Abstract
Context: Abnormal obesity and the related diseases, such as diabetes and cardiovascular disease, are the main causes of mortality, around the world. A key feature of the adipogenesis and obesity is angiogenesis-dependent tissue growth accompanied with extracellular remodeling. In this way, suppression of angiogenesis may be a key point for preventing the adipogenesis.
Objective: In the present study, to provide a deeper insight to understand obesity and screening for more effective therapeutics, we have developed a three-dimensional in vitro model of microvessel formation under collagen matrix culture using endothelial cells, extracted from a suitable tissue.
Materials and methods: In a successful approach for developing an angiogenesis model, the rat mesenteric microvascular endothelial cells (RMMECs) were isolated, coated on dextran beads and then suspended in collagen gel. Additionally, the proliferation as well as migration of endothelial cells were analyzed and compared with human umbilical vein endothelial cells (HUVECs).
Results: RMMECs showed remarkable migration ability and had higher growth during the logarithmic growth phase, when compared with HUVECs. Also, no significance differences in morphogenesis were observed between HUVECs and RMMECs.
Discussion and conclusion: The model may be useful in providing insights to develop potential intervention strategies in vivo against obesity-related disorders. Targeting endothelial cells is an interesting and exciting possibility that may be raised in further investigations.
Introduction
Angiogenesis, which is accompanied with adipose tissue remodeling, solid tumor development, wound healing and other physiological/pathophysiological conditions, is a multi-step process, including matrix degradation and endothelial cell sprouting followed by cell migration, proliferation, alignment, tube formation and anastomosis to other vessels (Bauer et al., Citation2005). Many natural inducers/inhibitors are known to influence angiogenesis but relatively little is known about the mechanism of angiogenesis as well as the absolute importance of each factor (Makrilia et al., Citation2009; Ribatti, Citation2009). In previous work, many in vitro, in vivo and ex vivo models of neovascularization were developed to clarify the factors involved, identify the molecular mechanism of angiogenesis and to design therapeutic strategies against tumor progression/growth (Auerbach et al., Citation2003).
Although in vivo assays may better mimic tissue angiogenesis these types of angiogenesis models (Keshavarz et al., Citation2011) involve multiple cell types and have often restricted application for the study of angiogenesis regulation due to interfering inflammatory reactions and potential metabolic processing of regulating agents. On the other hand, in vitro model systems have been developed not only to facilitate the study of angiogenesis stages but also allow for identification of direct effects of potential stimulators or inhibitors on endothelial cell function (Murray, Citation2001). Furthermore, this type of angiogenic assay can be more easily quantified and allow genetic manipulation of endothelial cells. To date, endothelial cells of different vascular origins have been used to develop in vitro models. These cells, which are the primary constituents of new vessels, typically present a cobblestone morphology but under the effect of extended starvation (or when the cells were plated on matrigel). They undergo a morphological differentiation to form a network of branching structures (Jain et al., Citation1997; Staton et al., Citation2006). It is noteworthy that endothelial-based in vitro angiogenesis assays can be easily quantified by measuring length/area of tube-like structures (Vailhe et al., Citation2001).
The most widely used two-dimensional (2-D) assay for endothelial cell angiogenesis/morphogenesis involves plating human umbilical vein endothelial cells (HUVECs), as the most popular cells, on matrigel while collagen/fibrin matrices are used as culture substratum for 3-D assays (Murray, Citation2001). Although many developed in vitro angiogenesis models (Vailhe et al., Citation2001) actually facilitate the study of angiogenesis (and drug development) and potentially reduce the number of challenging in vivo animal experiments, at a much lower frequency, they are used for evaluating a unique physiological process based on the type of endothelial cell. There are several differences among various endothelial cell types, including phenotype, matrix metalloproteinases (MMPs) and growth factor expression and 5′-nucleotidase activity, and they usually behave differently in cell culture assay (Gagnon et al., Citation2002; Jackson & Nguyen, Citation1997). Based on the above statements, the endothelial cell-type used for cell culture-based assay of angiogenesis should be chosen to resemble the tissue of interest as closely as possible.
