Abstract
Context. Tephrosia toxicaria is currently known as Tephrosia sinapou (Buc'hoz) A. Chev. (Fabaceae) and is a source of compounds such as flavonoids that inhibit inflammatory pain.
Objective: To investigate the analgesic effect and mechanisms of the ethyl acetate extract of T. sinapou in inflammatory pain in mice.
Materials and methods: Behavioral responses were evaluated using mechanical (1–24 h) and thermal hyperalgesia (0.5–5 h), writhing response (20 min) and rota-rod (1–5 h) tests. Neutrophil recruitment (myeloperoxidase activity), cytokines (tumor necrosis factor [TNF]α and interleukin [IL]-1β), aspartate aminotransferase (AST) and alanine aminotransferase (ALT) serum levels were determined by colorimetric assays. Pharmacological treatments were opioid receptor antagonist (naloxone, 0.1–1 mg/kg) and control opioid (morphine, 5 mg/kg). Inflammatory stimuli were carrageenin (100 µg/paw), complete Freund’s adjuvant (CFA, 10 µl/paw), prostaglandin E2 (PGE2, 100 ng/paw) and acetic acid (0.8%).
Results: The intraperitoneal pre-treatment with extract inhibited in a dose-dependent (30–300 mg/kg) dependent manner the mechanical hyperalgesia induced by carrageenin (up to 93% inhibition). The post-treatment (100 mg/kg) inhibited CFA-induced hyperalgesia (up to 63% inhibition). Naloxone (1 mg/kg) prevented the inhibitory effect of the extract over carrageenin-induced mechanical (100%) and thermal (100%) hyperalgesia, neutrophil recruitment (52%) and TNFα (63%) and IL-1β (98%) production, thermal threshold in naïve mice (99%), PGE2-induced mechanical hyperalgesia (88%) and acetic acid-induced writhing response (49%). There was no significant alteration in the rota-rod test, and AST and ALT serum levels by extract treatment.
Discussion and conclusion. Tephrosia sinapou ethyl acetate extract reduces inflammatory pain by activating an opioid receptor-dependent mechanism.
Introduction
The genus Tephrosia includes approximately 400 species distributed in warm regions of both hemispheres (Allen et al., Citation1981). Species of this genus are known by their larvicidal, antiviral, anticancer, hepatoprotective and antioxidant activities (Andel et al., Citation2000; Andrei et al., Citation1997, Citation2000; Jang et al., Citation2003; Khatri et al., Citation2009; Niassy et al., Citation2005; Pezzuto et al., Citation1999; Saleem et al., Citation2001; Vasconcelos et al., Citation2009; Yenesew et al., Citation2006).
Tephrosia sinapou (Buc'hoz) A. Chev. (Fabaceae) (syn. Tephrosia toxicaria) has insecticide and anticancer activities (Arriaga et al., Citation2009b; Arroio et al., Citation2004; Jang et al., Citation2003; Ribeiro et al., Citation2006; Vasconcelos et al., Citation2009). Preliminary data on the composition analysis of ethyl acetate extract of T. sinapou revealed the presence of flavonoids, including novel compounds. In agreement, many species of Tephrosia genus present flavonoids (Arriaga et al., Citation2009a; Arroio et al., Citation2004; Jang et al., Citation2003). There is evidence that isolated flavonoids reduce mechanical and thermal hyperalgesia (e.g., increased response to nociceptive stimuli; pain) by activating the endogenous opioid system (Anjaneyulu & Chopra, Citation2003; Delporte et al., Citation2007; Loscalzo et al., 2008, Citation2011) and inhibition of hyperalgesic cytokines production (Chou et al., Citation2003; Kandhare et al., Citation2012; Valério et al., Citation2009b; Valsecchi et al., Citation2008).
Opioids are effective analgesics presenting a variety of undesirable side effects, thus the activation of endogenous opioid mechanisms could avoid excessive opioid use. In this sense, drugs that activate such endogenous opioid mechanisms could represent useful therapeutic approaches (Carvalho et al., Citation2011; Cunha et al., Citation2010a; Verri et al., Citation2006b). Evidence suggests a relationship between opioids and cytokines since opioids inhibit cytokine-induced mechanical hyperalgesia, cytokine production and the consequent recruitment of neutrophils (Clark et al., Citation2007; Martin et al., Citation2010; Verri et al., Citation2004, Citation2005, 2008b; Wang et al., Citation2005). This is an important mechanism since hyperalgesic cytokines such as tumor necrosis factor α (TNFα) and interleukin (IL)-1β are crucial mediators in pain (Cunha et al., Citation2005, Citation2010b). Their nociceptive effect is consistent in models of overt pain-like behavior, and thermal and mechanical hyperalgesia suggesting that targeting nociceptive cytokines has potential therapeutic application (Verri et al., Citation2006a, Citation2007a). Despite the presence of flavonoids in plants of Tephosia genus and the known effects and mechanisms of flavonoids, no study assessed the analgesic effect of Tephrosia genus plants. Therefore, the present study determines whether T. sinapou ethyl acetate extract treatment inhibits inflammatory pain-like behavior in mice, focusing on its effect on the activation of opioid-dependent mechanisms.
Materials and methods
Animals
The experiments were performed with male Swiss mice (20–25 g, Universidade Estadual de Londrina, Londrina, PR, Brazil) housed in standard clear plastic cages (5 per cage) with free access to food and water. All testing was performed between 9:00 am and 5:00 pm in a temperature-controlled room. Animal care and handling procedures were performed with the approval of the Ethics Committee of the Universidade Estadual de Londrina (OF. CIRC. No 147/2010 – 80/10, process no 31468.2010.84) and followed the International Association for the Study of Pain guidelines as described by Zimmermann (Citation1983). All efforts were made to minimize the number of animals used and their suffering.
Plant material
Tephrosia sinapou specimen was collected in 2009 at the Instituto Agronomico de Campinas (IAC), Sao Paulo, Brazil by S. Myasaka. The identification was performed by A. K. Pastorek in June 2009, and a voucher specimen was deposited at IAC under the number IAC 17211.
Preparation and phytochemical characterization of the extract
The roots of T. sinapou were dried and ground with a knife mill and then the powder (8.9 kg) was subjected to cool exhaustive extraction with ethyl acetate during 10 days with cycles of 48 h. The ethyl acetate extract was exhaustively washed with methanol followed by evaporation (final yield 58.7 g). NMR and mass spectral analysis were used for preliminary phytochemical characterization of T. sinapou ethyl acetate extract. Preliminary phytochemical analysis of T. sinapou ethyl acetate extract by NMR and mass spectral analysis showed the presence of flavonoids: (1) a novel biflavonoid named toxicarine, constituted by glabranine and 5-O-methylnitenin units; (2) flavanone: 7-O-methylglabranine; (3) rotenoids: tephrosin, rotenolone, deguelin, 6-oxo-6a,12a-dehydrodeguelin, 6-oxo-6a,12a-dehydro-α-toxicarol, 6a,12a-dehydrorotenone, rotenonone and villosone; (4) flavanols: tephrowatsin A and quercetol B. Other identified compounds were (1) pterocarpan: flamichapparin B; (2) coumarins: 2,3-dihydro-p-coumaric acid. Two novel compounds were also identified, a substituted benzaldehyde and a chalcone.
