into eight groups: (1) control group: cells were cultured with 20% FBS, (2) control serum group: cells were cultured with 10% DTD serum, (3) TNF-α group: cells were co-treated with 200 U/mL TNF-α for 15 min and then cultured with 20% FBS, (4) 5% DTD serum group: cells were pretreated with 5% DTD serum for 6 h and then co-treated with TNF-α for 15 min, (5) 10% DTD serum group: cells were pretreated with 10% DTD serum for 6 h and then co-treated with TNF-α for 15 min, (6) 20% DTD serum group: cells were pretreated with 20% DTD serum for 6 h and then co-treated with TNF-α for 15 min, (7) SP600125 group: cells were pretreated with SP600125 (20 µM, 30 min) and then co-treated with TNF-α for 15 min, (8) SB203580 group: cells were pretreated with SB203580 (10 µM, 30 min) and then co-treated with TNF-α for 15 min. We used normal adult oral dose of DTD as 10% serum dilution in the medium, and then used geometric series as 5% and 20% serum dilutions (data were not shown).
RNA extraction and reverse transcription
Total RNA was extracted from HUVEC using Trizol according to the manufacturer’s protocol. The concentration of RNA was determined by an absorbance at 260 nm and RNA was reverse transcribed to cDNA. cDNA was synthesized from 1 µL of RNA. cDNA synthesis was conducted in a reaction mixture containing MgCl2 2 µL, 10× RT Buffer 1 µL, RNase Free H2O 3.75 µL, dNTP 1 µL, RNase inhibitors 0.25 µL, AMV 0.5 µL and 0.5 µL primer; the total reaction volume was 10 µL. Reverse transcription was performed at 42°C, 30 min; 99°C, 5 min; 5°C, 5 min. cDNA was analysed immediately or stored at −20°C for reverse transcription-polymerase chain reaction (RT-PCR). The details of all oligonucleotide primer sequences, predicted product lengths and gene bank accession by amplification in RT-PCR are listed in . The RT-PCR consisted of upstream PCR primer 0.5 µL, downstream PCR primer 0.5 µL, 5 × PCR Buffer 10 µL, Taq enzyme 0.25 µL, Sterilized distilled water 28.75 µL in a 40 µL reaction mixture under the following conditions: pre-denaturation at 94°C for 2 min, denaturation at 94°C for 30 s, annealing at 52°C for 30 s, extension at 72°C for 45 s for a total of 30 cycles, after which a final extension step was performed at 72°C for 7 min. RT-PCR assay was carried out with ABI GeneAmp® PCR System 9600 (Applied Biosystems). After RT-PCR, 5 µL from each product was run out on a 1.5% agarose gel, stained with ethidium bromide and viewed over UV light. The production of RT-PCR was compared with Image Master VDS (Pharmacia Biotech) according the area and brightness of every band.
Table 1. Sequences of primers used for RT-PCR.
Western blot analysis
HUVEC was starved for 12 h in serum-free medium. The cells were washed with ice-cold phosphate-buffered saline (pH 7.4) and lysed in the sample buffer (20 mmo1/L Tris-HCl (pH 8.0), 150 mmol/L NaCl, 50 mmol/L NaF, 2 mmol/L EDTA, 1 mmo1/L Na3VO4, 1% NP-40, 1 µg/mL leupeptin, 1 µg/mL pepstatin, 1 mmol/L PMSF), 100 µL perwell of 6-well plate, then sonicated for 15 s to shear DNA and reduce sample viscosity. Insoluble material was pelleted in a centrifuge at 16,000g for 10 min. The phosphorylation status of JNK1/2 and p38 was analysed as described before (CitationBellido et al., 1997). Briefly, 20 µg of proteins (measured by the method of Bradford) were separated on 15% SDS-polyacrylamide gels and electrophoresis for 1 h at 100 V. Proteins separated by SDS–PAGE were then transferred to polyvinylidene difluoride membranes for 2 h at 60 V. The membranes were blocked and then incubated at 4°C overnight with a primary antibody. The primary antibodies used in this study were as follows: rabbit polyclonal anti-p-p38, anti-p38, anti-p-JNK and anti-JNK (1:1000). The next day, membranes were washed three times with TBST. Then incubated for 1 h with a polyclonal goat-anti-rabbit IgG antibody (1:1000) conjugated with horseradish peroxidase at room temperature and then washed with TBST three times for 5 min. And the detection was performed by using enhanced chemiluminescence system (Pierce, Rockford, IL). Quantitation of the intensity of the bands was scanned with an image scanner and analysed with NIH image 1.61. Data are presented as ratios of phosphor-p38 versus total p38 and phosphor-JNK1/2 versus total JNK1/2 in the sample.