Ample evidence suggests that obesity/adipogenesis is coupled with angiogenesis and also impaired angiogenesis inhibits fat expansion in mice (Cao, Citation2010; Lijnen, Citation2008). Furthermore, detailed mechanism of angiogenesis in adipose tissue (such as mesenter) remains unclear, primarily, because of the absence of reliable model systems, we set out to develop a suitable in vitro model system to study (and provide deeper insights on) neovascularization of developing adipose tissue using rat mesenteric microvascular endothelial cells (RMMECs). In previous studies, in vitro angiogenesis/proliferation using HUVECs and temporal interrelationship between blood vessel formation and adipogenesis (adipocyte differentiation) were observed (Bouis et al., Citation2001). Since the coupled in vivo adipogenesis/angiogenesis is essential for the differentiation of adipocytes in obesity, it may be envisioned that the application of in vitro angiogenesis models with related endothelial cells, such as mesenteric endothelial cells give more reliable information about actual physiological conditions. Thus, the aim of the present study is to characterize this biologically relevant angiogenesis model, which may be useful in understanding the mechanism of adipogenesis–angiogenesis interrelation and finding an effective strategy for inhibition of obesity.
Materials and methods
Chemicals
Rat tail collagen 2 mg/ml in 0.5 M acetic acid (tendons from rat tail), 10× minimum essential medium (10× MEM) (Gibco, Grand Island, NY), 1.4% (W/V) sodium bicarbonate solution, sterile NaOH solution (1 M), Medium MCD131, fetal bovine serum (FBS) (Gibco), dextran-coated cytodex 3 microcarriers (Amersham Pharmasia Biotech, Piscataway, NJ), rabbit anti-Von Willebrand factor (Sigma, St. Louis, MO), antibody against smooth muscle α-actin (Sigma), endothelial cells from the mesenteric vascular bed, Trypan blue.
Isolation and culture of endothelial cells from the mesenteric vascular bed
This work is approved by the research ethic committee of Kermanshah University of Medical Sciences (KUMS). Isolation and culture of endothelial cells from the mesenteric vascular bed were according to the standard method using enzymatic isolation with modification, after anesthetizing Wistar rats with chloroform and sacrificing by decapitation, carefully dissecting the mesenteric fat containing arteries and veins, then transfer the fat to 50 ml test tubes containing 10 ml collagenase type II enzyme with 1, 2, 4 or 8 mg/ml concentration at 37 °C and incubation for 10, 20, 40 or 60 min in a shaker bath at 37 °C and 90 rpm. Following incubation, test tubes were centrifuged for 5 min and 3000 rpm, aspirate off fat and supernatant, resuspend pellet in 5 ml MCDB131 with 20% FBS and incubate at 37 °C and 5% CO2 (Snead et al., Citation1995). The endothelial cells were identified by immunostaining for the presence of Von-Willebrand factor using rabbit anti-Von Willebrand factor and goat anti-rabbit IgG (FITC conjugated). Human umbilical vein endothelial cells (HUVEC) can be easily isolated by perfusion of the umbilical vein with trypsin. The cells were cultured in MCDB131 medium containing 20% of FBS and passages 3 to 5 were used for the subsequent experiments and cell viability was determined by the Trypan blue exclusion assay.
Primary cell culture
RMMECs and HUVECs were started from frozen stock and grown in MCDB131 medium containing 20% heat-inactivated FBS, penicillin (100 units/ml) and streptomycin (100 µg/ml) in 25 cm2 flasks and maintained at 37 °C with 5% CO2 until 90% confluent. Endothelial cells (ECs) between passages 3 and 5 were used in the cell culture experiments. The animal protocol and ethical considerations were approved by the animal/ethic committee of KUMS.
Cell proliferation assays
Proliferation assays were performed on HUVECs and RMMECs in medium supplemented with 20% FBS. Identical initial cell densities of exponentially growing cells were seeded in 25 cm2 flasks and allowed to attach overnight. Cell proliferation curves were achieved by measuring cell growth for 24 h and 48 h by trypsinization and counting using a coulter counter (KX-21 Sysmex Co, Japan).