Drugs and reagents
Drugs and reagents were obtained from the following sources: carrageenin from FMC Corporation (Philadelphia, PA), Complete Freund’s Adjuvant (CFA), prostaglandin E2, (PGE2), Tween 80, aluminium chlorid, naloxone hydrochloride, hexadecyltrimethylammonium bromide (HTAB) and o-dianisidine dihydrochloride from Sigma Chemical Co. (St. Louis, MO). ELISA kits to determined murine TNFα and IL-1β from eBioscience (San Diego, CA), quercetin from Acros (Pittsburgh, PA), acetic acid and ethanol from F. Maia (Cotia, SP, Brazil), K2HPO4 from Labsynth (Diadema, SP, Brazil), H2O2 from Biotec (Curitiba, PR, Brazil) and morphine sulphate from Cristalia (Itapira, SP, Brazil).
Total flavonoid content
Total flavonoid content was determined using the AlCl3 colorimetric method. To 1.5 ml of T. sinapou ethyl acetate extract solution, 1.5 ml of 2% AlCl3 ethanol solution was added. After 1 h at room temperature, the absorbance was measured at 420 nm. Total flavonoid contents were calculated as quercetin (mg/g) from an analytical curve. The amount of 2% aluminium chloride was substituted by the same amount of distilled water in blank (Georgetti et al., Citation2006).
Experimental protocols
In the first series of experiments, mice were treated with T. sinapou (30–300 mg/kg, i.p. [intraperitoneal], 30 min), morphine (5 mg/kg, i.p. 30 min) or vehicle (20% Tween 80 in saline) before the carrageenin injection (100 µg/paw). The intensity of mechanical hyperalgesia was measured 1–5 h after carrageenin injection by the electronic pressure-meter test. In a model of prolonged inflammation, mice received complete Freund’s adjuvant (CFA) injection (10 µl/paw) or saline (same volume). After 48 h, mechanical hyperalgesia was determined and at 48.5 h mice were treated with T. sinapou (100 mg/kg, i.p.), morphine (5 mg/kg, i.p.) or vehicle. The intensity of mechanical hyperalgesia was measured 48–72 h after CFA injection by the electronic pressure-meter test. To determine the participation of endogenous opioids in the T. sinapou analgesic effect, mice were treated with naloxone (0.1–1 mg/kg, s.c. [subcutaneously], 1 h) before T. sinapou (100 mg/kg, i.p.), morphine (5 mg/kg, i.p.) or vehicle. After an additional 30 min, mice received carrageenin injection (100 µg/paw). The intensity of mechanical and thermal hyperalgesia was measured 3 and 5 h after carrageenin injection by the electronic pressure-meter and hot-plate test. To determine the participation of the inhibition of neutrophil recruitment and opioids in the analgesic effect of the extract, first, mice were treated with vehicle or T. sinapou (100 mg/kg, i.p.) 30 min before carrageenin (100 µg/paw) stimulus and the neutrophil recruitment was evaluated by the myeloperoxidase (MPO) activity at 1, 3 and 5 h. In the following experiment, mice were treated with naloxone (0.1–1 mg/kg, s.c., 1 h) before T. sinapou (100 mg/kg, i.p.), morphine (5 mg/kg, i.p.) or vehicle. After additional 30 min, mice received carrageenin injection (100 µg/paw). The neutrophil recruitment was evaluated by the MPO activity at 5 h after carrageenin injection. To determine the effect of T. sinapou in cytokine (tumor necrosis factor α [TNFα] and interleukin [IL]-1β) production and the dependence on opioid receptors, mice were treated with vehicle or T. sinapou (100 mg/kg, i.p.) 30 min before carrageenin (100 µg/paw) stimulus. Paw skin samples were collected 1, 3 and 5 h after carrageenin injection for ELISA assays to determine TNFα and IL-1β levels. In the following setting, mice were treated with naloxone (1 mg/kg, s.c., 1 h) before T. sinapou (100 mg/kg, i.p.), morphine (5 mg/kg, i.p.) or vehicle treatment. After an additional 30 min, mice received carrageenin (100 µg/paw) stimulus. Paw skin samples were collected 3 h after carrageenin injection, and TNFα and IL-1β levels were determined by ELISA. Possible neuronal effects of the extract were also addressed. Mice were treated with T. sinapou (100 mg/kg, i.p.), morphine (5 mg/kg, i.p.) or vehicle. Another group was treated with naloxone (1 mg/kg, s.c., 1 h) before T. sinapou (100 mg/kg, i.p.). After an additional 30 min, mice received prostaglandin E2 (PGE2) injection (100 ng/paw) and the intensity of mechanical hyperalgesia was measured 1–5 h after PGE2 injection by the electronic pressure-meter test. In a different setting, mice were treated with T. sinapou (100 mg/kg, i.p.), morphine (5 mg/kg, i.p.) or vehicle. Another group was treated with naloxone (1 mg/kg, s.c., 1 h) before T. sinapou (100 mg/kg, i.p.). The intensity of thermal hyperalgesia was measured 0.5–5 h after treatment using a hot plate. A protocol of overt pain-like behavior was used to confirm the results using hyperalgesia tests. Mice were treated i.p. with T. sinapou ethyl acetate extract (1–10 mg/kg, 30 min), morphine (5 mg/kg, i.p., 30 min) or vehicle before i.p. stimulus with acetic acid (0.8% diluted in saline). The writhing score was evaluated during 20 min after stimulus injection. After determining the optimal dose of the extract, in another setting, mice were treated with T. sinapou (10 mg/kg, i.p., 30 min) or vehicle and another group was treated with naloxone (1 mg/kg, s.c., 1 h) before T. sinapou (10 mg/kg, i.p., 30 min) before i.p. stimulus with acetic acid (0.8% diluted in saline). The writhing score was evaluated during 20 min after stimulus injection. All results are presented as means ± SEM of experiments performed with five mice per group and are representative of two separated experiments. In all nociception tests, mice were habituated during 1 h in the day before experiment and during 30 min in the day of experiment in the respective apparatus. Experiments were double-blinded.
Electronic pressure-meter test (Mechanical hyperalgesia)
Mechanical hyperalgesia was tested in mice as previously reported (Cunha et al., Citation2004). In a quiet room, mice were placed in acrylic cages (12 × 10 × 17 cm) with wire grid floors, 15–30 min before the start of testing. The test consisted of evoking a hindpaw flexion reflex with a hand-held force transducer (electronic anesthesiometer; IITC Life Science, Woodland Hills, CA) adapted with a 0.5 mm2 polypropylene tip. The investigator was trained to apply the tip perpendicularly to the central area of the hindpaw with a gradual increase in pressure. The end point was characterized by the removal of the paw followed by clear flinching movements. After the paw withdrawal, the intensity of the pressure was recorded automatically. The value for the response was an average of three measurements. The animals were tested before and after treatment. The results are expressed by delta (Δ) withdrawal threshold (in g) calculated by subtracting the zero-time mean measurements from the mean measurements at indicated time points after stimulus. Withdrawal threshold was 9.2 ± 0.5 g (mean ± SEM; n = 30) before injection of the hyperalgesic agents tested: carrageenin (100 µg/paw), CFA (10 µl/paw) and prostaglandin E2 (100 ng/paw).
