Abstract
Context: In the Indian traditional system of medicine, Streblus asper Lour (Moraceae) is prescribed for the treatment of diabetes mellitus.
Objective: In the present study, α-amyrin acetate isolated from S. asper, and the petroleum ether extract of S. asper stem bark (PESA) was screened for their antidiabetic properties in streptozotocin (STZ)-induced diabetic rats.
Materials and methods: Successive Soxhlet extraction of the dried stem bark with petroleum ether and then with ethanol (95%) yielded petroleum ether and ethanol extracts, respectively, which were concentrated under reduced pressure. Hyperglycemia was induced in rats by STZ (50 mg/kg, b.w.). Twenty-four hours after STZ induction, respective groups of diabetic rats received PESA (100, 250 and 500 mg/kg, b.w.) and α-amyrin acetate (25, 50 and 75 mg/kg, b.w.) respectively, orally daily for 15 days. Glibenclamide (0.5 mg/kg, orally) served as a reference. Blood glucose levels were measured on every 5th day during the 15 days of treatment. The serum lipid profiles and biochemical parameters, viz., serum glutamic oxaloacetic transaminase (SGOT), serum glutamic pyruvic transaminase (SGPT), alkaline phosphatase (ALP), insulin and glycosylated hemoglobin level, were measured.
Results: PESA significantly (p < 0.01) normalized blood-glucose levels and serum biochemical parameters as compared with those of STZ controls. α-Amyrin acetate (75 mg/kg, b.w.) exhibited maximum glucose lowering effect (71.10%) in diabetic rats compared to the other dose (25, 50 mg/kg) at the end of the study. The protective effect was further confirmed by histopathological examination of the liver.
Conclusion: PESA and α-amyrin acetate demonstrated remarkable antidiabetic activity in STZ-induced diabetic rats.
Keywords::
Introduction
Streblus asper Lour (Moraceae), commonly known as Shahra in Odiya, is widely distributed in the Shimlipal Bio-reserve, Mayurbhanj district of Odisha, India. It is a small tree with a crooked stem, bark light grey or greenish with faint ridges, laticiferous. It is used traditionally in the treatment of cancer (CitationRastogi & Dhawan, 1990), cholera, colic, diarrhea, dysentery, menorrhagia (CitationBhakuni et al., 1969), epilepsy and inflammatory swelling (CitationJain, 1991).
From the stem bark, α-amyrin acetate, lupeol acetate, β-sitosterol, α-amyrin, lupeol and diol (CitationRastogi et al., 2006), strebloside and mansonin (CitationFiebig et al., 1985) have been isolated. A pregnane glycoside named sioraside (CitationPrakash et al., 1992) has also been isolated ().
However, there is no scientific evidence to support the antidiabetic effect of S. asper stem bark. Hence, the objective of this study was to evaluate the antidiabetic activity of petroleum ether extracts of the stem bark of S. asper and its active isolated constituent in streptozotocin-induced diabetic rats.
Materials and methods
Plant material
The mature tree stem bark of S. asper was collected in August, 2008, from the Shimlipal Bio-reserve, Mayurbhanj district of Odisha, India and identified by Dr. M. S. Mondal, taxonomist of Botanical Survey of India, Howrah, India. A voucher specimen (CNH/I-I/(231)-2008/Tech. II/261) was deposited in the Department of Pharmaceutical Sciences, Utkal University, Vani Vihar, Bhubaneswar, Odisha, India. The collected stem bark was washed, shade-dried, and then milled to a coarse powder by a mechanical grinder for further studies.