Statistical analysis
Data are expressed as means ± S.D. of the indicated number of independent experiments. Statistical analyses were performed by one-way ANOVA, and the Newman–Keuls test was used to locate any significant differences identified in the ANOVA. A value of p < 0.05 was considered significant for all tests.
Results
Time- and dose-dependent effects of TNF-α on ICAM-1 mRNA expression
To determine the time-dependent effect of TNF-α, HUVEC was incubated with TNF-α (200 U/mL) for different times (6, 12, 24, 48, 72 h). RT-PCR reactions were performed to amplify ICAM-1 expression. The expression of ICAM-1 mRNA was induced and became detectable after 6 h and reached a maximum induction by 12 h of incubation. Then it declined and became undetectable by 72 h after incubation with TNF-α.
To determine the concentration-dependent effect of TNF-α, HUVEC was incubated with various concentrations (20, 100, 200 U/mL) of TNF-α for 12 h. RT-PCR results indicated that TNF-α increased the ICAM-1 mRNA expression in a concentration-dependent manner.
Inhibitory effect of DTD serum and MAPK pathways on the TNF-α-induced ICAM-1 expression in HUVEC
To investigate the role of DTD serum and MAPK on the expression of ICAM-1, the expression of ICAM-1 was determined after stimulation of HUVEC with TNF-α. The cells were pretreated with DTD serum (5%, 10%, and 20%) for 6 h and then co-treated with TNF-α (200 U/mL) for 12 h. The ICAM-1 mRNA levels induced by TNF-α were significantly reduced from 17 to 41%, as the concentration of DTD serum increased (). Also, the ICAM-1 mRNA level decreased significantly after the addition of SP600125 (20 µM), a JNK inhibitor and SB203580 (10 µM), a specific p38 inhibitor. These results indicate that DTD serum inhibits TNF-α-induced ICAM-1 expression. JNK and p38 are involved in TNF-α-induced ICAM-1 expression as previous reports (CitationKim et al., 2007).
Figure 1. RT-PCR analysis of TNF-α-induced ICAM-1 expression in HUVEC. (A) Cells were treated with TNF-α (200 U/mL) for 6, 12, 24, 48, 72 h. (B) Cells were treated with various concentrations (20, 100, 200 U/mL) of TNF-α for 12 h. The band intensities were assessed by scanning densitometry. Data were presented as means ± S.D. of three independent experiments. One-way analysis of variance was used to compare the multiple group means followed by Newman–Keuls test (*p < 0.05, **p < 0.01, vs. control group).
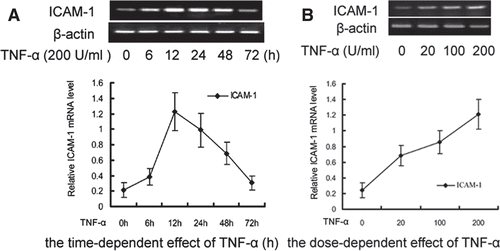
Figure 2. Effects of DTD serum on the expression of ICAM-1 induced by TNF-α through the JNK (A) and p38 (B) pathways. (A) The cells were pretreated with DTD serum (5%, 10%, 20%, 6 h), SP600125 (a JNK inhibitor, 20 µM, 30 min) and then co-treated with TNF-α. (1) Control group, (2) Control serum group, (3) TNF-α group, (4) 5% DTD serum group, (5) 10% DTD serum group, (6) 20% DTD serum group, (7) SP600125 group. (B) The cells were pretreated with DTD serum (5%, 10%, 20%, 6 h), SB203580 (a p38 inhibitor, 10 µM, 30 min) and then co-treated with TNF-α. (1) Control group, (2) Control serum group, (3) TNF-α group, (4) 5% DTD serum group, (5) 10% DTD serum group, (6) 20% DTD serum group, (7) SB203580 group. The band intensities were assessed by scanning densitometry. Data were presented as means ± S.D. of three independent experiments. One-way analysis of variance was used to compare the multiple group means followed by Newman–Keuls test (**p < 0.01, vs. control group; #p < 0.05, ##p < 0.01, vs. TNF-α group).