Measurement of motor performance
In order to discard possible non-specific muscle relaxant or sedative effects of T. sinapou, the mice motor performance was evaluated on the rota-rod test (Valério et al., Citation2007). The apparatus consisted of a bar with a diameter of 2.5 cm, subdivided into 6 compartments by disks 25 cm in diameter (Ugo Basile, Model 7600). The bar rotated at a constant speed of 22 rotations per min. The animals were selected 24 h previously by eliminating those mice that did not remain on the bar for 2 consecutive periods of 120 s (cut-off time). Motor performance was evaluated at 1.5, 3.5 and 5.5 h after treatment with T. sinapou ethyl acetate extract. These time points are equivalent to times in which mechanical hyperalgesia was evaluated after 30 min of pre-treatment with the extract.
Biochemical analysis
The activities of aspartate aminotransferase (AST) and alanine aminotransferase (ALT) in the serum were determined using commercial kits (Labtest – Lagoa Santa, Minas Gerais, Brazil) according to the manufacture’s instructions.
Hot plate test (Thermal hyperalgesia)
Naïve mice were placed in a 10 cm-wide glass cylinder on a hot plate (IITC Life Science Inc. Woodland Hills, CA) maintained at 52 °C. Two control latencies at least 10 min apart were determined for each mouse. The normal latency (reaction time) was 15 s and maximum latency (cut-off) was set at 30 s to avoid tissue damage. The reaction time was scored when the animal jumped or licked its paws.
Myeloperoxidase activity assay
The myeloperoxidase (MPO) activity assay was performed as previously described using a kinetic colorimetric assay (Casagrande et al., Citation2006). Samples of subcutaneous plantar tissue were collected in 50 mM K2HPO4 buffer (pH 6.0) containing 0.5% hexadecyl trimethylammonium bromide (HTAB) and kept at −80 °C until use. Samples were homogenized using a Polytron (PT3100), centrifuged at 16 100 g for 4 min and the resulting supernatant assayed spectrophotometrically for MPO activity determination at 450 nm (Spectra max, Molecular Devices, Sunnyvale, CA), with 3 readings in 1 min. The MPO activity of samples was compared to a standard curve of neutrophils. Briefly, 10 μl of sample were mixed with 200 μl of 50 mM phosphate buffer pH 6.0, containing 0.167 mg/ml O-dianisidine dihydrochloride and 0.0005% hydrogen peroxide. The results were presented as the MPO activity (number of neutrophils 106/paw).
Cytokine production
At 1, 3 or 5 h after the injection of carrageenin (100 µg/paw), animals were terminally anaesthetized, the skin tissues were removed from the injected and control paws (saline and naive). The samples were homogenized in 500 µl of the appropriate buffer containing protease inhibitors, and TNFα and IL-1β levels were determined as described previously (Verri et al., Citation2010) by enzyme-linked immunosorbent assay (ELISA) kits following the manufacture’s instructions (eBioscience). The results were expressed as picograms (pg) of each cytokine per 100 mg of paw tissue. As a negative control, the concentrations of these cytokines were determined in mice injected with saline.
Writhing response tests
The acetic acid-induced writhing model was performed as previously described (Verri et al., Citation2008a). Acetic acid (0.8% v/v, diluted in saline, 10 ml/kg) or vehicle was injected into the peritoneal cavities of mice. Each mouse was placed in a large glass cylinder and the intensity of nociceptive behavior was quantified by counting the total number of writhes occurring between 0 and 20 min after stimulus injection. The writhing response consisted of a contraction of the abdominal muscle together with a stretching of hind limbs. The intensity of the writhing response was expressed as the cumulative writhing scores over 20 min.
Statistical analysis
Results are presented as means ± SEM of measurements made on five animals in each group, all experiments were performed twice. Two-way analysis of variance (ANOVA) was used to compare the groups and doses at all times (curves) when the hyperalgesic responses were measured at different times after the stimulus injection. The analyzed factors were treatments, time and time versus treatment interaction. When there was a significant time versus treatment interaction, one-way ANOVA followed by Tukey’s t-test was performed for each time. On the other hand, when the hyperalgesic responses were measured once after the stimulus injection, the differences between responses were evaluated by one-way ANOVA followed by Tukey’s t-test. Statistical differences were considered to be significant at p < 0.05.
Results
Total flavonoid content in T. sinapou extract
Total flavonoid content was determined using the aluminium chloride colorimetric method and quercetin as control flavonoid. The extract presented 2.68 ± 0.02 mg of total flavonoids per gram of extract (data not shown in figures). Thus, confirming the presence of flavonoids in the T. sinapou ethyl acetate extract.
Tephrosia sinapou ethyl acetate extract inhibited carrageenin (pre-treatment) and CFA (post-treatment)-induced mechanical hyperalgesia without affecting locomotor activity, and aspartate aminotransferase (AST) and alanine aminotransferase (ALT) serum levels
Tephrosia sinapou ethyl acetate extract (30 mg/kg) inhibited the carrageenin-induced mechanical hyperalgesia only at 5 h after stimulus (). However, the doses of 100 and 300 mg/kg of T. sinapou ethyl acetate extract inhibited the mechanical hyperalgesia induced by carrageenin at 1, 3 and 5 h after stimulus injection with further significant differences at 3 and 5 h compared to the lower dose of 30 mg/kg (). Therefore, the dose of 100 mg/kg of T. sinapou was selected for next experiments. The control drug morphine (an opioid, 5 mg/kg, i.p., 30 min) also inhibited the carrageenin-induced hyperalgesia (). The post-treatment with the extract did not affect CFA-induced mechanical hyperalgesia 30 min after treatment (49 h after CFA injection; ), but significant inhibition of CFA-induced hyperalgesia was observed after 2.5, 4.5 and 6.5 h (51, 53 and 55 h after CFA injection, respectively). After 23.5 h (72 h after CFA injection), there was some residual analgesic effect of the extract, but without statistical difference. The control drug morphine (5 mg/kg, i.p., 30 min) inhibited CFA-induced hyperalgesia (). There was no induction of toxic, anesthetic or muscle relaxing effects that affect motor performance since there was no alteration in the rota-rod test at 1.5, 3.5 and 5.5 h after extract treatment (data no shown), which are equivalent to 1, 3 and 5 after the injection of carrageenin with 30 min of pre-treatment. Furthermore, no signs of hepatic toxicity were detected as determined by the serum levels of AST and ALT 5.5 h after extract treatment (data not shown).
Figure 1. Tephrosia sinapou ethyl acetate extract inhibited carrageenin (pre-treatment) and CFA (post-treatment)-induced mechanical hyperalgesia. Panel A: mice were treated with T. sinapou (30–300 mg/kg, 30 min), morphine (5 mg/kg, i.p. 30 min) or vehicle before the carrageenin injection (100 µg/paw). The intensity of mechanical hyperalgesia was measured 1–5 h after carrageenin injection by the electronic pressure-meter test. Panel B: mice received CFA injection (10 µl/paw) or saline (same volume). After 48 h, mechanical hyperalgesia was determined and at 48.5 h mice were treated with T. sinapou (100 mg/kg, i.p.), morphine (5 mg/kg, i.p.) or vehicle. The intensity of mechanical hyperalgesia was measured 48–72 h after CFA injection by the electronic pressure-meter test. Results are presented as means ± SEM of experiments performed with five mice per group and are representative of two separated experiments. *p < 0.05 compared to the carrageenin (Panel A) or CFA (Panel B) + vehicle group, #p < 0.05 compared to the carrageenin + vehicle group and the dose of 30 mg/kg of extract (one-way ANOVA followed by Tukey’s test).