Extraction and isolation
The powdered material (2 kg) was extracted with petroleum ether (60–80°C) and then with 4 L of ethanol (95%) in a Soxhlet apparatus. The solvent was removed under reduced pressure, to obtain petroleum ether (PESA, yield 2.92%) and ethanol (EESA, yield 4.42%) extracts, respectively, and tested for different constituents which revealed the presence of carbohydrate, phytosterols, glycosides, phenolic compounds, tannins, saponins, gums and mucilage. Both the fractions were evaluated for antidiabetic activity in STZ- induced diabetic rats. PESA was found to be more potent than EESA. Hence, PESA was further exploited for isolation, which led to the isolation of 3-acetoxy-α-amyrin. The petroleum ether extract (7 g) was chromatographed over the silica gel (100–200 mesh) packed in a glass column and eluted with solvents of increasing polarity from n-hexane to n-hexane-ethyl acetate mixtures (40%). Further purification of the fractions obtained by elution of the column with n-hexane: ethyl acetate (1.3: 8.7) and recrystallization from n-hexane yielded a white crystalline solid.
Acute toxicity studies
An acute toxicity study was performed for the extract according to the acute toxic classic method of CitationLitchfield and Wilcoxon (1949). The animals were divided into six groups containing 10 animals each. The PESA suspension was administrated orally in increasing doses up to 1500 mg/kg, b.w. These animals were observed for 2 h for behavioral, neurological and autonomic profiles and mortality and toxicity for 72 h.
Animals
Male Swiss albino rat weighing 150–200 g was used in the present investigation. They were housed in clean polypropylene cages and were fed with a standard pellet diet (Hindustan Lever, Kolkata, India) and water ad libitum. The animals were acclimatized to laboratory condition (temperature 25 ± 2°C) with dark/ light cycle (14/10 h) for one week before the start of the experiment. All procedures were approved by the Institutional Animal Ethical Committee, Regd. no. 1171/C/08/CPCSEA with approval no. 12/09/IAEC/SOAU, SOA University, Bhubaneswar, Odisha, India.
Induction of experimental diabetes
Diabetes was induced by a single intraperitoneal injection of freshly prepared streptozotocin (STZ) (50 mg/kg, b.w.) in ice cold citrate buffer (0.1 M, pH 4.5) to overnight-fasted rats (CitationKanter et al., 2003; CitationYoruk et al., 2003). Diabetes was identified by measuring glucose levels 48 h after injection of STZ.
Oral glucose tolerance test in normal and STZ-diabetic rats
The oral glucose tolerance test (OGTT) was performed for PESA and α-amyrin acetate in normal and STZ-induced diabetic rat model (CitationBonner-Weir, 1988). Four days after diabetes induction, the OGTT was performed by feeding glucose in the form of a solution through orally to STZ-induced diabetic rats fasted for 18 h. The animals were placed in nine groups and each group containing six animals. Group-I served diabetic control and received normal saline. Group-II received STZ only; animals of group III were treated with standard drug, glibenclamide (0.5 mg/kg b.w. p.o.), group IV-VI with PESA (100, 250 and 500 mg/kg b.w., p.o.), and group VII-IX with α-amyrin acetate (25, 50 and 75 mg/kg, b.w., p.o.), respectively. One hour later, glucose (2 g/kg) was administrated. Blood glucose levels from the tail vein were at 0, 2, 4, 6, 8, and 24 h after glucose administration using one-touch glucometer.
Estimation of blood glucose
Glucose was determined using the system active glucometer (Dr. Morepen, 9F, 31 codes).
Biochemical estimations
Lipid profiles and biomarkers were evaluated in normal and diabetic rats. Biochemical marker enzymes such as serum glutamic oxaloacetic transaminase (SGOT), serum glutamic pyruvic transaminase (SGPT), and alkaline phosphatase (ALP) were measured in the serum of the experimental animals (CitationReitman & Frankel, 1957; CitationBergmeyer & Brent, 1974). The serum lipid profiles such as total cholesterol, triglycerides, low-density lipoprotein (LDL) and high-density lipoprotein (HDL) were also measured (CitationBurstein & Scholnick, 1972; CitationFoster & Dunn, 1973). Serum insulin estimation was performed spectrophotometrically using a standard kit (Span Diagnostic Limited, Surat, India), whereas the HbA1c levels was measured using the glycosylated hemoglobin kit was purchased from Stangen Immunodiagnostics, Hyderabad, India.