Preparation of collagen gels
Type I collagen was solubilized by stirring adult rat tail tendons for 48 h at 4 °C in a sterile 0.02 M acetic acid solution. For collagen gel formation, 7 volumes of cold collagen solution with l volume of 10X minimal essential medium and 2 vol of sodium bicarbonate (l1.76 mg/ml) were mixed in a sterile flask kept on ice to prevent immediate gelation.
Capillary-like tube formation assay
EC (HUVEC and RMMEC) cells were grown in medium supplemented with 20% FBS at 37 °C and 5% CO2 and 3–5 passages were used for this experiment. The cells were mixed with sterilized cytodex-3 microcarriers coated beads with gelatin, at a ratio of 30 EC cells per bead in 1 ml of medium supplemented with 10% heat-inactivated FBS. Beads with cells were shaken gently every 20 min for 8 h at 37 °C and 5% CO2. The mixtures were transferred to four wells of a 24-well tissue culture plate and left for 12–16 h in 1 ml of medium at 37 °C and 5% CO2. The following day, cell-coated beads re-suspended (cultured) in type I collagen matrix and, for gel formation, were placed in 37 °C/5% CO2 incubator as described above. After collagen gel formation, MCDB-131 medium was added to each well. In order to study pseudovascular morphogenesis, capillary-like structures were monitored under a microscope, daily images were captured and then the number of structures were quantified by counting all branches in three random fields from each well.
Cell scratch wound healing assay
Cell migration was evaluated by a wound repair assay according to a previous report (Keshavarz et al., Citation2011). ECs (HUVEC and RMMEC) were cultured in 24-well culture plates. When the cells became confluent, identical straight strip wounds were created using sterile cell scraper. Then the cells were washed with PBS and further incubated with Dulbecco’s modified minimum essential medium (DMEM) containing 2% FBS (the concentration of FBS which allows ECs survival but not cell proliferation). After further incubation (24 h), the ECs were washed twice with PBS and fixed in 4% paraformaldehyde (in PBS) at room temperature. The cells were photographed with a camera connected to an inverted microscope at appropriate magnification. The migration was quantified by measuring the area of the cell that moved beyond the reference line.
Results
Cultured ECs are commonly used to study the mechanism of vascular-related diseases because they play an essential role in the abnormal angiogenesis processes. There are no differences in morphological features and EC-specific marker expression between them so that the isolated rat mesenteric and human umbilical ECs gave a typical cobblestone appearance and had positive reactions to the antibodies against the Von-Willebrand factor, indicating relatively normal basic features of ECs. However, cellular mechanisms characterized in macrovascular endothelium are not necessarily similar or appropriate to extrapolate to the microvascular endothelium and vice versa. For example, insulin exerts a differential modulation of ECs from macrocirculation compared with microcirculation, possibly due to an expression of different insulin receptor isoforms (Sobrevia et al., Citation2011). Additionally, it is demonstrated that the diabetic injury in microvasculature is earlier than that in major vessels in the cardiovascular system. EC characteristics depend on their organ and tissue origin (Bohlen, Citation2004; Michael & Fowler, Citation2008). Micro- and macrovascular ECs not only respond differently to positive/negative modulators of angiogenesis but also express unlike amounts of growth factors, enzymes and receptors. Interestingly, it seems that microvasculature is in general more reactive compared with endothelium from the macrovasculature (Murthi et al., Citation2007; Sobrevia et al., Citation2011; Wang et al., Citation2009) (see also ). Therefore, the characteristics of ECs of different origins may determine the angiogenesis behavior/impairments of micro- and macrovasculature. Due to these reasons, in the present study, we successfully used the RMMEC in the angiogenesis model and also compared it with the HUVEC.
