Abstract
Context: Curcuma caesia Roxb. (Zingiberaceae), commonly known as “Kala Haldi” in Bengali, has been traditionally used for the treatment of cancer, bruises, inflammation and as an aphrodisiac.
Objective: To evaluate the antitumor activity and antioxidant status of the methanol extract of Curcuma caesia (MECC) rhizomes on Ehrlich’s ascites carcinoma (EAC)-treated mice.
Materials and methods: In vitro cytotoxicity assay of MECC was evaluated by using Trypan blue method. Determination of in vivo antitumor activity was performed after 24 h of EAC cells (2 × 106 cells/mouse) inoculation; MECC (50 and 100 mg/kg i.p.) was administered daily for nine consecutive days. On day 10, half of the mice were sacrificed and the rest were kept alive for assessment of increase in lifespan. Antitumor effect of MECC was assessed by the study of tumor volume, tumor weight, viable and non-viable cell count, hematological parameters and biochemical estimations. Furthermore, antioxidant parameters were assayed by estimating liver and kidney tissue enzymes.
Results: MECC showed direct cytotoxicity (IC50 90.70 ± 8.37 μg/mL) on EAC cell line. MECC exhibited significant (p < 0.01) decrease in tumor volume, tumor weight, viable cell count and percentage increased the lifespan (57.14 and 88.09%) of EAC-treated mice. Hematological profile, biochemical estimation, tissue antioxidant assay significantly (p < 0.01) reverted to normal level in MECC-treated mice.
Conclusion: MECC possesses potent antitumor activity that may be due to its direct cytotoxic effect or antioxidant properties. Further research is in progress to find out the active principle(s) of MECC for its antitumor activity.
Introduction
Cancer represents one of the largest causes of death throughout the world. It already affects over six million lives annually and nearly 10 million new cases are diagnosed globally every year (Abdullaev, Citation2002). Moreover, incidences of cancer keep increasing on a global scale. There is only one plausible explanation for this: conventional medicine does not know the causes for cancer nor how this disease spreads. Because of this, there is no effective cancer therapy available and the disease keeps expanding on a global scale (Rath, Citation2001).
Although many synthetic anticancer agents are used in the treatment of cancer, side effects and emergence of synthetic drug resistant cancer cells among patients limits their use (Karthikeyan et al., Citation2007). The limitation of synthetic drugs highlights the pivotal need of anticancer agents from natural products, particularly medicinal plants (Chandrashekhar et al., Citation2011). Indeed, molecules derived from natural sources including plants, marine organisms and micro-organisms, have played, and continue to play, a dominant role in the discovery of leads for the development of conventional drugs for the treatment of most human diseases (Cragg & Newman, Citation2005).
The Ehrlich tumor was initially described as a spontaneous murine mammary adenocarcinoma (Dolai et al., Citation2012a). It is a rapidly growing carcinoma with very aggressive behavior and is able to grow in almost all strains of mice. In ascites form, it has been used as a transplantable tumor model to investigate the antitumor effects of several substances (Haldar et al., Citation2010).
Curcuma caesia Roxb. (Zingiberaceae), commonly known as “Kala Haldi” in Bengali and “Black turmeric” in English, is a perennial herb found throughout the Himalayan region, Northeast and Central India. It is also sparsely found in Papi Hills of East Godavari, root hills of the Himalayas and the North Hill forest of Sikkim (Karmakar et al., Citation2011c). The rhizomes are traditionally used in the treatment of hemorrhoids, leprosy, asthma, cancer, fever, wounds, vomiting, menstrual disorder, anthelmentic, aphrodisiac, gonorrheal discharges and inflammation (Amalraj et al., Citation1989; Singh & Jain, Citation2003). The rhizome paste is applied on bruises, contusions, and rheumatic pains. The rhizome is also used in dysentery, diarrhea and cough as an aromatic and as a source of d-camphor (Craker & Simon, Citation1996; Sarangthem & Haokip, Citation2010). Previous bioactivity studies have been revealed that plant rhizome possesses antifungal activity (Banerjee & Nigam, Citation1976), anxiolytic and CNS depressant activities (Karmakar et al., Citation2011a), neuropharmacological assessment (Karmakar et al., Citation2011c), anti-asthmatic, smooth muscle relaxant activity (Arulmozhi et al., Citation2006) and free radical scavenging activity against reactive oxygen and nitrogen species (Karmakar et al., Citation2011b). Presence of curcuminoids, 1,8-cineole, camphor, ar-turmeone, phenolics, flavonoids, protein and alkaloids were reported in the rhizomes of C. caesia (Paliwal et al., Citation2011; Sarangthem & Haokip, Citation2010). However, antitumor activity of its rhizomes has not yet been scientifically explored; therefore the main objective of present study is to evaluate antitumor and antioxidant status of the methanol extract of C. caesia (MECC) on Ehrlich ascites carcinoma (EAC) cell treated in Swiss albino mice.