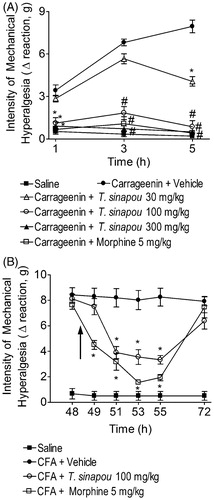
Tephrosia sinapou ethyl acetate extract inhibited carrageenin-induced mechanical and thermal hyperalgesia in an opioid-dependent mechanism
The treatment with the opioid receptor antagonist, naloxone (0.1–1 mg/kg, s.c., 1 h pre-treatment), inhibited in a dose-dependent manner the analgesic effect of T. sinapou extract over carrageenin-induced mechanical () and thermal () hyperalgesia. The dose of 0.1 mg/kg of naloxone prevented the analgesic effect of T. sinapou extract in mechanical hyperalgesia but not thermal hyperalgesia at both time points (3 and 5 h). The doses of 0.3 and 1 mg/kg of naloxone inhibited the antinociceptive effect of the extract compared to the lower dose of 0.1 mg/kg at both time points ( and ). The naloxone treatment also inhibited the analgesic effects of the control drug morphine (5 mg/kg, i.p., 30 min) compared to the naloxone negative control group ( and ).
Figure 2. Tephrosia sinapou ethyl acetate extract inhibited carrageenin-induced mechanical and thermal hyperalgesia in an opioid receptor-dependent manner. Mice were treated with naloxone (0.1–1 mg/kg, s.c., 1 h) before T. sinapou (100 mg/kg, i.p.), morphine (5 mg/kg, i.p.) or vehicle. After additional 30 min, mice received carrageenin injection (100 µg/paw). The intensity of mechanical (Panel A) and thermal (Panel B) hyperalgesia were measured 3 (open bars) and 5 (closed bars) h after carrageenin injection by the electronic pressure-meter and hot-plate test. Results are presented as means ± SEM of experiments performed with five mice per group and are representative of two separated experiments. *p < 0.05 compared to the carrageenin positive control vehicle group, #p < 0.05 compared to the naloxone negative control group and fp < 0.05 compared to the naloxone negative control group and the dose of 0.1 mg/kg of naloxone (one-way ANOVA followed by Tukey’s test).
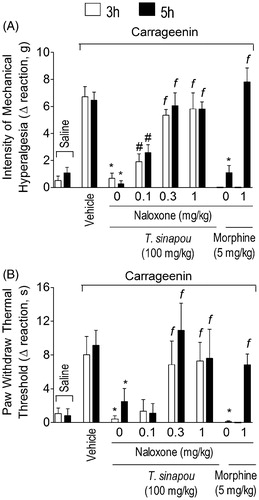
Tephrosia sinapou ethyl acetate extract inhibited in an opioid-dependent manner the increase of MPO activity
The carrageenin stimulus did not induce a significant increase of MPO activity in the paw skin 1 and 3 h after its injection (). On the other hand, a significant increase of MPO activity in paw skin samples was observed 5 h after carrageenin stimulus. Therefore, 5 h was selected as the time for the next experiment to assess a possible relationship between neutrophil recruitment and pain. The treatment with T. sinapou () inhibited carrageenin-induced increase of MPO activity and this effect of T. sinapou was prevented by naloxone (0.1–1 mg/kg, s.c., 1 h of pre-treatment) in a dose-dependent fashion (). The dose of 1 mg/kg of naloxone prevented T. sinapou extract-induced reduction of MPO activity compared to the naloxone negative control group (). Naloxone treatment also prevented the inhibition of myleoperoxidase activity increase by morphine (5 mg/kg, i.p., 30 min) compared to the naloxone negative control group ().
Figure 3. Tephrosia sinapou ethyl acetate extract inhibited neutrophil recruitment (myeloperoxidase activity) by an opioid-dependent mechanism. Panel A: mice were treated with vehicle or T. sinapou (100 mg/kg, i.p.) 30 min before carrageenin (100 µg/paw) stimulus. Panel B: mice were treated with naloxone (0.1–1 mg/kg, s.c., 1 h) before T. sinapou (100 mg/kg, i.p.), morphine (5 mg/kg, i.p.) or vehicle. After additional 30 min, mice received carrageenin injection (100 µg/paw). The neutrophil recruitment was evaluated by the myeloperoxidase activity at 1, 3 and 5 h (Panel A) and at 5 h (Panel B) after carrageenin injection using a kinetic colorimetric assay. Results are presented as means ± SEM of experiments performed with five mice per group and are representative of two separated experiments. *p < 0.05 compared to the carrageenin positive control vehicle group, #p < 0.05 compared to the naloxone negative control group (one-way ANOVA followed by Tukey’s test).
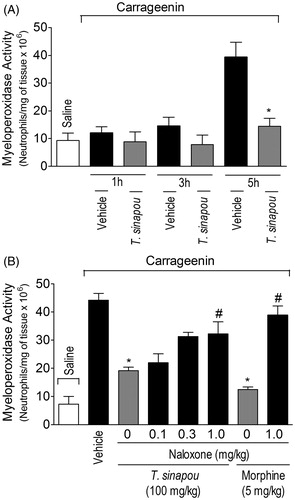
Tephrosia sinapou ethyl acetate extract inhibited carrageenin-induced production of TNFα in the paw skin by an opioid-dependent mechanism
Consistent with previous evidence (Cunha et al., Citation2005), carrageenin induced the production of TNFα in the paw skin 1 and 3 h after its injection while at 5 h non-significant levels were detected (). Carrageenin-induced production of the hyperalgesic cytokine TNFα was inhibited by T. sinapou treatment in the paw skin 1 and 3 h after stimulus injection (). In turn, naloxone (1 mg/kg, s.c., 1 h of pre-treatment) treatment prevented T. sinapou extract inhibitory effect over carrageenin-induced TNFα production in the paw skin at 3 h (). The naloxone treatment also prevented the inhibition of TNFα production by morphine (5 mg/kg, i.p., 30 min) compared to the naloxone negative control group ().
Figure 4. Tephrosia sinapou ethyl acetate extract inhibited carrageenin-induced TNFα production in the paw skin by an opioid-dependent mechanism. Panel A: mice were treated with vehicle or T. sinapou (100 mg/kg, i.p.) 30 min before carrageenin (100 µg/paw) stimulus. Panel B: mice were treated with naloxone (1 mg/kg, s.c., 1 h) before T. sinapou (100 mg/kg, i.p.), morphine (5 mg/kg, i.p.) or vehicle treatment. After additional 30 min, mice received carrageenin (100 µg/paw) stimulus. Paw skin samples were collected 1, 3 and 5 h (Panel A) and at 3 h (Panel B) after carrageenin injection, and TNFα levels were determined by ELISA. Results are presented as means ± SEM of experiments performed with five mice per group and are representative of two separated experiments. *p < 0.05 compared to carrageenin + vehicle group, #p < 0.05 compared to the naloxone negative control group (one-way ANOVA followed by Tukey’s test).