Scheme 1. A schematic representation of two distinct endothelial cell types in adipose tissue. The angiogenesis process which includes endothelial cell growth (proliferation), migration and maturation and leads to vessel formation occurs mainly at the microvasculature level. Differential expression of some growth factors, receptors, enzymes and homeobox genes in various macrovascular and microvascular ECs are also summarized in the table. VEGF, vascular endothelial growth factor; FLK-1, kinase insert domain containing receptor; bFGF, basic fibroblast growth factor; FGFR-1, fibroblast growth factor receptor-1; Ang-1, angiopoietin-1; Tie-1, tyrosine kinase with immunoglobulin-like and EGF-like domain-1; Tie-2, endothelial-specific receptor tyrosine kinase; HLX-1, TLX-1, TLX-2, homeobox genes; Hif-1α, hypoxia-inducible factor-1α (Murakami et al., Citation2001; Murthi et al., Citation2007; Sobrevia et al., Citation2011; Wang et al., Citation2009).
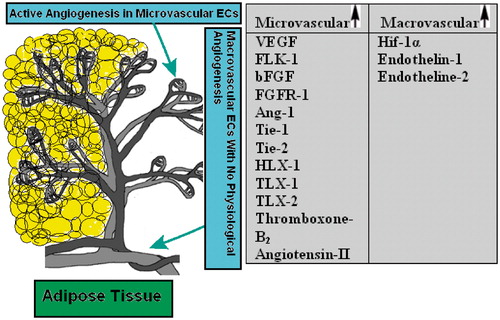
Mesentric endothelial cell isolation
Mesentric ECs were obtained from rat using the collagenase digestion method according to Snead et al. (Citation1995). To find efficient concentrations of collagenase as well as proper incubation time to isolate the single layer of endothelial cells, different doses of collagenase (1 to 8 mg/ml) and different incubation times (from 10 to 60 min) were tested. The results indicated that collagenase at a concentration of 4 mg/ml for 60 min isolates mesenteric endothelial cells, efficiently, with minimal contamination from other cell populations. The viability of separated cells was estimated more than 95% in these conditions by the Trypan blue exclusion assay (data not shown). The endothelial cells isolated using this method displayed viable cells with typical cobblestone morphology and free of contaminating smooth muscle cells (). Furthermore, indirect immunohistochemistry experiments demonstrated positive staining of isolated endothelial cells using an antibody against Von-Willebrand factor (). Additionally, immunohistochemical staining to identify alpha-smooth muscle actin showed no positive results (data not shown).
Figure 1. (A) Phase contrast light photomicrograph of confluent monolayer of cultured rat mesenteric endothelial cells (magnification 400×). (B) Immunofluorescent staining of mesenteric endothelial cells for Von-Willebrand factor (magnification 400×). The data are representative of three independent experiments. Further details are given in experimental procedures.
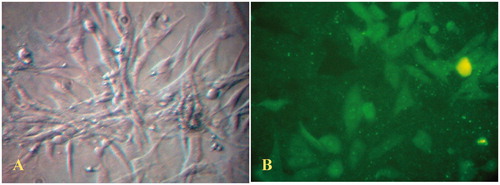
Evaluation of endothelial cell proliferation and migration
Proliferation of endothelial cells is a critical component of angiogenesis process. The results showed that RMMECs display significant growth rate and had higher growth during the logarithmic growth phase, when compared with HUVECs ().
Figure 2. Proliferation kinetics of RMMECs compared with HUVECs. The ECs were synchronized, seeded and allowed to attach overnight in 25 cm2 flasks. Data shown are the mean of ECs number and standard deviations were approximately within 5% of the experimental values of three independent experiments. Further details are given in experimental procedures.
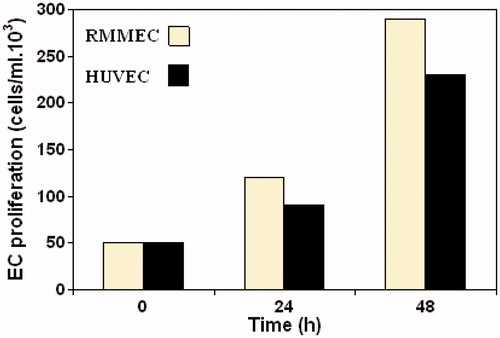
Endothelial cell migration is also critical to processes like wound healing and angiogenesis. A number of methods (such as the wound-healing assay) have been developed to measure cell migration. In the wound-healing assay, a confluent monolayer of cells is ‘‘scratched’’ away, and cell migration is measured on the basis of the amount of time it takes the bordering cells to repopulate the denuded area. To measure the rate of healing, the area between the wound edges is measured and compared relative to the area of the original wound at t = 0. As indicated by and contrary to HUVECs, rat mesenteric endothelial cells showed remarkable migration ability measured by the cell scratch wound healing assay (Brouillet et al., Citation2010; Wang et al., Citation2009) and repopulated the wound, completely, after 24 h.