Materials and methods
Plant material and preparation of extract
The rhizome of C. caesia was collected from the upper hill region of Sikkim in the month of September 2010 and identified by Dr. A. K. Panda, Taxonomist; Regional Research Institute (Ay), Gangtok, India and the voucher specimen (No. HPI/IK-01) was deposited in the Department of Pharmacology, Himalayan Pharmacy Institute, Sikkim, India for future reference. First, the collected rhizome was shade-dried at room temperature for 7 d and then powdered in a mechanical grinder. Next, the powdered plant material (225 g) was extracted by methanol using Soxhlet extraction apparatus. The solvent was completely removed under reduced pressure in a rotary evaporator. The concentrated extract was obtained by lyophilization and stored in vacuum desiccators (20 °C) for further use. The yield of the methanol extract was about 21.51% w/w.
Phytochemical analysis
The preliminary phytochemical analysis and planar chromatographic studies of MECC was done for qualitative analysis by using the standard method (Harborne, Citation1998).
Drugs and chemicals
Chemicals used were Trypan blue, 5-fluorouracil (Merck Limited, Mumbai, India). trichloroacetic acid, thiobarbituric acid, phenazine methosulphate, reduced nicotinamide adenine dinucleotide, nitroblue tetrazolin and dithionitrobenzene. They were obtained from Sigma-Aldrich, Bangalore, India. All the chemicals and reagents were used in highest analytical grade.
Animals
Male Swiss albino mice (20–25 g) of 8 weeks of age were used for the experiment. The mice were grouped and housed in polyacrylic cages (38 cm × 23 cm × 10 cm) with not more than six animals per cage. The animals were maintained under standard laboratory conditions (temperature 25–30 °C and 55–60% relative humidity with dark/light cycle 14/10 h) and were allowed free access to standard dry pellet diet and water ad libitum. The mice were acclimatized to laboratory conditions for 7 d before commencement of the experiment. All the procedures described were reviewed and approved by the University Animal Ethical Committee (367001/C/CPCSEA).
Acute toxicity
MECC was administered orally to male Swiss albino mice to evaluate the acute toxicity as per the reported method (Lorke, Citation1983).
Transplantation of tumor cell
EAC cells were obtained from Chittaranjan National Cancer Institute (CNCI, Kolkata, India). The EAC cells were maintained in vivo in Swiss albino mice by intraperitoneal transplantation of 2 × 106 cells per mouse after every 10 days and it is used for both in vivo and in vitro study (Haldar et al., Citation2010).
Assay for in vitro cytotoxicity
In vitro short term cytotoxicity of MECC was assayed by using Trypan blue exclusion method (Dolai et al., Citation2012a). At first, we have prepared different concentration of MECC solution as 250, 500, 1000, 1500, 2000 and 3000 μg/mL. Then, 1 × 106 EAC cells were suspended in 0.1 mL of phosphate buffered saline (PBS, 0.2 M, pH 7.4) and mixed with 100 μL of various aforementioned concentrations of MECC. Final concentration (25, 50, 100, 150, 200 and 300 μg/mL) of MECC was adjusted by PBS and incubated at 37 °C for 3 h. After the completion of incubation, the viability of the cells was determined using Trypan blue dye (0.4% in normal saline) and the percentage of cytotoxicity was determined by calculating % inhibition and IC50 values.