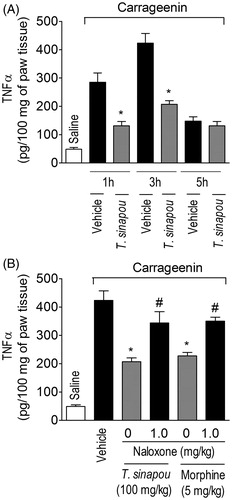
Tephrosia sinapou ethyl acetate extract inhibited carrageenin-induced production of IL-1β in the paw skin by an opioid-dependent mechanism
Carrageenin induced significant production of IL-1β in the paw skin 3 and 5 h after injection without significant production at 1 h after injection (). Carrageenin-induced production of the hyperalgesic cytokine IL-1β was inhibited by T. sinapou treatment in the paw skin 3 and 5 h after stimulus injection (). The treatment with naloxone (1 mg/kg) prevented T. sinapou extract and morphine (5 mg/kg, i.p., 30 min) inhibition of carrageenin-induced IL-1β production in the paw skin 3 h after stimulus injection (). Three hours was chosen to determine the effect of naloxone over the reduction of carrageenin-induced cytokine production provoked by T. sinapou because at this time point there is a significant production of TNFα and IL-1β in the carrageenin model ( and ).
Figure 5. Tephrosia sinapou ethyl acetate extract inhibited carrageenin-induced IL-1β production by an opioid-dependent mechanism. Panel A: mice were treated with vehicle or T. sinapou (100 mg/kg, i.p.) 30 min before carrageenin (100 µg/paw) stimulus. Panel B: mice were treated with naloxone (1 mg/kg, s.c., 1 h) before T. sinapou (100 mg/kg, i.p.), morphine (5 mg/kg, i.p.) or vehicle. After additional 30 min, mice received carrageenin (100 µg/paw) stimulus. Paw skin samples were collected 1, 3 and 5 h (Panel A) and at 3 h (Panel B) after carrageenin injection, and IL-1β levels were determined by ELISA. Results are presented as means ± SEM of experiments performed with five mice per group and are representative of two separated experiments. *p < 0.05 compared to carrageenin + vehicle group, #p < 0.05 compared to the naloxone negative control group (one-way ANOVA followed by Tukey’s test).
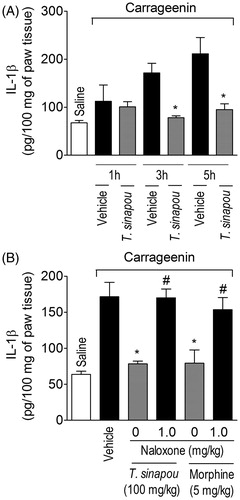
T. sinapou ethyl acetate extract inhibited PGE2-induced mechanical hyperalgesia and increased the thermal nociceptive threshold of naïve mice in the hot plate test in an opioid-dependent mechanism
The ethyl acetate extract of T. sinapou inhibited PGE2-induced mechanical hyperalgesia at all evaluated time points (). The naloxone treatment (1 mg/kg, s.c., 1 h of pre-treatment) significantly prevented the analgesic effect of T. sinapou extract in PGE2-induced mechanical hyperalgesia. Morphine (5 mg/kg, i.p., 30 min) treatment also inhibited PGE2-induced hyperalgesia (). The ethyl acetate extract of T. sinapou induced an increase of thermal threshold of naïve mice in the hot-plate test between 0.5 and 5 h after treatment (), which was prevented by naloxone treatment (1 mg/kg, s.c., 1 h of pre-treatment). Morphine (5 mg/kg, i.p., 30 min) also increased the thermal threshold of naïve mice in the hot plate test ().
Figure 6. Tephrosia sinapou ethyl acetate extract inhibited PGE2-induced mechanical hyperalgesia and increased the thermal nociceptive threshold of naïve mice in the hot-plate test by an opioid-dependent mechanism. Panel A: mice were treated with T. sinapou (100 mg/kg, i.p.), morphine (5 mg/kg, i.p.) or vehicle. Another group was treated with naloxone (1 mg/kg, s.c., 1 h) before T. sinapou (100 mg/kg, i.p.). After additional 30 min, mice received PGE2 injection (100 ng/paw). The intensity of mechanical hyperalgesia was measured 1–5 h after PGE2 injection by the electronic pressure-meter test. Panel B: mice were treated with T. sinapou (100 mg/kg, i.p.), morphine (5 mg/kg, i.p.) or vehicle. Another group was treated with naloxone (1 mg/kg, s.c., 1 h) before T. sinapou (100 mg/kg, i.p.). The intensity of thermal hyperalgesia was measured 0.5–5 h after treatment using a hot plate. Results are presented as means ± SEM of experiments performed with five mice per group and are representative of two separated experiments. *p < 0.05 compared to the PGE2 + vehicle group (Panel A) or vehicle group (Panel B), #p < 0.05 compared to the T. sinapou group (one-way ANOVA followed by Tukey’s test).
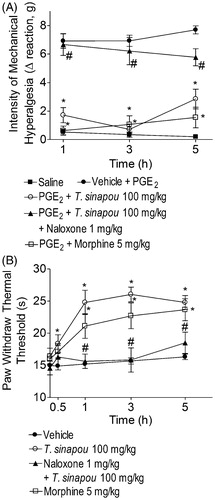
Tephrosia sinapou ethyl acetate extract inhibited acetic acid-induced writhing response by an opioid-dependent mechanism in mice
Tephrosia sinapou ethyl acetate extract inhibited in a dose-dependent manner the acetic acid-induced writhing response. It was observed significant inhibition with the doses of 3 and 10 mg/kg/i.p. of T. sinapou extract and no effect with the lower dose of 1 mg/kg (). The effect of the dose of 10 mg/kg of T. sinapou extract was greater than the dose of 3 mg/kg (). Therefore, the dose of 10 mg/kg of T. sinapou extract was chosen for the next experiment. The treatment with naloxone (1 mg/kg) prevented the inhibition of acetic acid-induced writhing response by T. sinapou extract (). Morphine (5 mg/kg, i.p., 30 min) treatment also inhibited the acetic acid-induced writhing response ().
Figure 7. Tephrosia sinapou ethyl acetate extract inhibited acetic acid-induced writhing response in mice by an opioid-dependent mechanism. Panel A: mice were treated i.p. with T. sinapou ethyl acetate extract (1–10 mg/kg, 30 min), morphine (5 mg/kg, i.p., 30 min) or vehicle before i.p. stimulus with acetic acid (0.8% diluted in saline). Panel B: mice were treated with T. sinapou (10 mg/kg, i.p., 30 min) or vehicle and another group was treated with naloxone (1 mg/kg, s.c., 1 h) before T. sinapou (10 mg/kg, i.p., 30 min) before i.p. stimulus with acetic acid (0.8% diluted in saline). The writhing score was evaluated during 20 min after stimulus injection. Results are presented as means ± SEM of experiments performed with five mice per group and are representative of two separated experiments. *p < 0.05 compared to the vehicle group, #p < 0.05 compared to the vehicle group, the dose of 1 mg/kg (Panel A) and compared to the naloxone negative control group (Panel B; one-way ANOVA followed by Tukey’s test).