Histopathological studies
Liver tissues were harvested from the sacrificed animals, and the fragments from tissues were fixed in 10% neutral formalin solution, embedded in paraffin and stained with haematoxylin and eosin.
Statistical analysis
Data were statistically evaluated using one-way analysis of variance (ANOVA), followed by post hoc Dunnett’s test using version 10 of the SPSS computer software. The values are expressed as mean ± S.D. and p < 0.01, were considered as significant.
Results
Characterization of secondary metabolite
This was obtained as a white crystalline solid (1.14 g), m.p. 122°C; TLC: Rf: 0.63 (n-hexane: ethyl acetate- 9.6: 0.4); IR (KBr, cm−1): 2915, 2142, 1736, 1455, 1371, 1242, 994; 1H NMR (CDCl3, 400 MHz): δH 5.14 (dd, 1H, J = 12.2; 4 Hz, H-12), 4.52 (m, 1H, H-3), 2.10 (brs, 3H, CH3COO), 1.92 (dd, 1H, J = 13.0 Hz, H-11), 1.12 (s, 3H, H-23), 1.07 (s, 3H, H-27), 1.00 (s, 3H, H-26), 0.95 (s, 3H, H-24), 0.91 (s, 3H, H-25), 0.88 (s, 3H, H-28), 0.85 (d, 3H, J = 8.0 Hz, H-30), 0.80 (d, 3H, J = 6.0 Hz, H-29); 13C NMR (CDCl3, 300 MHz): δC 37.70 (C1), 26.59 (C2), 80.96 (C3), 38.75 (C4), 55.25 (C5), 18.23 (C6), 32.86 (C7), 40.02 (C8), 47.63 (C9), 36.79 (C10), 23.36 (C11), 124.31 (C12), 139.63 (C13), 42.06 (C14), 28.74 (C15), 23.59 (C16), 33.74 (C17), 59.05 (C18), 39.64 (C19), 39.60 (C20), 31.24 (C21), 41.53 (C22), 28.06 (C23), 15.73 (C24), 16.73 (C25), 16.86 (C26), 23.22 (C27), 28.08 (C28), 17.49 (C29), 21.31 (C30), 21.39 (CH3COO), 171.80 (CH3COO); MS (m/z, %): 468 (M+), 218 (100), 202, 188.
It gave a positive Liebermann-Burchard test for triterpene. The IR spectrum showed an absorption band for -CH stretching at 2915, C=C at 2142 (as a small peak) and bending at 994. A characteristic ester carbonyl stretching was observed at 1736 cm−1.
The 1H NMR spectrum revealed the presence of two methine protons; one at δ 5.14 (dd, 1H, 12.2 Hz and 4 Hz) attached to the double bond (H-12), and the other is oxygenated at δ 4.52 (m, 1H) on 3-position (H-3) with corresponding carbon resonance at δ 80.96 (C3) in 13C NMR. A broad singlet appeared in δ 2.10 (brs, 3H) indicate the presence of one acetyl group in the molecule. Another multiplet at δ 1.92 was attributed to H-11. Besides, there are six tertiary methyl (appeared as singlet) and two secondary methyl (appeared as a doublet) at δ 1.12, 1.07, 1.00, 0.95, 0.91, 0.88, 0.85 (J = 8 Hz), and 0.80 (J = 6 Hz) were respectively assigned to H-23, H-27, H-26, H-24, H-25, H-28 and H-30, H-29.
Analysis of the 13C NMR spectrum confirms the presence of carbonyl carbon at δ 171.8 and one double bond carbon resonance in the molecule at δ 124.31 & 139.63 were assigned to C12-C13 double bond. There are 32 carbon signals found in the 13C NMR spectrum, and the EI mass spectra [M]+ m/z 468 (C32H52O2), with base peak at m/z 218, showed the fragmentary character for ursene type compounds. Based on the above spectroscopic analysis and comparative reported spectral data (CitationFeleke & Brehan, 2005; CitationDekebo et al., 2002), the isolate was identified as 3-acetoxy-α-amyrin.