Treatment schedule for assessment of in vivo antitumor potential
Swiss albino mice (20–25 g) were divided into five groups (n = 12). All the animals in each groups except Group-I received EAC cells [2 × 106 cells/mouse intraperitoneally (i.p.)]. This was taken as day “0”. Group-I served as normal saline control (5 mL/kg i.p.) and group-II served as EAC control. After 24 h of EAC transplantation, Groups-III and -IV received MECC at a dose of 50 and 100 mg/kg i.p. for nine consecutive days, respectively. Group-V received reference drug 5-FU (20 mg/kg i.p.) for nine consecutive days (Bala et al., Citation2010). After administration of the last dose, six mice from each group were kept fasting for 18 h and blood was subsequently collected by direct cardiac puncture for the estimation of hematological and determination of serum biochemical parameters. The animals were then sacrificed for collection of liver and kidney tissues to check the different antioxidant parameters. The rest of the animals, in each group were kept alive and given food and water ad libitum to evaluate percentage increase in their life span to determine the mean survival time (MST).
Antitumor activity of MECC was assessed by observation of changes with respect to the following (tumor volume, tumor weight, percentage increase in life span (%ILS), viable/non-viable tumor cell count, hematological parameters, biochemical parameters and tissue antioxidant assay) parameters (Haldar et al., Citation2010).
Determination of tumor volume and tumor weight
The ascites fluid was collected from the peritoneal cavity. The volume was measured in a graduated centrifuge tube and expressed in milliliter (mL).
The tumor weight was measured by weighing the mice before and after the collection of the ascetic fluid from peritoneal cavity and expressed in grams (g).
Percentage increase in life span
The effect of MECC on percentage increased in life span was calculated on the basis of the mortality rates of the experimental mice, expressed as:
Estimation of viable/non-viable tumor cell count
The ascites fluid was taken in a white blood cell (WBC) pipette and diluted to 20 times with PBS. A drop of the diluted cell suspension was then placed on the Neubauer’s counting chamber and the number of cells counted.
The viability and non-viability of the cells were checked by Trypan blue dye exclusion assay method. The cells were stained with Trypan blue (0.4% in normal saline) dye. The cells that did not take up the dye were viable and those that took the dye were nonviable. The viable and nonviable cells were determined by using the following formula:
Determination of hematological parameters
Collected blood was used for the estimation of hemoglobin (Hb), red blood cell (RBC) and WBC count by standard procedures (D’Armour et al., Citation1965; Wintrobe et al., Citation1961).
Estimation of biochemical parameters
The blood samples were allowed to clot and the serum was separated by centrifugation at 5000 rpm for 10 min. Serum was utilized for the estimation of various biochemical parameters such as total protein, serum glutamic oxaloacetate transaminase (SGOT), serum glutamic pyruvic transaminase (SGPT) and alkaline phosphatase (ALP). All analyzes were performed by using commercially available kits (Span Diagnostics Ltd, Surat, India).
Determination of tissue antioxidant assay parameters
The tissue antioxidant assay was performed with liver and kidney tissues and evaluate by measuring the level of total protein (Span Diagnostics kit), protein carbonylation (PC) (Abraham et al., Citation1999), lipid peroxidation (Ohkawa et al., Citation1979), the amount of enzymatic catalase (CAT) (Aebi, Citation1974) and superoxide dismutase (SOD) (Kakkar et al., Citation1984) and nonenzymatic antioxidant system such as reduced glutathione (GSH) (Ellman, Citation1959).
Statistical analysis
All the experimental data are expressed as the mean ± SEM. Data was statistically analyzed by using one way analysis of variance followed by Dunnett’s post hoc test by Instat software (version 4). p Values of <0.01 were considered statistically significant.
Results
Phytochemical analysis
MECC extract indicated the presence of phenols and tannins (ferric chloride test), terpenoids (Liberman Burchard test), saponins (froth test), alkaloids (Dragendroff’s test), flavonoids (Shinoda test) and volatile oil (spot test).
Acute toxicity
The MECC was found to be safe in male Swiss albino mice up to the dose of 3000 mg/kg body weight per oral.
In vitro cytotoxicity
From the in vitro cytotoxicity study, it is clear that MECC showed direct cytotoxic effect on the EAC cell line, with an IC50 value of 90.70 ± 8.37 μg/mL ().