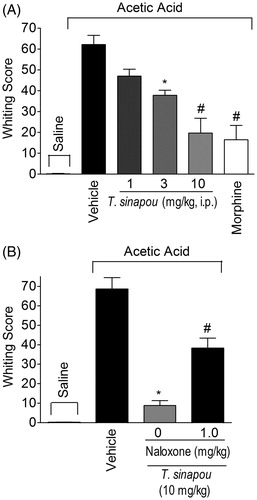
Discussion
Flavonoids are phenolic compounds with analgesic activity (Anjaneyulu & Chopra, Citation2003; Chou et al., Citation2003; Delporte et al., Citation2007; Kandhare et al., Citation2012; Loscalzo et al., 2008, Citation2011; Valério et al., Citation2009b; Valsecchi et al., Citation2008). Preliminary results detected the presence of flavonoids including novel compounds in the Tephrosia sinapou ethyl acetate extract and others have also shown the presence of flavonoids in Tephrosia genus plants (Arriaga et al., Citation2009a; Arroio et al., Citation2004; Jang et al., Citation2003). However, there was no previous demonstration of analgesic activity for T. sinapou extract. Herein, we provide the first evidence that T. sinapou ethyl acetate extract inhibits mechanical and thermal hyperalgesia by a mechanism related to the activation of opioid receptors with two possible and distinct actions: (i) indirect analgesic effect by peripheral reduction of cytokine production (tumor necrosis factor α [TNFα] and Interleukin [IL]-1β) resulting in reduction of neutrophil recruitment to the paw skin tissue and (ii) direct analgesic effect by activating opioid receptors in peripheral and central nervous systems neurons.
Pre- and post-treatment with T. sinapou ethyl acetate extract reduced the mechanical hyperalgesia in the carrageenin and prostaglandin E2 (PGE2), and complete Freund’s adjuvant (CFA) models, respectively. The carrageenin- and CFA-induced inflammatory hyperalgesia are extensively used models in pre-clinical studies searching for novel drugs and mechanisms of drugs. Therefore, inhibition in these models is an important finding consistent with conceivable applicability. In the carrageenin- and CFA-induced hyperalgesia, there are consistent evidences that cytokines have a crucial role (Cunha et al., Citation2005, Citation2010b; Safieh-Garabedian et al., Citation1995; Verri et al., Citation2007b). In mice, carrageenin induces the sequential production of cytokines such as TNFα and IL-1β (Cunha et al., Citation2005; Valério et al., 2009a; Verri et al., Citation2007b). In this sense, the inhibition of cytokine production is an effective analgesic mechanism in inflammatory pain (Cunha et al., Citation2005, Citation2008a; Verri et al., Citation2007b). In fact, T. sinapou ethyl acetate extract inhibited carrageenin-induced TNFα and IL-1β production.
In particular, the inhibition of PGE2-induced hyperalgesia indicates that the extract components also inhibit the hyperalgesia induced by a direct acting mediator (Valério et al., Citation2009b; Verri et al., Citation2006a). Inflammatory nociceptive mediators can be defined as indirect and direct acting, in which the indirect acting mediators such as some cytokines induce the production of other mediators with direct action in the nociceptors including PGE2. In turn, PGE2 causes nociceptor sensitization by inducing cyclic adenosine monophosphate (cAMP)/cAMP-dependent protein kinase (PKA)-dependent phosphorylation of tetrodotoxin resistant sodium channels lowering the action potential threshold, and facilitating the nociceptor depolarization observed as hyperalgesia (Gold et al., Citation1996a, Citationb; Khasar et al., Citation1998). Drugs such as opioids that increases the intracellular nitric oxide levels to open potassium channels via PKG phosphorilation can inhibit the direct sensitization of nociceptors by PGE2 (Cunha et al., Citation2010a). Opioids inhibit hyperalgesia by three mechanisms: (i) inhibition of adenilate cyclase in neurons, which for instance, counteracts PGE2-induced activation of adenilate cyclase and consequent hyperalgesia; (ii) peripherally by activating the signaling pathway phosphatidylinositol 3-kinase (PI3K)γ/protein kinase B (AKT)/neuronal nitric oxide synthase (nNOS)/nitric oxide (NO)/cyclic guanosine monophosphate-dependent protein kinase (PKG)/ATP-sensitive potassium channel (KATP) causing the hyperpolarization of nociceptors (Cunha et al., Citation2010a); and (iii) inhibition of cytokine production with consequent reduction of hyperalgesia and neutrophil recruitment (Clark et al., Citation2007; Martin et al., Citation2010; Wang et al., Citation2005). It has been demonstrated that neutrophils contribute to the development of pain. In humans, the injection of leukotriene B4 (LTB4) induces hyperalgesia with a kinetic profile similar to the neutrophil recruitment temporal profile (Bisgaard & Kristensen, Citation1985). In animals, LTB4-induced hyperalgesia was inhibited by leukocyte depletion (Levine et al., Citation1984). Recently, it has been demonstrated that neutrophils are important for the hyperalgesia induced by carrageenin, zymosan and antigen-induced inflammation by further producing nociceptive mediators including LTB4 and PGE2 (Cunha et al., Citation2008b; Guerrero et al., Citation2008; Ting et al., Citation2008; Verri et al., Citation2009). On the other hand, it has also been described that neutrophils can reduce hyperalgesia by releasing opioids at the local inflammatory site (Cunha & Verri, Citation2006; Rittner et al., Citation2006). However, considering the T. sinapou extract inhibited the neutrophil recruitment and hyperalgesia, it is unlikely that neutrophils would inhibit the hyperalgesia since their recruitment was damped by the extract.
It is possible that T. sinapou extract induces an opioid-like mechanism involving central and peripheral actions. In agreement, the increase of thermal threshold in the hot-plate test in naïve animals (without inflammation or nerve lesion) indicates supra-spinal mechanisms, which include opioid-dependent mechanisms (Carvalho et al., Citation2011; Le Bars et al., Citation2001). In fact, the extract increased the thermal threshold of naïve mice in the hot-plate test by a mechanism amenable by naloxone (opioid receptor antagonist). Furthermore, the treatment with naloxone prevented the inhibition by the T. sinapou ethyl acetate extract of carrageenin-induced mechanical and thermal hyperalgesia, and the inhibition of acetic acid-induced inflammatory overt pain-like behavior, which could relay on peripheral and central actions (Le Bars et al., Citation2001; Pavão-de-Souza et al., Citation2012). It is noteworthy to mention that the extract was more potent to inhibit the acetic acid-induced writhing response compared to mechanical and thermal hyperalgesia. However, in the writhing test the T. sinapou extract was administrated at the same site of stimulus and in the mechanical and thermal hyperalgesia tests the T. sinapou extract was administrated i.p. and stimuli were injected i.p., therefore, pharmacokinetic factors might explain the possible potency difference. The T. sinapou extract also inhibited the production of TNFα and IL-1β, and neutrophil recruitment (as determined by the MPO activity) to the peripheral inflammatory site by a mechanism amenable by naloxone treatment. Therefore, indicating a peripheral mechanism similar to previous demonstrations for morphine (Clark et al., Citation2007; Martin et al., Citation2010; Wang et al., Citation2005).