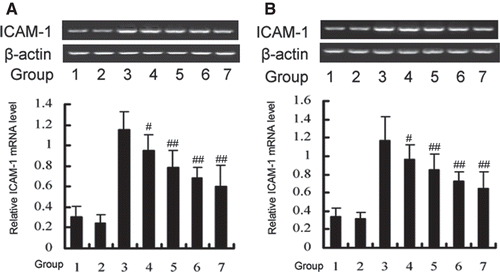
Inhibitory effect of DTD serum on TNF-α-induced JNK and p38 activation in HUVEC
While HUVEC was exposed to 200 U/mL TNF-α, JNK and p38 were activated within 15 min (data are not shown). To clarify whether DTD serum affects TNF-α-induced MAPK activation, we examined the effect of various concentrations of DTD serum on TNF-α-induced JNK and p38 activation in HUVEC (). The cells were pretreated with DTD serum for 6 h before the addition of TNF-α (200 U/mL) for 15 min for JNK and p38 activation. TNF-α-induced JNK and activation was inhibited from 12 to 50%, by DTD serum in a concentration-dependent manner. TNF-α-induced p38 activation was inhibited from 10 to 39%, by DTD serum in a concentration-dependent manner. These findings suggest that TNF-α-induced JNK and p38 activations, which are involved in ICAM-1 expression induced by TNF-α, are specifically sensitive to DTD serum in HUVEC.
Figure 3. DTD serum inhibited the TNF-α induced activation of JNK (A) and p38 (B). Cells were pretreated with DTD serum for 6 h and then co-treated with 200 U/mL TNF-α for 15 min. Cells were extracted and protein levels were determined by Western blot. DTD serum inhibited phosphorylation of JNK and p38. (A) The cells were pretreated with DTD serum (5%, 10%, 20%, 6 h), SP600125 (20 µM, 30 min) and then co-treated with TNF-α. (1) control group, (2) control serum group, (3) TNF-α group, (4) 5% DTD serum group, (5) 10% DTD serum group, (6) 20% DTD serum group, (7) SP600125 group. (B) The cells were pretreated with DTD serum (5%, 10%, 20%, 6 h), SB203580 (10 µM, 30 min) and then co-treated with TNF-α. (1) control group, (2) control serum group, (3) TNF-α group, (4) 5% DTD serum group, (5) 10% DTD serum group, (6) 20% DTD serum group, (7) SB203580 group. The band intensities were assessed by scanning densitometry. Data are presented as means ± S.D. of three independent experiments. One-way analysis of variance was used to compare the multiple group means followed by Newman–Keuls test (*p < 0.05, **p < 0.01, vs. control group; #p < 0.05, ##p < 0.01, vs. TNF-α group).
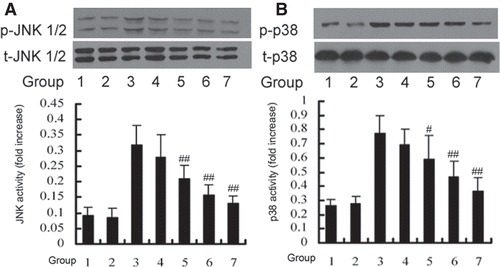
Discussion
Monocyte adhesion to the vascular endothelium and migration into the subendothelial areas of the artery are key initial steps in the development of atherosclerosis. It has also been reported that ICAM-1 was expressed in human atherosclerotic plaques (CitationHaverslag et al., 2008), suggesting that ICAM-1 plays a role in atherosclerosis.
Since injury of vascular endothelial cells has been implicated in the pathogenesis of atherosclerosis, and endothelial cell-adhesion molecules play a key role in this process, we examined the role of DTD serum on the expression of these adhesion molecules in the present study. Our results demonstrate that DTD serum inhibited TNF-α-induced ICAM-1 expression. It was also found that DTD serum inhibited TNF-α-induced ICAM-1 expression through regulating JNK and p38 activation in HUVEC.
