Abstract
Context. Sargassum has been used in traditional Chinese medicine since the eighth century AD to treat goiter. Sargassum wightii Greville (Sargassaceae) is a major source of alginic acid used widely in food and drug industries.
Objective: To evaluate the anti-Alzheimer potential of S. wightii through evaluation of antioxidant and cholinesterase inhibitory activities.
Materials and methods: Successive extraction was done using solvents of varying polarity. Solvent extracts (100–500 µg/mL) were employed for all the antioxidant assays. Free radical scavenging activity was evaluated by 2,2-diphenyl-1-picrylhydrazyl, OH•, H2O2 radical scavenging assay. The reducing power of the seaweed was evaluated by ferric reducing antioxidant power and reducing power assay. Cholinesterase (ChE) inhibitory activity was evaluated and the Km, Vmax and Ki were calculated. Further, compound characterization was done by GC-MS analysis.
Results: The non-polar extracts were found to possess significant antioxidant activity. At 100 μg/mL, petroleum ether, hexane, benzene and dichloromethane extracts showed significant ChE inhibitory activity with IC50 values of 19.33 ± 0.56, 46.81 ± 1.62, 27.24 ± 0.90, 50.56 ± 0.90 µg/mL, respectively, for AChE, and 17.91 ± 0.65, 32.75 ± 1.00, 12.98 ± 0.31, 36.16 ± 0.64 µg/mL, respectively, for BuChE. GC-MS reveals that 1,2-benzenedicarboxylic acid, diisooctyl ester is the major compound present in dichloromethane extract of S. wightii. The mode of inhibition exhibited by dichloromethane extract against the cholinesterases was found to be competitive type.
Discussion and conclusion: The presence of high amount of terpenoids could be the possible reason for its potential antioxidant and ChE inhibitory activity.
Introduction
Neurodegenerative disorders are chronic and progressive, characterized by selective and symmetric loss of neurons in motor, sensory, or cognitive systems. Most of them have common cellular and molecular pathological mechanisms such as oxidative stress, protein aggregation and formation of inclusion bodies (Ross & Poirier, Citation2004). The central nervous system (CNS), due to high consumption rate of oxygen, abundant lipid content and relative scarcity of antioxidant enzymes, is highly vulnerable to free radical damage when compared to other tissues (Markesbery, Citation1997). The free radicals generated in the cells, upon accumulation, react with macromolecules like lipids, proteins and nucleic acids and damage cellular functions. Moreover, oxidative damage to the cellular components results in alteration of the membrane properties such as fluidity, ion transport, enzyme activities and protein cross-linking eventually resulting in cell death (Chauhan & Chauhan, Citation2006). It is believed that oxidative stress plays a significant role in Alzheimer’s disease (AD) pathogenesis and it has been demonstrated that the increased load of reactive oxygen species in the brain is specifically associated with neuritic plaques (Pereira et al., Citation2005).
AD is a multineurotransmitter deficiency disease, as it involves loss of cholinergic markers like choline acetyltransferase and AChE in the cerebral cortex region. Several studies have demonstrated that the activity of AChE is high around the amyloid plaques and in neurofibrillar tangles bearing neurons. This increase in activity may be caused by the direct effect of Aβ on this enzyme. Furthermore, it has been previously demonstrated that AChE promotes Aβ aggregation. It has also been demonstrated that a remarkable increase in the cortical levels of BuChE is associated with neuritic plaques and neurofibrillary tangles, which are the major neuropathological features of AD (Darvesh et al., Citation2003). Hence, in the current senario, inhibitors of AChE and BuChE have become a major target for the development of therapeutic strategies for AD. The ChE inhibitors directly block the ACh and BuCh hydrolysis thereby promoting the cholinergic functions, which ultimately results in the improvement of cognitive deficit (Rhee et al., Citation2004). There are a few synthetic medicines, like tacrine, donepezil and rivastigmine, for treatment of cognitive dysfunction and memory loss associated with AD. These compounds have been reported to have adverse effects including gastrointestinal disturbances and problems associated with bioavailability, which necessitates the interest in finding better AChE inhibitors from natural resources (Mukherjee et al., Citation2007).