Figure 3. Migration of RMMECs and HUVECs. The representative images of cell migration in the scratch wound-healing model were evaluated under a light microscope at 900× magnification after 24 h of incubation. Red (top) and green (bottom) vertical lines depicted the initially wounded and remained unoccupied regions, respectively. Data shown are representative of the results of three independent experiments. For interpretation of the references to color in this figure legend, the reader is referred to the web version of this article.
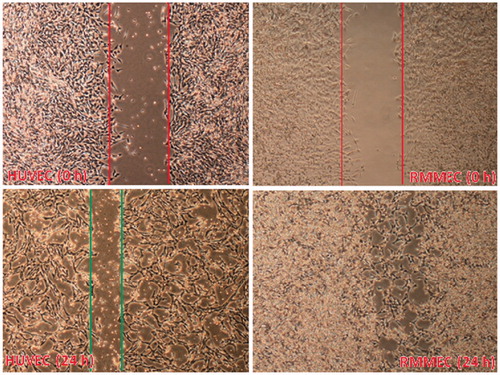
Evaluation of angiogenesis activity (pseudovascular morphogenesis) of isolated endothelial cells
The ability of mesenteric endothelial cells to form tubular structures (on Cytodex matrix) offers an in vitro model of angiogenesis. The first endothelial sprouts were seen to arise from the cytodex explants after 24 h of culture. Within this finite duration, endothelial sprouts progressively invaded the collagen-rich matrix and showed branching/anastomosing morphology. Within ∼3 days of being seeded on carrier, ECs flattened into a reticular network and then form a mesh of capillary-like vessels (). A 3-day-old culture (and older) contained numerous mature microvessels with lumens accompanied with occasional solid endothelial sprouts (). Although, the extent of pseudovascular morphogenesis of ECs is a common criterion for angiogenesis, no significant differences in morphogenesis were observed between HUVECs and RMMECs.
Figure 4. Rat mesenteric endothelial cell for in vitro angiogenesis. (A) Spontaneous formation of capillary-like structures by mesenteric ECs on the microcarriers. Microcarriers seeded with rat mesenteric endothelial cell were embedded in collagen matrix. The endothelial cell attached to particles has been migrated through the collagen matrix (B) total explants area and (C) more details of outgrowth area, in which endothelial cells are seen to form tube-like structures (magnification 400×). The data are representative of 2–3 independent experiments. Further details are given in experimental procedures.
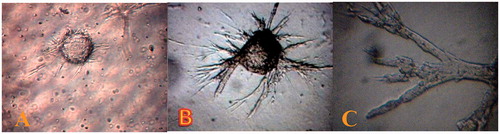
Discussion
Endothelial cells are extensively used in culture to study diverse aspects of endothelial cell biology and angiogenesis. Among the most extensively used endothelial cells of human origin are HUVECs that can easily be isolated by perfusion of the umbilical vein with trypsin and have been successfully cultured since 1973 (Jaffe et al., Citation1973). However, a major drawback of primary endothelial cells is their limited lifespan in culture before they enter senescence. It has been shown that endothelial cells isolated from fresh tissues retain their phenotype for no prolonged time (Bayas et al., Citation2002; Relou et al., Citation1998) allowing for detailed analysis of their functional properties. However, although change in the phenotype of freshly isolated ECs with time is unavoidable, their native gene expression profiles are different from immortal tumor endothelial cells (Griffioen, Citation1997; Madden et al., Citation2004). In this study, we described an in vitro angiogenesis system which allows angiogenic responses of specific endothelial cells to be quantified in a three-dimensional (3D) matrix. One of the most critical technical challenges in studies of angiogenesis is the selection of the appropriate assay. The ideal angiogenesis assay would be fast, easy, robust with reliable readouts. In studying the angiogenic process, it is necessary to develop models of angiogenesis representative of the clinical situation. Animal models are difficult to interpret for routine screening and are relatively expensive (Auerbach et al., Citation2003; Jain et al., Citation1997). Thus, in recent years, a number of in vitro models have been developed in an effort to quantify angiogenesis in 3-D matrices in vitro. Potential angiogenic stimulators and inhibitors may be applied throughout the entire length of culture, or over defined periods in order to observe their impact at various stages of the angiogenic response. In addition to the advantages and limitations of the 3-D assays, described above, types of endothelial cell used should be also taken into consideration. Different in vitro endothelial cell-based assays can be used to evaluate angiogenic as well as anti-angiogenic factors, though the choice of assays may vary depending on which type of (physiologic or synthetic) factor is being tested.