Significant progress has been made in the treatment of atherosclerosis with Chinese medicine, the mechanism of compound recipe, single Chinese herb or effective ingredients of Chinese herbs on atherosclerosis were observed. According to the theory of traditional Chinese medicine (TCM), the pathogenesis of atherosclerosis is mainly because of the “blood” and “phlegm” stayed in vessels (CitationSong et al., 2008; CitationLei et al., 2009). On account of this, the TCM method of “dissolving phlegm” was chosen for the treatment of atherosclerosis patients. Previous study had shown that this method could alleviate these patients’ symptoms, reverses or delays the course of atherosclerosis, which mechanisms are possibly related with the function of protecting vascular endothelium (CitationHuang et al., 2007). Mean while, some other studies showed that some Chinese medicine decoctions, which have the function of “dissolving phlegm”, could influence atherosclerosis by the way of modulating the metabolism of proteoglycans (CitationWang et al., 2008).
DTD, a typical TCM prescription, can promote blood circulation, remove blood stasis, and scavenge the channels or blood vessels, by “dissolving phlegm” in the body. In clinical practice, it is applied to treat with stroke (CitationWang et al., 1986), headache (CitationSun, 2009), high blood lipid (CitationZhang, 1998) and some diseases (CitationLiu & Wang, 2009), which are caused by “phlegm stasis” in TCM. The DTD was mainly made by Pinellia Tuber, Arisaema cum Bile, Epicarpium citri Exocarpium citri rubrum, Immature Bitter Orange. And, β-sitosterol and hesperidin, which had the function of antioxidation, anticoagulant and antihyperlipidemics (CitationGupta et al., 2010; CitationJain & Parmar, 2011), are two main components of these four medicines (CitationZhao et al., 2009; CitationWei et al., 2010). Also, β-sitosterol and hesperidin could inhibit the superoxide anion generated by the xanthine oxidase/hypoxanthine system. Hesperidin and β-sitosterol had obvious synergies in eliminating free radicals, and their free radical scavenging capacity increases with the increase of drug concentration, in a certain range of concentration.
Different with previous studies which mainly examined the DTD’s function of decreasing lipid (CitationGuo, 2000), our results demonstrated that DTD could inhibit the expression of ICAM-1, and significantly prevent the activation of JNK and p38.
The roles of JNK and p38 in the process of HUVECs activation have been investigated, showing that JNK and p38 are both activated by TNF-α stimulation on endothelial cells (CitationKim et al., 2007) and induce expression of proinflammatory effector molecules (CitationHoefen & Berk, 2002). It was reported that Raf and Ras are involved in TNF-α-induced activation of JNK in HUVEC (CitationXu et al., 1998). And, it has also been demonstrated that the cytoskeletal remodeling and subsequent neutrophil transmigration in response to neutrophil adherence require the activity of p38 (CitationWang & Doerschuk, 2001). Thus, here we investigated the effects of specific inhibitors of JNK and p38 on the expression of ICAM-1 protein to determine if these signaling pathways were involved in the activation of ICAM-1 by TNF-α in HUVEC. The data support that JNK and p38 pathways were involved in ICAM-1 activation induced by TNF-α. Moreover, we found that DTD serum suppressed ICAM-1 expression in TNF-α-stimulated HUVEC, and this effect probably is related to the inhibition of TNF-α-induced JNK and p38 activations.
Conclusions
Taken together, our results suggest that p38 and JNK are regulators of ICAM-1 expression in TNF-α-stimulated HUVEC. And to our knowledge, we first examined the inhibitory effect of DTD serum on ICAM-1 is mediated, at least in part, by inhibiting p38 and JNK activation. Although the mechanisms of the beneficial effect of DTD serum against the p38 and JNK activation remain to be elucidated, the findings of the present study may shed light on the pharmacological basis for the clinical application of Chinese medicine DTD for treatment of atherosclerosis.
Declaration of interest
This study was supported by grants from the National Natural Science Foundation of China, No. 30772843 (PI: Zongxin Li), the National Natural Science Foundation of China, No. 30801481 (PI: Wenqiang Chen), the National Natural Science Foundation of China, No. 81001586 (PI: Fen Wang) and the Beijing Nova Program, No. 2008A090 (PI: Wenqiang Chen).
References
- Bellido T, Borba VZ, Roberson P, Manolagas SC. (1997). Activation of the Janus kinase/STAT (signal transducer and activator of transcription) signal transduction pathway by interleukin-6-type cytokines promotes osteoblast differentiation. Endocrinology, 138, 3666–3676.