Natural products are a major resource for drug development. A large number of plants, microbes and marine animals have been examined for bioactive secondary metabolites. Seaweeds or marine algae are found to be the potential source of secondary metabolites (Villarreal-Gómez et al., Citation2010). These are primitive nonflowering plants, which are the good source of food and medicine (Kaliaperumal, Citation2003). Various species of Sargassum (Sargassaceae) have been found to possess antiviral, anticancer and antitumor activities (Dias et al., Citation2005). Farnasylacetone derivatives obtained from S. sagamianum Yendo have been found to possess anti-cholinesterase activity (Ryu et al., Citation2003). Recent reports demonstrated that S. thunbergii Kuntze, S. polycystum C.Agardh and S. siliquastrum C.Agardh exhibited potential antioxidant activity (Kim et al., Citation2010). Although many species of seaweeds have been reported to be beneficial for the treatment of various human diseases, only very few have been studied in the treatment of neurological disorders such as AD (Suganthy et al., Citation2009). Therefore, our study evaluates the potential effects of the brown alga, S. wightii, against AD, in particular, its antioxidant and anti-ChE activity.
Materials and methods
Chemicals
DPPH (1,1-diphenyl,2-picrylhydrazyl), 2,4,6-tripyridyl-S-triazine (TPTZ), electric eel AChE (Type-VI-S, EC 3.1.1.7) and horse serum BuChE (EC 3.1.1.8) were purchased from Sigma-Aldrich, St. Louis, MO. Acetylthiocholine iodide (ATCI), butyrylthiocholine iodide (BuTCI) and 5,5′-dithio-bis(2-nitrobenzoic)acid (DTNB) were obtained from Hi-Media Laboratories, Mumbai, India. All other chemicals used were of commercially available highest purity grade.
Seaweed collection
Marine macroalgae S. wightii was collected during low tide (summer) in the South Indian coastal area, Tamil Nadu, and the species was identified according to the references Oza and Zaidu (Citation2003) and Krishnamurthy and Joshi (Citation1970) and further confirmed by Dr. M. Ganesan, Scientist, CSMCRI, Mandapam Camp, Tamil Nadu. The voucher specimen was deposited at the Department of Biotechnology, Alagappa University, under the accession number AUDBTSW20100102.
Solvent extraction in Soxhlet apparatus
The collected seaweeds were processed to remove the attached specimens on its surface. After that, the samples were washed thoroughly at first, in tap water and later in distilled water. To remove the adhered microflora, the seaweeds were washed with alcohol. The processed seaweeds were dried under shade, powdered and stored in airtight zip-lock containers until used for extraction. For extraction, 100 g of dried seaweed was packed in a Soxhlet apparatus and 300 mL of the respective solvents were placed in the solvent reservoir for extraction. The process of successive extraction was carried out for 6 h using solvents of varying polarity, which included petroleum ether, hexane, benzene, dichloromethane, chloroform, ethyl acetate, acetone, methanol and water. After extraction, all the extracts were subjected to re-distillation to remove the solvents from the extracts. Then, the extracts were filtered using Whatman No. 1 filter paper and kept in a dessicator to remove the solvents completely. After drying, the extracts were weighed and stored until used for analysis. The yield of the extracts was calculated using the formula mentioned below:
Antioxidant assays
Assay of DPPH radical scavenging activity
The DPPH assay method is based on the reduction of DPPH•, a stable free radical (Shimada et al., Citation1992). To 333 µL of 0.1 mM DPPH reagent (prepared in methanol), 1 mL of different doses of S. wightii extracts (100–500 µg/mL) in water was added, mixed well, incubated at room temperature for 30 min. The absorbance was read at 517 nm in a UV-visible spectrophotometer (U-2800 model, Hitachi, Japan). BHT (100–500 µg/mL) was used as positive control. The percentage of DPPH• scavenging activity was calculated from the equation mentioned below:
Assay for the scavenging effect on hydroxyl radicals
The hydroxyl radical scavenging activity of various solvent extracts of S. wightii was evaluated by the method of Halliwell et al. (Citation1987). Various solvent extracts of S. wightii was added separately to 1 mL of reaction mixture containing 1 mM EDTA, 10 mM FeCl3, 10 mM deoxyribose, 10 mM H2O2, 1 mM ascorbic acid and 50 mM phosphate buffer (pH 7.4) and incubated at 37 °C for 60 min. After incubation, about 1 mL of the incubation mixture was added to 1 mL of 10% TCA and 1 mL of 0.4% TBA (prepared in glacial acetic acid pH 3.5) which forms a pink chromagen and the absorbance was measured at 532 nm. BHT (100–500 μg/mL) was used as positive control. The percentage of scavenging activity was calculated as mentioned above.