Acute toxicity study
Acute toxicity studies revealed the non-toxic nature of the petroleum ether extract of S. asper. There was no lethality or toxic reaction found at any dose selected.
Effect of PESA, and α-amyrin acetate on fasting blood glucose and body weight in STZ-induced diabetic rats
After 15 days of treatment with STZ, the fasting blood-glucose level was significantly changed (). Treatment of PESA led to a dose-dependent fall in blood sugar levels by 68.98–70.24%. The initial antidiabetic activity was observed at the 5th day. The observed effect with isolated compounds was more significantly (p < 0.01) pronounced than that of the PESA. α-Amyrin acetate (75 mg/kg) exhibited maximum glucose lowering effect (71.10%) in diabetic rats compared to the other doses (25, 50 mg/kg) at the end of the study period. Glibenclamide (p < 0.01) exhibited a 55.76% reduction in blood-glucose level on the 15th day when compared to diabetic control.
Table 1. Effect of PESA and α-amyrin acetate on glucose levels in STZ-induced diabetic rats.
Biochemical enzymes
The effect of PESA on the serum GOT, GPT and ALP levels in normal and diabetic rats is shown in . The results showed that serum GOT, GPT and ALP levels in diabetic groups increased when compared with normal rats. The administration of α-amyrin acetate significantly (p < 0.01) decreased the serum GOT, GPT and ALP levels when compared with control diabetic rats.
Table 2. Effect of PESA and α-amyrin acetate on serum biomarkers in STZ-induced diabetic rats after treatment.
Lipid profiles
Streptozotocin treatment resulted in elevation of plasma triglycerides, total cholesterol, LDL-C and reduction in HDL-C levels as compared to the normal control rats (). The administration of PESA and α-amyrin acetate resulted in significant (p < 0.01) reduction in lipid profiles level in STZ diabetic rats with the maximum reduction in α-amyrin acetate treated groups.
Table 3. Effect of PESA and α-amyrin acetate on serum lipid profiles in STZ-induced diabetic rats after treatment.
There was a significant reduction in plasma triglycerides, total cholesterol and LDL-C levels of diabetic rats treated with α-amyrin acetate (2 and 4 mg/kg). However, there was a significant (p < 0.01) elevation in the HDL-C level in all treated diabetic rats on the 15th day as compared to the diabetic control group ().
Effect of PESA and α-amyrin acetate on serum insulin and HbA1c levels in STZ-induced diabetic rats
STZ caused a significant (p < 0.01) decrease in serum insulin. Administration of PESA and α-amyrin acetate caused significantly (p < 0.01) increase in insulin level at the end of the study period ().
Table 4. Effect of PESA and α-amyrin acetate on serum insulin, glycosylated hemoglobin (HbA1c) of STZ treated diabetic rats.
The blood HbA1c level in the diabetic control group was significantly (p < 0.01) higher than the blood HgA1c level of the normal control group. The extract, α-amyrin acetate and glibenclamide reduced significantly the elevated blood HbA1c level of the diabetic rats compared with the control diabetic group (p < 0.01) ().
Histopathalogical studies of liver
Histopathological evaluation of livers revealed that STZ-induced diabetes () resulted in glomerular hypertrophy, tubular dilation, intra-tubular hyaline casts and interstitial inflammatory cell infiltration. Treatment with extracts and isolated compounds reduced cell infiltration and maintaining near normal architecture. Treatment of rats with STZ led to several large areas of necrosis with severe inflammatory cell infiltration () in rat livers. Histological examination showed that the livers of rats treated with extracts and isolated compounds showed only slight neutrophil infiltration and swelling of cells (–).
Figure 2. Photomicrographs of 15 day experimental rat liver. Section A (10×) shows a normal histological section with well arranged cells and clear large central vein, cytoplasm and nucleus are well preserved. Section B (10×) shows the complete destruction of hepatocytes degeneration of central vein, fatty degeneration, loss of cell structure and damage in cell membrane in STZ-induced diabetic rat liver. Sections C, D, and E (10×) are treated with PESA (100, 250 & 500 mg/kg) and shows slight necrosis and restored to normal, respectively. Picture F, G & H (10×) depicts no damage and the hepatocyte architecture is near to normal by treatment with α-amyrin acetate (25, 50 & 75 mg/kg). These depict the well preserved hepatocytes, clear cytoplasm and nucleus with less neutrophil infiltration. In case of standard glibenclamide (0.5 mg/kg), I (10×) treatment shows no damage in the hepatocytes and well arranged with central vein.