Conclusions
This study provides the first pre-clinical evidence of analgesic activity of an extract of Tephrosia genus. The analgesic effect of T. sinapou ethyl acetate extract involves the inhibition of hyperalgesic cytokine production and neutrophil migration by activating an opioid-dependent mechanism. It remains to be determined whether compounds from the T. sinapou extract can activate opioid receptors and/or induce the release of endogenous opioids. We speculate that isolated compounds from Tephrosia genus plants, including T. sinapou, are possible sources of novel analgesic compounds worthy of further investigation.
Declaration of interest
This work was supported by grants from Fundação Araucária, Conselho Nacional de Desenvolvimento Científico e Tecnológico (CNPq), and Coordenadoria de aperfeiçoamento de Pessoal de Nível Superior (CAPES), Brazil. W. A. V. J. received senior research fellowship from CNPq, and MMB and RC received senior research fellowship from Fundação Araucária. The authors are responsible for the content and writing of the article.
Acknowledgements
We appreciated the technical support of Jesus A. Vargas and Pedro S. R. Dionísio Filho.
References
- Allen C, Sievers J, Berry M, Jenner S. (1981). Experimental studies on cerebellar foliation. II. A morphometric analysis of cerebellar fissuration defects and growth retardation after neonatal treatment with 6-OHDA in the rat. J Comp Neurol 20:771–83
- Andel H, Klune G, Andel D, et al. (2000). Propofol without muscle relaxants for conventional or fiberoptic nasotracheal intubation: A dose-finding study. Anesth Analg 91:458–61
- Andrei CC, Ferreira DT, Faccione M, et al. (2000). C-Prenylflavonoids from roots of Tephrosia tunicata. Phytochemistry 55:799–804
- Andrei G, Snoeck R, Vandeputte M, De Clercq E. (1997). Activities of various compounds against murine and primate polyomaviruses. Antimicrob Agents Chemother 41:587–93
- Anjaneyulu M, Chopra K. (2003). Quercetin, a bioflavonoid, attenuates thermal hyperalgesia in a mouse model of diabetic neuropathic pain. Prog Neuropsychopharmacol Biol Psychiatr 27:1001–5
- Arriaga AM, Lima JQ, Vasconcelos JN, et al. (2009a). Unequivocal assignments of flavonoids from Tephrosia sp. (Fabaceae). Magn Reson Chem 47:537–40
- Arriaga AM, Lima JQ, Vasconcelos JN, et al. (2009b). Antioxidant and larvicidal activities of Tephrosia egregia Sandw against Aedes aegypti. Nat Prod Commun 4:529–30
- Arroio A, Honório KM, Weber KC, et al. (2004). A theoretical study on the chemopreventive activity of flavonoid compounds. Internet Electron J Mol Des 3:781–8
- Bisgaard H, Kristensen JK. (1985). Leukotriene B4 produces hyperalgesia in humans. Prostaglandins 30:791–7
- Carvalho TT, Flauzino T, Otaguiri ES, et al. (2011). Granulocyte-Colony Stimulating Factor (G-CSF) induces mechanical hyperalgesia via spinal activation of MAP kinases and PI(3)K in mice. Pharmacol Biochem Behav 98:188–95
- Casagrande R, Georgetti SR, Verri WA Jr, et al. (2006). Protective effect of topical formulations containing quercetin against UVB-induced oxidative stress in hairless mice. J Photochem Photobiol B 84:21–7
- Chou TC, Chang LP, Li CY, et al. (2003). The antiinflammatory and analgesic effects of baicalin in carrageenan-evoked thermal hyperalgesia. Anesth Analg 97:1724–9
- Clark JD, Shi X, Li X, et al. (2007). Morphine reduces local cytokine expression and neutrophil infiltration after incision. Mol Pain 2007: 28–40
- Cunha TM, Roman-Campos D, Lotufo CM, et al. (2010a). Morphine peripheral analgesia depends on activation of the PI3Kgamma/AKT/nNOS/NO/KATP signaling pathway. Proc Natl Acad Sci USA 107:4442–7
- Cunha TM, Talbot J, Pinto LG, et al. (2010b). Caspase-1 is involved in the genesis of inflammatory hypernociception by contributing to peripheral IL-1β maturation. Mol Pain 6:63–73
- Cunha TM, Verri WA Jr. (2006). Neutrophils: Are they hyperalgesic or anti-hyperalgesic? J Leukoc Biol 80:727–8
- Cunha TM, Verri WA Jr, Schivo IR, et al. (2008b). Crucial role of neutrophils in the development of mechanical inflammatory hypernociception. J Leukoc Biol 83:824–32
- Cunha TM, Verri WA Jr, Silva JS, et al. (2005). A cascade of cytokines mediates mechanical inflammatory hypernociception in mice. Proc Natl Acad Sci USA 102:1755–60
- Cunha TM, Verri WA Jr, Valério DA, et al. (2008a). Role of cytokines in mediating mechanical hypernociception in a model of delayed-type hypersensitivity in mice. Eur J Pain 12:1059–68
- Cunha TM, Verri WA Jr, Vivancos GG, et al. (2004). An electronic pressure-meter nociception paw test for mice. Braz J Med Biol Res 37:401–7
- Delporte C, Backhouse N, Inostroza V, et al. (2007). Analgesic activity of Ugni molinae (murtilla) in mice models of acute pain. J Ethnopharmacol 112:162–5
- Georgetti SR, Casagrande R, Vicentini FTMC, et al. (2006). Evaluation of the antioxidant activity of soybean extract by different in vitro methods and investigation of this activity after its incorporation in topical formulations. Eur J Pharm Biopharm 64:99–106
- Gold MS, Reichling DB, Shuster MJ, Levine JD. (1996a). Hyperalgesic agents increase a tetrodotoxin-resistant Na+ current in nociceptors. Proc Natl Acad Sci USA 93:1108–12
- Gold MS, Shuster MJ, Levine JD. (1996b). Role of a Ca(2+)-dependent slow afterhyperpolarization in prostaglandin E2-induced sensitization of cultured rat sensory neurons. Neurosci Lett 205:161–4
- Guerrero AT, Verri WA Jr, Cunha TM, et al. (2008). Involvement of LTB4 in zymosan-induced joint nociception in mice: Participation of neutrophils and PGE2. J Leukoc Biol 83:122–30
- Jang DS, Park EJ, Kang YH, et al. (2003). Potential cancer chemopreventive flavonoids from the stems of Tephrosia sinapou. J Nat Prod 66:1166–70
- Kandhare AD, Raygude KS, Ghosh P, et al. (2012). Neuroprotective effect of naringin by modulation of endogenous biomarkers in streptozotocin induced painful diabetic neuropathy. Fitoterapia 83:650–9
- Khatri A, Garg A, Agrawal SS. (2009). Evaluation of hepatoprotective activity of aerial parts of Tephrosia purpurea L. and stem bark of Tecomella undulata. J Ethnopharmacol 122:1–5