Overweight and especially obesity increases the risk of multiple conditions, including hypertension, cardiovascular diseases, gallstones and osteoarthritis and negatively affect the quality of life (Lijnen, Citation2008). Adipose tissue exhibits extensive vascularity, so that adipogenesis is tightly associated with angiogenesis. It is generally accepted that angiogenesis controls lipogenesis and the growth of adipose tissue, strictly (Cao, Citation2010). Therefore, pharmacologic inhibition of angiogenesis (as well as MMP proteolysis) may have a key role in the control of adipogenesis and impairs adipose tissue development. Assessment of anti-angiogenic (anti-lipogenesis) factors is accomplished using assays in which baseline angiogenesis is inherently high. This can be done using mouse metatarsal- (as well as rat mesenteric-) based assays that provide high baseline levels of angiogenic activity. Cultured mesenteric endothelial cells are similar in many ways to other angiogenic ECs. On the other hand, it has been confirmed that various (arterial/venous) ECs, in different species and tissue types, display different phenotype, angiogenic activity, growth factor receptor and MMP expression, and have also different physiological functions (Akahane et al., Citation2004). Furthermore, they may behave differently in cell culture assays, under baseline or inhibited/stimulated angiogenic conditions.
Mesentric fat as the main representative of visceral adipose tissue may play a significant role in the pathogenesis of metabolic syndrome and cardiovascular diseases (Liu et al., Citation2006). On the other hand, it is metabolically more active than subcutaneous or extra peritoneal fats, and its content directly drains into the portal vein. Development of obesity (increase of mesenteric fat thickness) is associated with extensive modification in adipose tissue involving the rate of adipogenesis, angiogenesis and remodeling of extracellular matrix (ECM). Similar to neoplastic tissues, the growth of adipose tissue is an angiogenesis-dependent process so that this storage tissue is highly vascularized and each adipocyte is nourished by an extensive capillary network. Additionally, it is supported by several reports that adipose tissue mass is sensitive to angiogenesis inhibitors (Lijnen, Citation2008). Thus, taking the above statement into account, the cell type used for the study of lipogenesis (mechanism and inhibitors) should be chosen to resemble the target tissue as closely as possible.