Tumor growth and survival parameters
Antitumor activity of MECC against EAC tumor bearing mice was assessed by the parameters such as tumor volume, tumor weight, cell count (viable and non-viable), MST and %ILS. The tumor volume, tumor weight and viable cell count were found to be significantly (p < 0.01) increased and non-viable cell count declined significantly (p < 0.01) in EAC control animals, when compared with normal control animals (). Administration of MECC at doses of 50 and 100 mg/kg significantly (p < 0.01) decreased the tumor volume and viable cell count. Non-viable cell count was significantly (p < 0.01) higher in MECC treated animals as compared to EAC control animals. Furthermore, the MST was increased to 33 ± 0.80 (% ILS = 57.14) and 39.5 ± 0.51 (% ILS = 88.09) on administration of MECC 50 and 100 mg/kg, respectively.
Table 1. Effect of MECC on tumor volume, tumor weight, total cell count, viable and nonviable cell count, MST and %ILS in EAC bearing mice.
Hematological parameters
There was significantly (p < 0.01) elevated levels of WBC and a significant (p < 0.01) reduction of RBC and Hb levels in EAC control group as compared to the normal control group (). Administration of MECC at doses of 50 and 100 mg/kg significantly (p < 0.01) reduced WBC count in respect to EAC control group. RBC count and Hb content were found to be significantly (p < 0.01) restored to the normal levels. These results implied the protective effect of MECC on the hematological profile of EAC bearing mice.
Table 2. Effect of MECC on hematological parameters in EAC bearing mice.
Biochemical parameters
Biochemical parameters like SGPT, SGOT and ALP indicates the significant (p < 0.01) elevated levels of liver functional enzymes in the serum of the EAC-treated group with respect to normal animals. The total protein content was found to be significantly (p < 0.01) decreased in the EAC control group was compared to the normal control group. Administration of MECC significantly (p < 0.01) increased the total protein content as compared with the EAC control mice (). Treatment with MECC (50 and 100 mg/kg) in EAC bearing mice significantly (p < 0.01) decreased the SGOT, SGPT and ALP levels as compared to EAC control groups ().
Figure 2. Effect of MECC on serum biochemical parameters such as total protein count (a), SGOT (b), SGPT (c) and ALP (d) in EAC bearing mice. Values are represented as mean ± SEM, where n = 6. aEAC control group versus normal control group, #p < 0.01. bAll treated groups versus EAC control group, *p < 0.01.
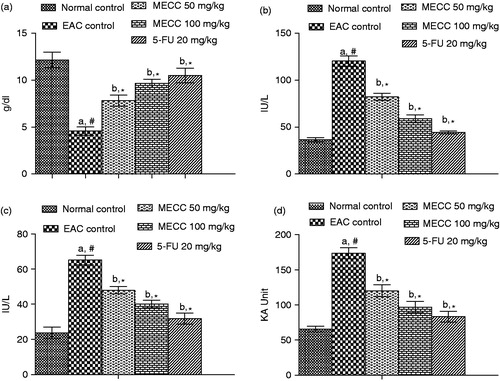
Tissue antioxidant assay parameters
Total protein content and PC were significantly (p < 0.01) raised in both liver and kidney in EAC control mice when compared to normal control mice. Administration of MECC at the doses of 50 and 100 mg/kg significantly (p < 0.01) attenuated the PC of both liver and kidney tissues () as compared to EAC control mice.
Figure 3. Effect of MECC on tissue antioxidant defense parameters like total protein count (a), protein oxidation (b), lipid peroxidation (c), CAT (d), reduce GSH (e) and SOD (f) in EAC bearing mice. Values are represented as mean ± SEM, where n = 6. aEAC control group versus normal control group, #p < 0.01. bAll treated groups versus EAC control group, *p < 0.01.