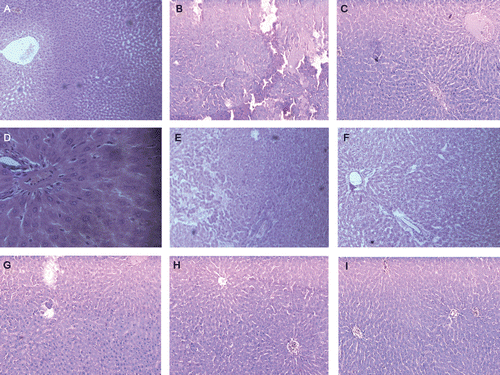
Discussion
STZ is a broad-spectrum antibiotic from Streptomyces achromogenes. Since the finding, that STZ possesses diabetogenic properties mediated by pancreatic β cell destruction, this compound has been widely used to induce diabetes in experimental animals. Evidence has accumulated suggesting that STZ induces oxidative stress. Oxidative stress is caused by a relative overload of oxidants, i.e., reactive oxygen species. Once, STZ enters inside the cell, it is spontaneously decomposed to form an isocyanate compound and a methyldiazohydroxide. Isocyanate compound and methyldiazohydroxide undergo intramolecular carboxylation and alkylation of cellular components, respectively. The DNA damage of β cells of the pancreas is mainly by alkylation with carbonium ion produced by methyldiazohydroxide (CitationWeiss, 1982; CitationWilson & Leiter, 1990).
It is well known that diabetes mellitus causes a disturbance in the uptake of glucose as well as glucose metabolism. In this study, the low dose of STZ (50 mg/kg) produced an incomplete destruction of pancreatic β cells even though the rats become permanently diabetic and induce disturbance in glucose metabolism (CitationAybar et al., 2001). The single high-dose STZ-induced diabetic rat is one of the animal models of human insulin-dependent diabetes mellitus (IDDM) or type I diabetes mellitus. In this model, diabetes arises from irreversible destruction of the islet cells of the pancreas, causing degranulation or reduction of insulin secretion and leads to hyperglycemia. In the search of hypoglycemic drugs from plants have been reported that phytoconstituents such as saponins, steroids, and flavonoids playing important roles (CitationPushparaj et al., 2001; CitationHosseinzadeh et al., 2002). The isolated α-amyrin acetate from S. asper is a steroid and stem bark of the tree were used by traditional people for the treatment of diabetes. In the present study, the high dose of PESA and α-amyrin acetate significantly reduced hyperglycemia in STZ-induced diabetic rats.
The increased levels of plasma glucose in STZ-induced diabetic rats were lowered by administration of α-amyrin acetate. The plasma glucose lowering activity was compared with glibenclamide, a standard hypoglycemic drug. Glibenclamide has been used for many years to treat diabetes (CitationTian et al., 1998).
Lipids play a vital role in the pathogenesis of diabetes mellitus. The most common lipid abnormalities in diabetes are hypertriglyceridemia and hypercholesterolemia. In this study, we have noticed elevated levels of serum lipids such as cholesterol and triglycerides in diabetic rats. The levels of increased serum lipids in diabetes represent a risk factor for coronary heart disease (CitationAl-Shamaony et al., 1994). Under normal circumstances, insulin activates lipoprotein lipase and hydrolyzes lipoprotein bound triglycerides (CitationNg et al., 1987; CitationWelihinda et al., 1982). The strong antihyperlipidemic activity of PEAS and its isolated compound could also through its control of hyperglycemia, as this is a major determinant of total cholesterol, LDL and triglycerides concentration. HDL is an antiatherogenic lipoprotein. It transports cholesterol from peripheral tissues into the liver and thereby acts as a protective factor against coronary heart disease. The level of HDL-cholesterol, which increased after α-amyrin acetate administration, might be due to the increase in the activity of lecithin cholesterol acyl transferase (LCAT), which may contribute to the regulation of blood lipids (CitationPatil et al., 2004). Administration of α-amyrin acetate lowers serum lipids. It is suggested that α-amyrin acetate may have the hypolipidemic activity.