- Berk BC, Abe JI, Min W, Surapisitchat J, Yan C. (2001). Endothelial atheroprotective and anti-inflammatory mechanisms. Ann N Y Acad Sci, 947, 93–109; discussion 109.
- Bevilacqua MP. (1993). Endothelial-leukocyte adhesion molecules. Annu Rev Immunol, 11, 767–804.
- Bochu W, Liancai Z, Qi C. (2005). Primary study on the application of Serum Pharmacology in Chinese traditional medicine. Colloids Surf B Biointerfaces, 43, 194–197.
- Chen Hancheng. (2001). Clinical effect of daotan decoction on fatty liver. Chin J Integr Med, 21, 457–458.
- Chen Wenqiang, Li Zongxin, Huang Xiaobo, Wang Ningqun, Li Bin. (2008). Influence of daotan decoction on the expression of ICAM-1 in cerebrovascular endothelial cells of rat. Chin J Inf Tradit Chin Med, 15, 28–29.
- Duan Xiaofang, Huang Shijie. (2011). Clinical observation on 68 Cases of angina pectoris treated by daotan decoction. Hunan J Tradit Chin Med, 27, 12–14.
- Dustin ML, Rothlein R, Bhan AK, Dinarello CA, Springer TA. (1986). Induction by IL 1 and interferon-γ: Tissue distribution, biochemistry, and function of a natural adherence molecule (ICAM-1). J Immunol, 137, 245–254.
- Güray U, Erbay AR, Güray Y, Yilmaz MB, Boyaci AA, Sasmaz H, Korkmaz S, Kütük E. (2004). Levels of soluble adhesion molecules in various clinical presentations of coronary atherosclerosis. Int J Cardiol, 96, 235–240.
- Guo Xuanxian. (2000). The research of daotan decoction on hyperlipidemia. Chin J Tradit Chin Med Pharm, 15, 71–72.
- Gupta P, Balwani S, Kumar S, Aggarwal N, Rossi M, Paumier S, Caruso F, Bovicelli P, Saso L, DePass AL, Prasad AK, Parmar VS, Ghosh B. (2010). β-sitosterol among other secondary metabolites of Piper galeatum shows inhibition of TNFα-induced cell adhesion molecule expression on human endothelial cells. Biochimie, 92, 1213–1221.
- Gutiérrez G, Mendoza C, Zapata E, Montiel A, Reyes E, Montaño LF, López-Marure R. (2007). Dehydroepiandrosterone inhibits the TNF-α-induced inflammatory response in human umbilical vein endothelial cells. Atherosclerosis, 190, 90–99.
- Haverslag R, Pasterkamp G, Hoefer IE. (2008). Targeting adhesion molecules in cardiovascular disorders. Cardiovasc Hematol Disord Drug Targets, 8, 252–260.
- Hoefen RJ, Berk BC. (2002). The role of MAP kinases in endothelial activation. Vascul Pharmacol, 38, 271–273.
- Huang F, Yao GX, Huang XL, Liu YN. (2007). Clinical observation on acupuncture for treatment of hypertension of phlegm-stasis blocking collateral type. Zhongguo Zhen Jiu, 27, 403–406.
- Huo Y, Ley K. (2001). Adhesion molecules and atherogenesis. Acta Physiol Scand, 173, 35–43.
- Jaffe EA, Nachman RL, Becker CG, Minick CR. (1973). Culture of human endothelial cells derived from umbilical veins. Identification by morphologic and immunologic criteria. J Clin Invest, 52, 2745–2756.
- Jain M, Parmar HS. (2011). Evaluation of antioxidative and anti-inflammatory potential of hesperidin and naringin on the rat air pouch model of inflammation. Inflamm Res, 60, 483–491.
- Kaplanski G, Marin V, Fabrigoule M, Boulay V, Benoliel AM, Bongrand P, Kaplanski S, Farnarier C. (1998). Thrombin-activated human endothelial cells support monocyte adhesion in vitro following expression of intercellular adhesion molecule-1 (ICAM-1; CD54) and vascular cell adhesion molecule-1 (VCAM-1; CD106). Blood, 92, 1259–1267.
- Kaur J, Woodman RC, Kubes P. (2003). P38 MAPK: Critical molecule in thrombin-induced NF-kappa B-dependent leukocyte recruitment. Am J Physiol Heart Circ Physiol, 284, H1095–H1103.