FRAP Assay
The total antioxidative power of S. wightii was assessed by FRAP assay according to the method of Benzie and Strain (Citation1996). Different concentrations of seaweed (100–500 µg/mL) were added separately to 1.5 mL of FRAP reagent (25 mL of 300 mM acetate buffer, pH 3.6, 2.5 mL of 10 mM TPTZ in 40 mM of HCl, 20 mM of ferric chloride solution) and 150 µL of distilled water. Absorbance was measured at 593 nm for 4 min (every 10 seconds). Aqueous solution of known FeSO4 ċ 7H2O (100–500 µg/mL) was used as standard for calibration. The relative activity of the sample was compared with standard ascorbic acid (100–500 µg/mL).
Reducing power
The reducing potential of solvent extracts of S. wightii was determined by the method of Oyaizu (Citation1986). Different concentrations (100–500 µg/mL) of solvent extracts of the seaweed were mixed with 0.5 mL of phosphate buffer (0.2 M, pH 6.6) and 0.5 mL of potassium ferricyanide (1%) and incubated at 50 °C for 20 min. The incubation mixture was then added with 0.5 mL of TCA (10%) and centrifuged at 1000 × g for 10 min. The upper layer was separated and mixed with 0.5 mL of distilled water and 0.1 mL of FeCl3 (0.1%). The absorbance was measured at 700 nm. Ascorbic acid (100–500 µg/mL) was used as a positive control.
Hydrogen peroxide radical scavenging assay
H2O2 scavenging capability of various solvent extracts of S. wightii was determined according to Gulcin et al. (Citation2003). H2O2 solution (40 mM pH 7.4) was added to various concentrations (100–500 µg/mL) of all the solvent extracts of S. wightii. Subsequently, the reaction mixtures were incubated at room temperature for 10 min and the absorbance was measured at 230 nm using phosphate buffer as blank solution. Ascorbic acid (100–500 µg/mL) was used as positive control. The percentage of scavenging activity was calculated as mentioned above.
Cholinesterase inhibitory activity
AChE and BuChE inhibitory activity was evaluated by Ellman et al. (Citation1961) and Ingkaninan et al. (Citation2008). Different concentrations (20–100 µg/mL) of all the solvent extracts of S. wightii were incubated with 10 µL of AChE or BuChE for 45 min at room temperature. To the reaction mixtures, 125 µL of 3 mM DTNB was added and the total volume was made up to 1 mL with Tris-HCl buffer (pH 8.0). Subsequently, 25 µL of 15 mM ATCI or BuTCI was added to the reaction mixtures in order to initiate the enzyme activity. The formation of 5-thio-2-nitrobenzoate anion was detected by yellow coloration and the absorbance was detected in the wavelength of 405 nm using UV-Visible spectrophotometer (U-2800, Hitachi, Japan). The percentage of inhibition was determined by employing the following formula: [(E − S)/E] × 100, where E is the activity of enzyme without test sample and S is the activity of the enzyme with the test sample. The experiments were done in triplicates. Donepezil (currently employed cholinesterase inhibitory drug) was used as a standard.
GC-MS analysis
The components of dichloromethane extract (the extract which showed better antioxidant and ChE inhibition activities) was analyzed by GC-MS (GC Clarus 500 Perkin Elmer) with capillary column of Elite-5MS [(5% diphenyl/95% dimethyl polysiloxane), 30 × 0.25 mm × 0.25 µm df]. The inlet oven temperature was kept at 110 °C initially. The injector temperature was kept at 250 °C. The sample (2 µL) was injected into GC-MS instrument for analysis. For detection, an electron ionization system with ionization energy of 70 eV was used. Helium was used as carrier gas at flow rate of 1 mL/min. The chemical components of the extract were identified by comparing their retention indices (RI) and mass fragmentation patterns with those on the stored NIST library (National Institute of Standards and Technology).