The increase in the activities of serum GOT, GPT and ALP indicated that diabetes may be induced due to liver dysfunction and also found that liver was necrotized in STZ-induced diabetic rats. Therefore, an increase in the activities of GOT, GPT and ALP in plasma may be mainly due to the leakage of these enzymes from the liver cytosol into the blood stream (CitationFortson et al., 1985), which gives an indication of the hepatotoxic effect of STZ. On the other hand, treatment of the diabetic rats with α-amyrin acetate caused the reduction in the activity of these enzymes in plasma compared to the mean values of diabetic group and consequently, may alleviate liver damage caused by STZ-induced diabetes. These results are in agreement with those obtained by CitationEl-Demerdash et al. (2005) in rats. Our finding shows that oral administration of α-amyrin acetate produced the significant antihyperglycemic effect, lower both total cholesterol and triglyceride levels and, at the same time, increases HDL-cholesterol in STZ-induced diabetic rats.
HbA1c has usually served as an indicator of glycemic control. The present study demonstrates that PESA, and α-amyrin acetate, effectively control glycemia as it is evident from the significantly decreased glycosylated hemoglobin (HbA1c) level in the treated animals. The antihyperglycemic effect was also evident from the significant increase in the level of insulin in the extract and α-amyrin acetate treated animals. This investigation reveals the potential effect of α-amyrin acetate for use as a natural oral agent, with both hypoglycemic and hypolipidemic effects.
Conclusion
From the above results, it may be concluded that the petroleum ether extract of Streblus asper and its isolated compound, i.e., α-amyrin acetate has significant antidiabetic activity, thereby justifying the ethnopharmacological claim.
Acknowledgement
The authors are thankful to UDPS, Utkal University, SOA University, Bhubaneswar and SIPS, Jharpokharia, Mayurbhanj, Odisha for providing facilities to carry out this work.
Declarations of interest
The authors declare no conflicts of interest.
References
- Al-Shamaony L, al-Khazraji SM, Twaij HA. (1994). Hypoglycaemic effect of Artemisia herba alba. II. Effect of a valuable extract on some blood parameters in diabetic animals. J Ethnopharmacol, 43, 167–171.
- Aybar MJ, Sánchez Riera AN, Grau A, Sánchez SS. (2001). Hypoglycemic effect of the water extract of Smallantus sonchifolius (yacon) leaves in normal and diabetic rats. J Ethnopharmacol, 74, 125–132.
- Bergmeyer HU, Brent E. (1974). Methods of Enzymatic Analysis. 2nd Edition. New York: Verlag Chemie Weunheun, Academic Press, 735, 760.
- Bhakuni DS, Dhar ML, Dhar MM, Dhawan BN, Mehrotra BN. (1969). Screening of Indian plants for biological activity. II. Indian J Exp Biol, 7, 250–262.
- Bonner-Weir S. (1988). Morphological evidence for pancreatic polarity of β-cell within islets of Langerhans. Diabetes, 37, 616–621.
- Burstein M, Scholnick HR. (1972). Precipitation of chyclomicrons and very low density lipoproteins from human serum with sodium lauryl sulfate. Life Sci, 11, 177–184.
- Dekebo A, Dagne E, Gautun OR, Aasen AJ. (2002). Triterpenes from the resin of Boswellia neglecta. Bull Chem Soc Ethiop, 16, 87–90.
- El-Demerdash FM, Yousef MI, El-Naga NI. (2005). Biochemical study on the hypoglycemic effects of onion and garlic in alloxan-induced diabetic rats. Food Chem Toxicol, 43, 57–63.