To the best of our knowledge, previous studies on lipogenesis/angiogenesis relationship have mainly employed HUVEC to perform tube formation assay. Since the role of adipocyte-derived angiogenic factors, such as vascular endothelial growth factor-A (VEGF-A), epidermal growth factor-2 (EGF-2) and MMPs has been highlighted in angiogenesis, microvessel maturation and development of adipose tissue, we thought it is more plausible that adipogenesis-related angiogenesis (and its inhibition) can be precisely evaluated using mesenteric ECs (instead of HUVECs). Various ECs are isolated from both macrovascular (e.g., HUVECs) and microvascular (e.g., mesenteric ECs) sources. More importantly, micro- and macro-vascular endothelial cells are different in MMP secretion, cell ultrastructure, lectin-binding properties and VCAM/MHC expression (Auerbach et al., Citation2003; Brouillet et al., Citation2010; Lang et al., Citation2003; Murakami et al., Citation2001) (). Moreover, pathogenic angiogenesis (during tumor and/or obesity neovascularization) mainly encompasses microvascular level of blood vessel system. In this regard, the current model, contrary to HUVEC-based models, can be commonly used in other 2-D/3-D in vitro angiogenesis models using microvascular ECs isolated from mesenter and other suitable tissue sources. Overall, although the VEGF/VEGFR system is always an attractive target to attenuate pathogenic angiogenesis (Chen et al., Citation2010; Tseng et al., Citation2011), it is evident that mRNA levels of VEGF/VEGFR isoforms and neuropilin-1 were not markedly modulated by obesity (Lijnen, Citation2008). Furthermore, since multiple tissue-specific angiogenic factors including PPAR-γ, prohibitin and adipokines (leptin, resistin and adiponectin) either alone or collectively stimulate neovascularization in developing adipose tissue, development of specific anti-angiogenic agents targeting these factors may provide a novel therapeutic option for selective prevention and/or treatment of human obesity and its related disorders with no inhibitory effect on non-pathogenic tissue growth.
Taken together, the present model may allow performance of an extensive series of experiments in vitro including rapid screening of pharmaceuticals and serve as a reproducible and serve as a reliable tool for analyzing angiogenesis inducers/inhibitors, in an easy straightforward manner (Hong et al., Citation2011; Yarani et al., Citation2012). Additionally, it may be envisioned that this model can be applied to compare/discriminate angiogenic responses of endothelial cells extracted from healthy and unhealthy (e.g. Obese) animals.
Declaration of interest
No potential conflicts of interest were disclosed. The authors thank the Research Council of the Kermanshah University of Medical Sciences for financial support of this investigation.
References
- Akahane T, Akahane M, Shah A, et al. (2004). Timp-1 inhibits microvascular endothelial cell migration by MMP-dependent and MMP-independent mechanisms. Exp Cell Res 301:158–67
- Auerbach R, Lewis R, Shinners B, et al. (2003). Angiogenesis assays: A critical overview. Clin Chem 49:32–40
- Bauer SM, Bauer RJ, Velazquez OC. (2005). Angiogenesis, vasculogenesis, and induction of healing in chronic wounds. Vasc Endovascular Surg 39:293–306
- Bayas A, Hummel V, Kallmann BA, et al. (2002). Human cerebral endothelial cells are a potential source for bioactive bdnf. Cytokine 19:55–8
- Bohlen HG. (2004). Mechanisms for early microvascular injury in obesity and type ii diabetes. Curr Hypertens Rep 6:60–5
- Bouis D, Hospers GA, Meijer C, et al. (2001). Endothelium in vitro: A review of human vascular endothelial cell lines for blood vessel-related research. Angiogenesis 4:91–102
- Brouillet S, Hoffmann P, Benharouga M, et al. (2010). Molecular characterization of eg-VEGF-mediated angiogenesis: Differential effects on microvascular and macrovascular endothelial cells. Mol Biol Cell 21:2832–43
- Cao Y. (2010). Adipose tissue angiogenesis as a therapeutic target for obesity and metabolic diseases. Nat Rev Drug Discov 9:107–15
- Chen J, Ran Y, Hong C, et al. (2010). Anti-cancer effects of celecoxib on nasopharyngeal carcinoma hne-1 cells expressing cox-2 oncoprotein. Cytotechnology 62:431–8