Enzyme kinetics studies
The mechanism of inhibition exhibited by the dichloromethane extract of S. wightii on cholinesterease (both AChE and BuChE) was evaluated by enzyme kinetics studies. The concentration of substrates (ATCI and BTCI) used ranged from 5 to 25 mM. Both AChE and BuChE were incubated with various concentrations of dichloromethane extract of S. wightii with increasing concentration of the substrates. The specific activity of the enzymes was determined according to Ellman et al. (Citation1961). The kinetic parameters like Km, Vmax and Ki were calculated by using the software EZ-Fit model (Perella Scientific Inc, Amherst, NH).
Calculation of IC50
The IC50 value was determined for various solvent extracts of S. wightii by Probit analysis method.
Statistical analysis
Statistical analysis was performed using SPSS 10.0 software package. The results of all the experiments were represented as mean ± SD of triplicates employed. Analysis of variance was performed by one-way ANOVA. Significant differences between means were determined by Duncan’s multiple range tests. A p value <0.05 were regarded as significant and p value <0.01 as very significant.
Results
Successive solvent extraction was performed using different solvents in the order of increasing polarity. The percentage of yield of all the solvent extracts of S. wightii was calculated (data not shown). Water extract exhibited highest yield with the value of 5.7% when compared to other extracts.
Evaluation of in vitro antioxidant activity
Free radical scavenging activity
DPPH free radicals are very stable and it is a widely used method for determining the antioxidant potential in less time period. The scavenging ability was evaluated with various concentrations of different solvent extracts of S. wightii (). The results suggest that among all the extracts, petroleum ether (100%), hexane (100%), benzene (95.90 ± 0.03%), and dichloromethane (100%) extracts exhibited significant scavenging activity (p < 0.05) (at 500 µg/mL) with IC50 values of 73.85 ± 1.50, 93.09 ± 1.88, 229.58 ± 10.33 and 85.83 ± 2.40 µg/mL, respectively. Chloroform, ethyl acetate and acetone extracts showed moderate scavenging ability with IC50 values of 292.68 ± 11.78, 460.16 ± 27.23 and 181.36 ± 4.30 µg/mL, respectively. Methanol and water extracts did not exhibit DPPH radical scavenging activity. Butylated hydroxytoluene (BHT), a standard antioxidant, was used as a positive control, and it showed highest antioxidant activity with an IC50 value of 60.7 ± 1.72 µg/mL.
Determination of reducing power
The reducing power of all the solvent extracts increased with increase in the concentration of the extract (). At 500 µg/mL, hexane, benzene and dichloromethane extracts showed excellent reducing power when compared to other extracts evaluated. Ascorbic acid, a standard antioxidant, showed the highest reducing power.
Evaluation of hydroxyl radical scavenging activity
Hydroxyl radical is an extremely reactive free radical formed in biological systems and has the capacity to cause DNA strand breakage, which contributes to carcinogenesis, mutagenesis and cytotoxicity (Gordon, Citation1990). illustrates that benzene (52.06 ± 0.05%), chloroform (71.94 ± 0.002%) and acetone (54.86 ± 0.003%) extracts showed significant scavenging activity (p < 0.05) with IC50 values of 484.82 ± 14.98, 309.18 ± 19.96 and 394.51 ± 48.21 µg/mL, respectively. However, the scavenging effect of the extract was less when compared to BHT (standard antioxidant) (89.77 ± 0.002%), which showed excellent activity with an IC50 value of 59.18 ± 0.43 µg/mL.