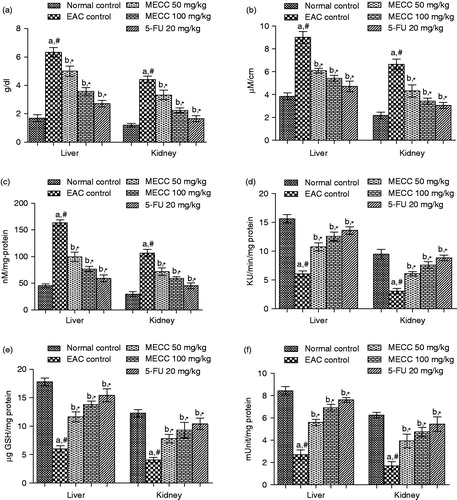
Lipid peroxidation results in the formation of reactive oxygen species and subsequently elevates the level of malondialdehyde (MDA) which induces cancer in liver and kidney tissues. In the present study, the MDA level was significantly (p < 0.01) increased in EAC control animals when compared with normal control animals. Interestingly, treatment with MECC significantly (p < 0.01) reduced the MDA levels as compared with EAC control group ().
The levels of CAT, reduced GSH and SOD were significantly (p < 0.01) decreased in EAC control group when compared with the normal control group. Administration of MECC (50 and 100 mg/kg) significantly (p < 0.01) raised the CAT, reduced GSH and SOD levels as compared with EAC control animals ().
Discussion
The Ehrlich ascites tumor implantation induces a local inflammatory reaction, with increasing vascular permeability, which results in an intense edema formation, cellular migration, and a progressive ascites fluid accumulation (Bala et al., Citation2010). The ascites fluid is essential for tumor growth, since it constitutes a direct nutritional source for tumor cells (Dolai et al., Citation2012a). The present study showed that MECC significantly decreased the tumor volume, tumor weight, viable tumor cell and increased the non-viable tumor cell and lifespan of the EAC bearing control mice. The reliable criteria for judging the value of any anticancer drug are prolongation of lifespan (Dolai et al., Citation2012b). The observed increase in the lifespan of tumor bearing mice by reduction of nutritional fluid volume and seization of the tumor growth is a positive result and further corroborates the antitumor potential of MECC.
Usually in cancer chemotherapy, the major problems are myelosuppression and anemia. The anemia encountered in tumor bearing mice is mainly due to reduction in RBC or Hb percentage, and this may occur either due to iron deficiency or due to hemolytic or myelopathic conditions (Haldar et al., Citation2011). Pharmacotherapy with MECC replenishes the RBC and Hb content to the normal levels. The WBC count was reduced as compared with that of EAC control mice. These indicating parameters revealed that MECC exerted less toxic effect to the hemopoietic system and plausibly had selective affinity to the tumor cell and thereby it could maintain the normal hematological profile. It is evident from the result that MECC possesses a protective action on hemopoietic system.
Enzymes in serum have been studied as both early possible indicators of neoplasia and as an aid in monitoring the progression and regression of disease. In certain circumstances serum enzymes can be carcinogenic and may engender hepatoxicity (Dolai et al., Citation2012b). Elevated levels of serum parameters, i.e., SGPT, SGOT and ALP which are indicative of impaired liver functions can be clearly seen due to hepatotoxicity associated after 9 d of inoculation with EAC cells (Haldar et al., Citation2011). The analysis of changes in serum total protein in malignancy is in itself a means of studying abnormality in the protein metabolism in this condition. Treatment with the MECC restored the elevated biochemical parameters more or less to normal range, indicating the protection of the tumor cell induced hepatotoxicity by MECC.
Carbonylation of protein often leads to a loss of protein function, which is considered a widespread marker of severe oxidative stress, damage and disease-derived protein dysfunction. Significantly elevated plasma level of PC is found in various types of cancer including hematological malignancies and neurodegenerative diseases as well as in human cell lines (Dalle-Donne et al., Citation2006). The proposed mechanism for high levels of PC is that myeloid cells are the major source of free radicals which lead to irreversible protein oxidation in the cells (Dolai et al., Citation2012a). Administration of MECC restored the increased PC levels more or less to normal range signifying the antioxidant and free radical scavenging property of MECC.