- Feleke S, Brehan A. (2005). Triterpene compounds from the latex of Ficus sur I. Bull Chem Soc Ethiop, 19, 307–310.
- Fiebig M, Duh CY, Pezzuto JM, Kinghorn AD, Farnsworth NR. (1985). Plant anticancer agents, XLI. Cardiac glycosides from Streblus asper. J Nat Prod, 48, 981–985.
- Fortson WC, Tedesco FJ, Starnes EC, Shaw CT. (1985). Marked elevation of serum transaminase activity associated with extrahepatic biliary tract disease. J Clin Gastroenterol, 7, 502–505.
- Foster LB, Dunn RT. (1973). Stable reagents for determination of serum triglycerides by a colorimetric Hantzsch condensation method. Clin Chem, 19, 338–340.
- Hosseinzadeh H, Ramezani M, Danaei AR. (2002). Antihyperglycaemic effect and acute toxicity of Securigera securidaca L. seed extracts in mice. Phytother Res, 16, 745–747.
- Jain SK. (1991). Dictionary of Indian Folk Medicine and Ethanobotany. New Delhi: Deep Publication, 172.
- Kanter M, Yoruk M, Koc A, Meral I, Karaca T. (2003). Effects of cadmium exposure on morphological aspects of pancreas, weights of fetus and placenta in streptozotocin-induced diabetic pregnant rats. Biol Trace Elem Res, 93, 189–200.
- Litchfield JT Jr, Wilcoxon F. (1949). A simplified method of evaluating dose-effect experiments. J Pharmacol Exp Ther, 96, 99–113.
- Ng TB, Li WW, Yeung HW. (1987). Effects of ginsenosides, lectins and Momordica charantia insulin-like peptide on corticosterone production by isolated rat adrenal cells. J Ethnopharmacol, 21, 21–29.
- Patil UK, Saraf S, Dixit VK. (2004). Hypolipidemic activity of seeds of Cassia tora Linn. J Ethnopharmacol, 90, 249–252.
- Prakash K, Deepak D, Khare A, Khare MP. (1992). A pregnane glycoside from Streblus asper. Phytochemistry, 31, 1056–1057.
- Pushparaj NP, Tan HKB, Tan HC. (2001). The mechanism of hypoglycemic action of the semi-purified fractions of Averrhoa bilimbi in streptozotocin diabetic rats. Life Sci, 70, 535–547.
- Rastogi RP, Dhawan BN. (1990). Anticancer and antiviral activities in Indian medicinal plants: A review. Drug Develop Res, 19, 1–12.
- Rastogi S, Kulshreshtha DK, Rawat AK. (2006). Streblus asper Lour. (Shakhotaka): A review of its chemical, pharmacological and ethnomedicinal properties. Evid Based Complement Alternat Med, 3, 217–222.
- Reitman S, Frankel S. (1957). A colorimetric method for the determination of serum glutamic oxalacetic and glutamic pyruvic transaminases. Am J Clin Pathol, 28, 56–63.
- Tian YA, Johnson G, Ashcroft SJ. (1998). Sulfonylureas enhance exocytosis from pancreatic β-cells by a mechanism that does not involve direct activation of protein kinase C. Diabetes, 47, 1722–1726.
- Weiss RB. (1982). Streptozocin: A review of its pharmacology, efficacy, and toxicity. Cancer Treat Rep, 66, 427–438.
- Welihinda J, Arvidson G, Gylfe E, Hellman B, Karlsson E. (1982). The insulin-releasing activity of the tropical plant Momordica charantia. Acta Biol Med Ger, 41, 1229–1240.
- Wilson GL, Leiter EH. (1990). Streptozotocin interactions with pancreatic β cells and the induction of insulin-dependent diabetes. Curr Top Microbiol Immunol, 156, 27–54.
- Yoruk M, Kanter M, Meral I, Agaoglu Z. (2003). Localization of glycogen in the placenta and fetal and maternal livers of cadmium-exposed diabetic pregnant rats. Biol Trace Elem Res, 96, 217–226.