Hydrogen peroxide scavenging assay
The hydrogen peroxide molecule in the cells is rapidly converted into singlet oxygen and hydroxyl radicals, which are the powerful pro-oxidant molecules. Similar to 1O2 and OH•, hydrogen peroxide can also cross the membrane and acts directly on a number of compounds in the cell system (Senevirathne et al., Citation2006). Therefore, the ability of the various solvent extracts of S. wightii to scavenge H2O2 was evaluated through a H2O2 scavenging assay. At a concentration of 500 µg/mL, petroleum ether (100%), benzene (68.32 ± 0.01%) and dichloromethane (59.26%) extracts showed significant scavenging activity (p < 0.05) with IC50 values of 287.79 ± 1.81, 448.8 ± 3.98 and 395.28 ± 0.45 µg/mL, respectively (). Ascorbic acid (standard) showed 100% scavenging activity with an IC50 value of 50 µg/mL.
Evaluation of total antioxidant power
The FRAP assay is based on the ability of antioxidants to reduce Fe3+ to Fe2+ in the presence of TPTZ forming an intense blue Fe2+-TPTZ complex with an absorption maximum at 593 nm. illustrates the total antioxidative power of the different solvent extracts of S. wightii (100–500 µg/mL) in comparison with l-ascorbic acid, which was used as positive control. FeSO4 (200–1000 mM) was used as a standard for calibration. The FRAP value is expressed as millimolar equivalent of Fe(II)/L. The results show that the petroleum ether extract (500 µg/mL) exhibits significant (p < 0.05) ferric reducing capacity (with the absorbance of 0.029 ± 0.003) which is equivalent to absorbance of 100 mM of Fe(II) in FeSO4, which in turn is comparable with the positive control vitamin C (300 µg/mL) (with absorbance of 0.032 ± 0.003). Hexane, benzene, ethyl acetate and acetone extracts showed moderate ferric reducing ability with the absorbance of 0.0085 ± 0.0005, 0.0074 ± 0.001, 0.0084 ± 0.001 and 0.011 ± 0.004, respectively, when compared to control.
Evaluation of cholinesterase inhibitory activity of S. wightii
AChE inhibitory activity of different solvent extracts of S. wightii
Improvement of cholinergic neurotransmission has been considered as one of the potential therapeutic approaches against Alzheimer’s disease. The use of AChE inhibitors (which increases the amount of acetylcholine present in the synapses between cholinergic neurons) has become one among the various therapeutic strategies to enhance the cholinergic functions in the brain (Cho et al., Citation2006). demonstrates the AChE inhibitory activity of various concentrations (20–100 µg/mL) of solvent extracts of S. wightii. Results show that petroleum ether, hexane, benzene, dichloromethane, chloroform and acetone extracts showed inhibitory activity in a concentration dependent manner. At 100 µg/mL, petroleum ether (100 ± 5.9%), hexane (87.21 ± 6.62%), benzene (100 ± 2.2%) and dichloromethane (82.58 ± 1.18%) showed significant AChE inhibitory activity (p < 0.01) with IC50 values of 19.33 ± 0.56, 46.81 ± 1.62, 27.24 ± 0.90 and 50.56 ± 0.90 µg/mL, respectively. Chloroform (73.82 ± 1.2%) and acetone (63.68 ± 3.4%) extracts showed moderate inhibitory activity with IC50 values of 70.90 ± 1.07 and 69.58 ± 3.55 µg/mL, respectively. Donepezil, a standard cholinesterase inhibitor, was used as a positive control, which shows an inhibitory activity of 98.26 ± 0.23% with an IC50 value of 5.09 ± 0.004 µg/mL at the concentration of 50 µg/mL ().
Table 1. AChE and BuChE inhibitory activities of different solvent extracts of S. wightii.
BuChE inhibitory activity of different solvent extracts of S. wightii
The activity of BuChE has been found to be increased during the later stages of plaque maturation, which subsequently results in neuronal degeneration (Guillozet et al., Citation1997). Therefore, the BuChE inhibitory activity of various concentrations of solvent extracts of S. wightii was evaluated. demonstrates the BuChE inhibitory activity of various concentrations (20–100 µg/mL) of solvent extracts of S. wightii. Results show that petroleum ether, hexane, benzene, dichloromethane, ethyl acetate and acetone extracts showed inhibitory activity in a concentration dependent manner. At 100 µg/mL, petroleum ether (98.83 ± 0.30%), hexane (94.16 ± 0.65%), benzene (99.09 ± 0.61%), dichloromethane (98.81 ± 0.17%) and acetone (92.19 ± 0.17%) extracts showed significant BuChE inhibitory activity (p < 0.01) with IC50 values of 17.91 ± 0.65, 32.75 ± 1.00, 12.98 ± 0.31, 36.16 ± 0.64 and 10.49 ± 0.03 µg/mL, respectively. Ethyl acetate extract (53.50 ± 3.63%) showed moderate inhibitory activity with an IC50 value of 72.23 ± 7.23 µg/mL. At 50 µg/mL, the standard donepezil exhibited about 91.57 ± 2.41% BuChE inhibitory activity with an IC50 value of 7.26 ± 0.06 µg/mL ().