- Gagnon E, Cattaruzzi P, Griffith M, et al. (2002). Human vascular endothelial cells with extended life spans: In vitro cell response, protein expression, and angiogenesis. Angiogenesis 5:21–33
- Griffioen AW. (1997). Phenotype of the tumor vasculature; cell adhesion as a target for tumor therapy. Cancer J 10:249–55
- Hong Y, Kim MY, Yoon M. (2011). The anti-angiogenic herbal extracts ob-x from Morus alba, Melissa officinalis, and Artemisia capillaris suppresses adipogenesis in 3t3-l1 adipocytes. Pharm Biol 49:775–83
- Jackson CJ, Nguyen M. (1997). Human microvascular endothelial cells differ from macrovascular endothelial cells in their expression of matrix metalloproteinases. Int J Biochem Cell Biol 29:1167–77
- Jaffe EA, Nachman RL, Becker CG, Minick CR. (1973). Culture of human endothelial cells derived from umbilical veins. Identification by morphologic and immunologic criteria. J Clin Invest 52:2745–56
- Jain RK, Schlenger K, Hockel M, Yuan F. (1997). Quantitative angiogenesis assays: Progress and problems. Nat Med 3:1203–8
- Keshavarz M, Bidmeshkipour A, Mostafaie A, et al. (2011). Anti tumor activity of Salvia officinalis is due to its anti-angiogenic, anti-migratory and anti-proliferative effects. Yakhteh 12:477–82
- Lang I, Pabst MA, Hiden U, et al. (2003). Heterogeneity of microvascular endothelial cells isolated from human term placenta and macrovascular umbilical vein endothelial cells. Eur J Cell Biol 82:163–73
- Lijnen HR. (2008). Angiogenesis and obesity. Cardiovasc Res 78:286–93
- Liu KH, Chan YL, Chan WB, et al. (2006). Mesenteric fat thickness is an independent determinant of metabolic syndrome and identifies subjects with increased carotid intima-media thickness. Diabetes Care 29:379–84
- Madden SL, Cook BP, Nacht M, et al. (2004). Vascular gene expression in nonneoplastic and malignant brain. Am J Pathol 165:601–8
- Makrilia N, Lappa T, Xyla V, et al. (2009). The role of angiogenesis in solid tumours: An overview. Eur J Intern Med 20:663–71
- Michael J, Fowler MD. (2008). Microvascular and macrovascular complications of diabetes. Clin Diabetes 26:77–82
- Murakami S, Morioka T, Nakagawa Y, et al. (2001). Expression of adhesion molecules by cultured human glomerular endothelial cells in response to cytokines: Comparison to human umbilical vein and dermal microvascular endothelial cells. Microvasc Res 62:383–91
- Murray JC. (2001). Angiogenesis Protocols. Totowa (NJ): Humana Press
- Murthi P, So M, Gude NM, et al. (2007). Homeobox genes are differentially expressed in macrovascular human umbilical vein endothelial cells and microvascular placental endothelial cells. Placenta 28:219–23
- Relou IA, Damen CA, van der Schaft DW, et al. (1998). Effect of culture conditions on endothelial cell growth and responsiveness. Tissue Cell 30:525–30
- Ribatti D. (2009). Endogenous inhibitors of angiogenesis: A historical review. Leuk Res 33:638–44
- Snead MD, Papapetropoulos A, Carrier GO, Catravas JD. (1995). Isolation and culture of endothelial cells from the mesenteric vascular bed. Methods Cell Sci 17:257–62
- Sobrevia L, Abarzua F, Nien JK, et al. (2011). Review: Differential placental macrovascular and microvascular endothelial dysfunction in gestational diabetes. Placenta 32:S159–64
- Staton C, Lewis CE, Bicknell RJ. (2006). Angiogenesis Assays: A Critical Appraisal of Current Techniques. Chichester: J. Wiley & Sons
- Tseng FJ, Liu YK, Chung YS, et al. (2011). A fusion protein composed of receptor binding domain of vascular endothelial growth factor-a and constant region fragment of antibody: Angiogenesis antagonistic activity. Cytotechnology 63:285–93
- Vailhe B, Vittet D, Feige JJ. (2001). In vitro models of vasculogenesis and angiogenesis. Lab Invest 81:439–52
- Wang XH, Chen SF, Jin HM, Hu RM. (2009). Differential analyses of angiogenesis and expression of growth factors in micro- and macrovascular endothelial cells of type 2 diabetic rats. Life Sci 84:240–9
- Yarani R, Mansouri K, Mohammadi-Motlagh HR, et al. (2013). In vitro inhibition of angiogenesis by hydroalcoholic extract of oak (Quercus infectoria) acorn shell via suppressing VEGF, MMP-2, and MMP-9 secretion. Pharm Biol 51:361--8