Oxidative stress may lead to damage of the macromolecules such as lipids and can induce lipid peroxidation in vivo (Dolai et al., Citation2012a). In EAC bearing mice, the level of lipid peroxide in liver was significantly raised, which was however reduced near to normal level in MECC treated animal groups. This reflects the decline in free radical production and subsequent reduction in oxidative stress, which are the main risk factors of the ailment. GSH, a potent inhibitor of neoplastic process plays an important role as an endogenous antioxidant system that is found particularly in high concentration in liver and is known to have key function in the protective process (Haldar et al., Citation2010). The level of reduced GSH was depleted in cancer bearing mice, which may be due to its utilization, by the excessive amount of free radicals. Treatment with MECC was found to increase the GSH content in the liver and kidney as compared to EAC control animals.
Cells are also equipped with enzymatic antioxidant mechanisms that play an important role in the elimination of free radicals. SOD is involved in the clearance of superoxide. The inhibition of SOD activities as a result of tumor growth was reported (Gupta et al., Citation2000) and similar findings were observed in our present results in EAC bearing mice. The administration of MECC at both doses significantly recovered the SOD level towards normal level. On the other hand, the free radical scavenging enzyme CAT is present in all oxygen-metabolizing cells and its function is to provide a direct defense against the potentially damaging reactivities of superoxide and hydrogen peroxide. The inhibition of CAT activities as a result of tumor growth has also been reported (Dolai et al., Citation2012a). Similar findings were observed in the present investigation with EAC bearing mice. The administration of MECC at both doses increased the CAT levels, which along with the restoration of lipid peroxide and GSH contents to near normal indicate the antioxidant and free radical scavenging property of MECC.
Conclusion
The present investigation is quite encouraging as it explored the potential antitumor activity of MECC presumably potentiated by its direct cytotoxic effect and antioxidant property. It was assumed that attenuation of oxidative stress in different tissues in EAC bearing mice decreased the viability of EAC cells. Phytochemical study showed the presence of steroids, tannins, saponins, volatile oil, proteins alkaloids and flavonoids in MECC; these compounds have been known to possess potent antitumor properties. Finally, our findings suggest that the MECC exhibits potential antitumor and antioxidant activities which enlighten a novel source of phytomedicines in free radical and tumor biology. Further investigations are in progress in our laboratory to identify the active principle(s) responsible for these antitumor and antioxidant activities.
Declaration of interest
The authors report no conflict of interest. The authors alone are responsible for the content and writing of the paper. The authors are thankful to the AICTE (RPS-File No: 8023/BOR/RID/RPS (NER)-91/2010-11), New Delhi, India, for financial assistance for the project.
Acknowledgements
The authors greatly acknowledge Department of Pharmaceutical Technology, Jadavpur University, Kolkata.
References
- Abdullaev FI. (2002). Cancer chemopreventive and tumoricidal properties of saffron (Crocus sativus L.). Exp Biol Med 227:20–5
- Abraham P, Wilfred G, Catherine SP. (1999). Oxidative damage to the lipids and proteins of the lungs, testis and kidney of rats during carbon tetrachloride intoxication. Clin Chim Acta 289:177–9
- Aebi H. (1974). Catalase. In: Packer L, ed. Methods in Enzymatic Analysis. New York: Academic Press, 673--84
- Amalraj VA, Velayudhan KC, Muralidharan VK. (1989). A note on the anomalous flowering behaviour in Curcuma caesia (Zingiberaceae). J Bombay Nat History Soc 86:278–9