Chemical composition of dichloromethane extract of S. wightii
GC-MS analysis of dichloromethane extract of S. wightii identified 10 chemical constituents with the molecular weight ranging from 200 to 400 (). The components were identified as 2,6-dichloro-4-nitro-phenol, methyl 3-(dicholoromethyl)-4,4-dichloro-2-butenoate, 1,5-hexadiene, 1,1,2,5,6,6-hexachloro-, 1,2-benzenedicarboxylic acid, bis (2-methylpropyl) ester, 2-undecanethiol, 2-methyl-, hexanedioic acid, bis(2-ethylexyl) ester, phosphonic acid, phenyl-, bis (trimethylsilyl)ester, thiophene-2-carbonitrile, 5-tert-butyl-3-(-4-chlorobenzylidenamino)-, 1,2-benzenedicarboxylic acid, diisooctyl ester, 2,4,6-cycloheptatrien-1-one, 3,5-bis-trimethylsilyl-. Among the components 1,2-benzenedicarboxylic acid, diisooctyl ester was found to be high with values of 69.62%. Next to that, 1,2-benzenedicarboxylic acid, bis(2-methylpropyl) ester was found to be present in a high amount with the percentage of peak area of 20.14%. Benzene dicarboxylic acid, diisooctyl ester is a triterpenoid with potential antioxidant, antitumor, cancer preventive and immunostimulant activities (Senthilkumar et al., Citation2011).
Table 2. GC-MS analysis of dichloromethane extract of S. wightii.
Determination of the type of inhibition of AChE and BuChE by dichloromethane extract of S. wightii
The results of enzyme kinetic studies reveal that both AChE and BuChE showed maximum enzyme velocity at the concentration of 15 mM. IC50 and IC25 values of dichloromethane extract of S. wightii were employed for studying the kinetic parameters. The values of Km, Vmax and Ki were calculated by plotting Lineweaver–Burk plot. An increase in Km value (1.01 ± 0.49 mM) was observed in the extract-treated sample when compared to control (0.881 ± 0.13 mM) in the case of AChE. However, it did not show any significant change in the Vmax (0.005 ± 0.0002 nmoles/min/mg of protein) when compared to the control (0.0049 ± 0.0001 nmoles/min/mg of protein) (). The Ki value obtained for dichloromethane extract against AChE was 27.07 ± 11.02 µg/mL. Similarly, in the case of BuChE, an increase in the Km value (2.335 ± 0.42 mM) was observed when compared to control (2.274 ± 0.26 mM). The Vmax value of both control and extract treated (0.0028 ± 0.0001 nmoles/min/mg of protein) remains the same (). The Ki value obtained for dichloromethane extract against BuChE was 3.18 ± 1.24 µg/mL. Since the Km value alone is increased without showing any significant alteration in Vmax values the type of inhibition demonstrated by the extract towards AChE and BuChE was determined as competitive type.
Figure 6. (A and C) LB plot of AChE and BuChE with various concentrations of ATCI and BTCI (5–25 mM) as substrates respectively; (B and D) LB plot showing inhibitory activity of dichloromethane extract of S. wightii against AChE and BuChE with ATCI and BTCI as substrates respectively. •– Control; ♦– IC25; ○– IC50; (1/v – Inverse of absorbance measured at 405 nm and 1/s – Substrate concentration).