- Khasar SG, Gold MS, Levine JD. (1998). A tetrodotoxin-resistant sodium current mediates inflammatory pain in the rat. Neurosci Lett 256:17–20
- Le Bars D, Gozariu M, Cadden SW. (2001). Animal models of nociception. Pharmacol Rev 53:597–652
- Levine JD, Lau W, Kwiat G, Goetzl EJ. (1984). Leukotriene B4 produces hyperalgesia that is dependent on polymorphonuclear leukocytes. Science 225:743–5
- Loscalzo LM, Wasowski C, Paladini AC, Marder M. (2008). Opioid receptors are involved in the sedative and antinociceptive effects of hesperidin as well as in its potentiation with benzodiazepines. Eur J Pharmacol 580:306–13
- Loscalzo LM, Yow TT, Wasowski C, et al. (2011). Hesperidin induces antinociceptive effect in mice and its aglycone, hesperetin, binds to μ-opioid receptor and inhibits GIRK1/2 currents. Pharmacol Biochem Behav 99:333–41
- Martin JL, Koodie L, Krishnan AG, et al. (2010). Chronic morphine administration delays wound healing by inhibiting immune cell recruitment to the wound site. Am J Pathol 176:786–99
- Niassy B, Lobstein A, Um BH, et al. (2005). Flavonoids from Tephrosia deflexa and T. albifoliolis. Biochem Systemat Eco 33:309–12
- Pavão-de-Souza GF, Zarpelon AC, Tedeschi GC, et al. (2012). Acetic acid- and phenyl-p-benzoquinone-induced overt pain-like behavior depends on spinal activation of MAP kinases, PI(3)K and microglia in mice. Pharmacol Biochem Behav 101:320–8
- Pezzuto JM, Song LL, Lee SK, et al. (1999). Bioassay methods useful for activity-guided isolation of natural product cancer chemopreventive agents. In: Hostettmann K, Gupta MP, Marston A, eds. Chemistry, Biological and Pharmacological Properties of Medicinal Plants from the Americas. Amsterdam, Netherlands: Harwood Academic, 81–110
- Ribeiro WH, Vasconcelos JN, Arriaga AM, et al. (2006). Tephrosia toxicaria pers essential oil: Chemical composition and larvicidal activity. Nat Prod Commun 1:391–4
- Rittner HL, Mousa SA, Labuz D, et al. (2006). Selective local PMN recruitment by CXCL1 or CXCL2/3 injection does not cause inflammatory pain. J Leukoc Biol 79:1022–32
- Safieh-Garabedian B, Poole S, Allchorne A, et al. (1995). Contribution of interleukin-1 beta to the inflammation-induced increase in nerve growth factor levels and inflammatory hyperalgesia. Br J Pharmacol 115:1265–75
- Saleem M, Ahmed Su, Alam A, Sultana S. (2001). Tephrosia purpurea alleviates phorbol ester-induced tumor promotion response in murine skin. Pharmacol Res 43:135–44
- Ting E, Guerrero AT, Cunha TM, et al. (2008). Role of complement C5a in mechanical inflammatory hypernociception: Potential use of C5a receptor antagonists to control inflammatory pain. Br J Pharmacol 153:1043–53
- Valério DA, Cunha TM, Arakawa NS, et al. (2007). Anti-inflammatory and analgesic effects of the sesquiterpene lactone budlein A in mice: Inhibition of cytokine production-dependent mechanism. Eur J Pharmacol 562:155–63
- Valério DA, Ferreira FI, Cunha TM, et al. (2009a). Fructose-1,6-bisphosphate reduces inflammatory pain-like behaviour in mice: Role of adenosine acting on A1 receptors. Br J Pharmacol 158:558–68
- Valério DA, Georgetti SR, Magro DA, et al. (2009b). Quercetin reduces inflammatory pain: Inhibition of oxidative stress and cytokine production. J Nat Prod 72:1975–9
- Valsecchi AE, Franchi S, Panerai AE, et al. (2008). Genistein, a natural phytoestrogen from soy, relieves neuropathic pain following chronic constriction sciatic nerve injury in mice: Anti-inflammatory and antioxidant activity. J Neurochem 107:230–40
- Vasconcelos JN, Lima JQ, Lemos TLG, et al. (2009). Estudo químico e biológico de Tephrosia toxicaria Pers. Quím Nova 15:1–5
- Verri WA Jr, Cunha TM, Ferreira SH, et al. (2007b). Role of IL-15 in antigen-induced neutrophil migration by triggering IL-18 production. Eur J Immunol 37:3373–80
- Verri WA Jr, Cunha TM, Magro DA, et al. (2008a). Role of IL-18 in overt pain-like behaviour in mice. Eur J Pharmacol 588:207–12
- Verri WA Jr, Cunha TM, Magro DA, et al. (2009). Targeting endothelin ETA and ETB receptors inhibits antigen-induced neutrophil migration and mechanical hypernociception in mice. Naunyn Schmiedebergs Arch Pharmacol 379:271–9
- Verri WA Jr, Cunha TM, Parada CA, et al. (2006a). IL-15 mediates immune inflammatory hypernociception by triggering a sequential release of IFN-gamma, endothelin, and prostaglandin. Proc Natl Acad Sci USA 103:9721–5
- Verri WA Jr, Cunha TM, Parada CA, et al. (2006b). Hypernociceptive role of cytokines and chemokines: Target for analgesic drug development? Pharmacol Ther 112:116–38
- Verri WA Jr, Cunha TM, Poole S, et al. (2007a). Cytokine inhibitors and pain control. Rev Bras Reumatol 47:341–53
- Verri WA Jr, Guerrero AT, Fukada SY, et al. (2008b). IL-33 mediates antigen-induced cutaneous and articular hypernociception in mice. Proc Natl Acad Sci USA 105:2723–8
- Verri WA Jr, Molina RO, Schivo IR, et al. (2005). Nociceptive effect of subcutaneously injected interleukin-12 is mediated by endothelin (ET) acting on ETB receptors in rats. J Pharmacol Exp Ther 315:609–15
- Verri WA Jr, Schivo IR, Cunha TM, et al. (2004). Interleukin-18 induces mechanical hypernociception in rats via endothelin acting on ETB receptors in a morphine-sensitive manner. J Pharmacol Exp Ther 310:710–17
- Verri WA Jr, Souto FO, Vieira SM, et al. (2010). IL-33 induces neutrophil migration in rheumatoid arthritis and is a target of anti-TNF therapy. Ann Rheum Dis 69:1697–703
- Wang J, Barke RA, Charboneau R, Roy S. (2005). Morphine impairs host innate immune response and increases susceptibility to Streptococcus pneumoniae lung infection. J Immunol 174:426–34
- Yenesew A, Kiplagat JT, Derese S, et al. (2006). Two unusual rotenoid derivatives, 7a-O-methyl-12a-hydroxydeguelol and spiro-13-homo-13-oxaelliptone, from the seeds of Derris trifoliate. Phytochemistry 67:988–91
- Zimmermann M. (1983). Ethical guidelines for investigations of experimental pain in conscious animals. Pain 16:109–10