- Arulmozhi DK, Sridhar N, Veeranjaneyulu A, Arora SK. (2006). Preliminary mechanistic studies on the smooth muscle relaxant effect of hydro alcoholic extract of Curcuma caesia. J Herb Pharmacother 6:117–24
- Bala A, Kar B, Haldar PK, et al. (2010). Evaluation of anticancer activity of Cleome gynandra on Ehrlich’s ascites carcinoma treated mice. J Ethnopharmacol 129:131–4
- Banerjee A, Nigam SS. (1976). Antifungal activity of the essential oil of Curcuma caesia Roxb. Indian J Med Res 64:1318–21
- Chandrashekhar G, Joshi M, Gopal N, Kumari S. (2011). Antitumor activity of hexane and ethyl acetate extracts of Tragia involucrata. Int J Cancer Res 7:267–77
- Cragg GM, Newman DJ. (2005). Plants as a source of anticancer agents. J Ethnopharmacol 100:72–9
- Craker LE, Simon JE. (1996). Herb, Spices and Medicinal Plants: Recent Advances in Botany, Horticulture and Pharmacology. New York: Food Products Press
- D’Armour FE, Blood FR, Belden DA. (1965). The Manual for Laboratory Work in Mammalian Physiology. 3rd ed. Chicago (IL): The University of Chicago Press
- Dalle-Donne I, Aldini G, Carini M, et al. (2006). Protein carbonylation, cellular dysfunction, and disease progression. J Cell Mol Med 10:389–406
- Dolai N, Karmakar I, Kumar RBS, et al. (2012a). Evaluation of antitumor activity and in vivo antioxidant status of Anthocephalus cadamba on Ehrlich ascites carcinoma treated mice. J Ethnopharmacol 142:865–70
- Dolai N, Karmakar I, Kumar RBS, et al. (2012b). Antitumor potential of Castanopsis indica (Roxb. ex. Lindl.) A. DC. leaf extract against Ehrlich’s ascites carcinoma cell. Indian J Exp Biol 50:359–65
- Ellman GL. (1959). Tissue sulfhydryl groups. Arch Biochem Biophys 82:70–7
- Gupta M, Mazumder UK, Rath N, Mukhopadhyay DK. (2000). Antitumor activity of methanolic extract of Cassia fistula L. seed against Ehrlich ascites carcinoma. J Ethnopharmacol 72:151–6
- Haldar PK, Bhattacharya S, Dewanjee S, Majumder UK. (2011). Chemopreventive efficacy of Wedelia caledulaceae against 20-methylcholanthrene-induced carcinogenesis in mice. Environ Toxicol Pharmacol 31:10–15
- Haldar PK, Kar B, Bala A, et al. (2010). Antitumor activity of Sansevieria roxburghiana rhizome against Ehrlich ascites carcinoma in mice. Pharm Biol 48:1337–43
- Harborne JB. (1998). Phytochemical Methods, A Guide to Modern Techniques of Plant Analysis. New Delhi: Springer (India) Pvt. Ltd
- Kakkar P, Das B, Vishwanath PN. (1984). A modified spectrophotometric assay of superoxide dismutase. Indian J Biochem Biophys 21:130–2
- Karmakar I, Dolai N, Bala A, Haldar PK. (2011a). Anxiolytic and CNS depressant activities of methanol extract of Curcuma caesia. Pharmacologyonline 2:738–47
- Karmakar I, Dolai N, Saha P, et al. (2011b). Scavenging activity of Curcuma caesia rhizome against reactive oxygen and nitrogen species. Orient Pharm Exp Med 11:221–8
- Karmakar I, Saha P, Neelanjan S, et al. (2011c). Neuropharmacological assessment of Curcuma caesia rhizome in experimental animal models. Orient Pharm Exp Med 11:251–5
- Karthikeyan R, Karthigayan S, Balasubashi MS, et al. (2007). Antitumor effect of snake venom (Hydrophis spiralis) on Ehrlich ascites carcinoma bearing mice. Int J Cancer Res 3:167–73
- Lorke DA. (1983). A new approach to practical acute toxicity testing. Arch Toxicol 54:275–87
- Ohkawa H, Onishi N, Yagi K. (1979). Assay for lipid peroxidation in animal tissue by thiobarbituric acid reaction. Anal Biochem 95:351–8
- Paliwal P, Pancholi SS, Patel RK. (2011). Pharmacognostic parameters for evaluation of the rhizomes of Curcuma caesia. J Adv Pharm Technol Res 2:56–61
- Rath M. (2001). Cellular Health Series: Cancer. 1st ed. Santa Clara: M R Publishing, Inc
- Sarangthem K, Haokip MJ. (2010). Bioactive components in Curcuma caesia Roxb. grown in Manipur. Bioscan 5:113–15
- Singh V, Jain AP. (2003). Ethnobotany and Medicinal Plants of India and Nepal. Jodhpur: Scientific Publishers
- Wintrobe MM, Lee GR, Boggs DR, et al. (1961). Clinical Hematology. 5th ed. Philadelphia: Lea and Febiger