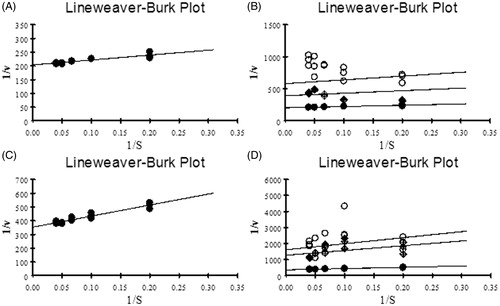
Discussion
Factors such as aging, amyloid plaques and neurofibrillary tangles (NFT) contribute to the stimulation of oxidative stress, which is a major pathologic event in AD. From the past decade, treatment with antioxidants has become a promising approach for slowing the onset and progression of disease. Seaweeds have been found to possess excellent antioxidant capabilities, when checked with various in vitro antioxidant systems (Chew et al., Citation2008; Duan et al., 2006; Kumar et al., Citation2008). Several in vitro studies demonstrated that among various classes of antioxidants, the direct antioxidants are having high neuroprotective potentials. Je et al. (Citation2009) demonstrated that enzymatic extracts of the brown seaweed Undaria pinnatifida Harvey (Alariaceae) have shown significant antioxidant activity, when checked in vitro. The methanol extract of Padina antillarum Kutzing (Dictyotaceae) showed higher ascorbic acid equivalent antioxidant capacity and ferric reducing antioxidant power (Chew et al., Citation2008). Different studies have indicated that the antioxidant effect is related to the development of reductones. These reductones were reported to be the terminators of free radical chain reactions (Wang et al., Citation2009). In the present study, the antioxidant potential of various solvent extracts of S. wightii were evaluated. Among all the extracts evaluated, petroleum ether, hexane, benzene and dichloromethane showed excellent antioxidant activity. More specifically, petroleum ether (reducing power assay) and benzene (FRAP assay) extract showed higher reducing power. Dichloromethane extract showed highest DPPH radical scavenging activity, when compared to other solvent extracts. The hydroxyl radical and hydrogen peroxide radical scavenging activity was checked in vitro. The results suggests that benzene and chloroform extract showed excellent hydroxyl radical scavenging activity and petroleum ether, benzene, dichloromethane extracts showed significant hydrogen peroxide scavenging activity. From the above results, it is apparent that the non-polar solvent extracts of S. wightii possess significant antioxidant and reducing potential.
Numerous studies reveal that AChE, the enzyme involved in the degradation of neurotransmitter acetylcholine, accelerates the aggregation of Aβ peptides resulting in the formation of stable Aβ-AChE complex (Alvarez et al., Citation1997). This complex has been found to act at the synaptic region and alters the normal synaptic functions in the hippocampal neurons. Moreover, the AChE gets infused stereotaxically into CA1 region of the rat hippocampus and induces the formation of plaque-like structures and directly promotes the deposition of Aβ plaques. More recently, it has been demonstrated that Aβ-AChE complexes are more toxic than Aβ fibrils alone and neurons treated with Aβ-AChE complex showed a neurite network more highly disrupted than one treated with Aβ alone (Dinamarc et al., Citation2010). In addition to that, the activity of BuChE has been found to be increased during the later stages of plaque maturation, which subsequently results in neuronal degeneration (Guillozet et al., Citation1997). Hence, in the current scenario, it is vital to develop potential anti-cholinesterase lead compounds to reduce its toxic effects and for the progression of disease. In recent years, the marine algae have been demonstrated as potent cholinesterase inhibitors and lack harmful side-effects (Pangestuti & Kim, Citation2011). Ryu et al. (Citation2003) demonstrated that the farnasylacetone derivatives obtained from S. sagamianum exhibit excellent cholinesterase inhibitory activity (both AChE and BuChE).