- Kim YS, Ahn Y, Hong MH, Joo SY, Kim KH, Sohn IS, Park HW, Hong YJ, Kim JH, Kim W, Jeong MH, Cho JG, Park JC, Kang JC. (2007). Curcumin attenuates inflammatory responses of TNF-α-stimulated human endothelial cells. J Cardiovasc Pharmacol, 50, 41–49.
- Lei Y, Wang ZH, Zhao H, Liu JG. (2009). Study of the relationship between carotid intima-media thickness and traditional Chinese medicine syndrome of dyslipidemia. Chin J Integr Med, 15, 112–116.
- Liu Jia, Wang Fengrong. (2009). From the sputum on the treatment of coronary heart disease and angina. J Pract Tradit Chin Intern Med, 12, 36–37.
- Ross R, Glomset JA. (1973). Atherosclerosis and the arterial smooth muscle cell: Proliferation of smooth muscle is a key event in the genesis of the lesions of atherosclerosis. Science, 180, 1332–1339.
- Ross R. (1993). The pathogenesis of atherosclerosis: A perspective for the 1990s. Nature, 362, 801–809.
- Smith CW, Marlin SD, Rothlein R, Toman C, Anderson DC. (1989). Cooperative interactions of LFA-1 and Mac-1 with intercellular adhesion molecule-1 in facilitating adherence and transendothelial migration of human neutrophils in vitro. J Clin Invest, 83, 2008–2017.
- Song JN, Liu JL, Fang XZ, Hu YH, Lei Y, Niu XH, Wu G, Chen BS, Ma YL, Chen B, Jin H. (2008). Relationship between plasma protein expression profiles and states of Zang-Fu organs in patients with phlegm or blood stagnation syndromes due to hyperlipidemia and atherosclerosis. Zhong Xi Yi Jie He Xue Bao, 6, 1233–1237.
- Staunton DE, Marlin SD, Stratowa C, Dustin ML, Springer TA. (1988). Primary structure of ICAM-1 demonstrates interaction between members of the immunoglobulin and integrin supergene families. Cell, 52, 925–933.
- Sun Zheng. (2009). 43 headache patients of phlegm and blood stasis syndrome were treated by dissolving phlegm and blood method. J Emerg Tradit Chin Med, 18, 1156–1157.
- Wang Jian, Huang Shuiqing, Xu Zhiwei. (2008). Effect of gualou xiebai banxia decocation on metabolism of proteoglycans in aorta in rabbits of atherosclerosis model. Chin J Arterioscler, 16, 290–292.
- Wang Q, Doerschuk CM. (2001). The p38 mitogen-activated protein kinase mediates cytoskeletal remodeling in pulmonary microvascular endothelial cells upon intracellular adhesion molecule-1 ligation. J Immunol, 166, 6877–6884.
- Wang Yongyan, Li Xiouqin, Deng Zhenming, Sun Sulun, Long Chengxiang, Ren Zhanli, Feng Xiaofei, Wang Yulai, Zhao Wei, Zhang Yunling, Ren Ke, Zhao Shuying, Zhang Liping, Chen Zhigang. (1986). 158 stroke patients were treated by dissolving phlegm and bowel relaxing method. Chin J Tradit Chin Med Pharm, 2, 86–88.
- Wei Huizhen, Xie Fei, Rao Yi, Du Yanlong, Jin Haoxin, Li Xinnan. (2010). Determination of flavone ingredients in Citrus aurantium, bitter orange immature, walnut peel and tangerine peel by internal standard multi-control method. Chin J Exp Tradit Med Form, 16, 75–77.
- Xu XS, Vanderziel C, Bennett CF, Monia BP. (1998). A role for c-Raf kinase and Ha-Ras in cytokine-mediated induction of cell adhesion molecules. J Biol Chem, 273, 33230–33238.
- Zhang Huiming. (2008). Daotan decoction is used in treating with 30 hemorrhagic stroke patients. Guid J Tradit Chin Med Pharm, 14, 26–27.
- Zhang Xuchu. (1998). 32 high blood lipid patients were treated by dissolving phlegm method. Beijing J Tradit Chin Med, 5, 33–34.
- Zhao Qing, Guo Hui, Cui Guihua. (2009). The research of several Araceae similarity of medicinal plants. Hebei Med J, 31, 2975–2977.