In the present study, the cholinesterase inhibitory activity of all the solvent extracts of S. wightii was evaluated. Similar to antioxidant assays, the non-polar solvent extracts exhibit significant cholinesterase inhibitory activity at its least concentration (100 µg/mL). Among all the extracts evaluated, dichloromethane extract showed excellent antioxidant and anti-cholinesterase activity and therefore the dichloromethane extract was employed for kinetic evaluation and characterization of compound. The mode of inhibition of dichloromethane extract on AChE and BuChE was analyzed by computing the kinetic parameters. Since the Km value is altered, not exhibiting any significant change in the velocity of enzyme, the results suggest that both the enzymes were inhibited through competitive inhibition by the dichloromethane extract of S. wightii (Elamin, Citation2003; White & Harmon, Citation2002). The chemical constituents responsible for the antioxidant and anti-cholinesterase activity were evaluated by phytochemical screening and GC-MS analysis. Phytochemical analysis of the dichloromethane extract showed the presence of a high amount of alkaloids and terpenoids (data not shown). To further validate this, the extract was subjected to GC-MS analysis and the results showed the presence of a high amount of triterpene which constitutes about 69.62% among all the components identified. Earlier, it was reported this triterpene has potential antioxidant and cancer preventive activities (Senthilkumar et al., Citation2011), which suggests that the presence of this triterpenoid might be the possible reason for the potential antioxidant and anti-cholinesterase activity. However, a detailed study needs to be undertaken for identifying the bioactive component responsible for the activities in vitro.
Conclusion
The present study evaluates the antioxidant and anticholinesterase activity of the brown seaweed S. wightii. The results demonstrate the dichloromethane extract of S. wightii exhibits strong antioxidant and anti-cholinesterase activity when verified with in vitro models. Moreover, the extract inhibits the enzyme competitively and thereby reduces the activity. GC-MS data implies the presence of high amount of triterpenes in the extract may contribute to the potential activities.
Declaration of interest
The authors declare that there is no declaration of interest. The authors alone are responsible for the content and writing of the paper.
Acknowledgements
The authors gratefully acknowledge the computational and bioinformatics facility provided by the Alagappa University Bioinformatics Infrastructure Facility. K.P.D. wishes to thank UGC, India for offering Major Research Grant. S.A.N. wishes to thank UGC-MANF for the Junior Research Fellowship provided.
The authors gratefully acknowledge the computational and bioinformatics facility provided by the Alagappa University Bioinformatics Infrastructure Facility funded by the Department of Biotechnology, Government of India; Grant No. BT/BI/25/001/2006. K.P.D. acknowledges UGC, India for offering Major Research Grant [F.No.36-6/2008 (SR)].
References
- Alvarez A, Opazo C, Alarcon R, et al. (1997). Acetylcholinesterase promotes the aggregation of amyloid-β-peptide fragments by forming a complex with the growing fibrils. J Mol Biol 272:348–61
- Benzie IFF, Strain JJ. (1996). Ferric reducing ability of plasma (FRAP) as a measure of antioxidant power: The FRAP assay. Anal Biochem 239:70–6
- Chauhan V, Chauhan A. (2006). Oxidative stress in Alzheimer’s disease. Pathophysiology 13:195–208
- Chew YL, Lim YY, Omar M, Khoo KS. (2008). Antioxidant activity of three edible seaweeds from two areas in South East Asia. LWT 41:1067–72
- Cho KM, Yoo ID, Kim WG. (2006). 8-Hydroxydihydrochelerythrine and 8-hydroxydihydrosanguinarine with a potent acetylcholinesterase inhibitory activity from Chelidonium majus L. Biol Pharm Bull 29:2317–20
- Darvesh S, Hopkins D, Geula C. (2003). Neurobiology of butyrylcholinesterase. Nat Rev Neurosci 4:131–8
- Dias PF, Siqueira JM, Vendruscolo LF, et al. (2005). Antiangiogenic and antitumoral properties of a polysaccharide isolated from the seaweed Sargassum stenophyllum. Cancer Chemother Pharmacol 56:436–46
- Dinamarc MC, Sagal JP, Quintanilla RA, et al. (2010). Amyloid-β-acetylcholinesterase complexes potentiate neurodegenerative changes induced by the Aβ peptide. Implications for the pathogenesis of Alzheimer’s disease. Mol Neurodegeneration 5:4
- Duan XJ, Zhang WW, Li X, Wang B. (2006). Evaluation of antioxidant property of extract and fractions obtained from a red alga, Polysiphonia urceolata. Food Chem 95:37–43
- Elamin B. (2003). Dibucaine inhibition of serum cholinesterase. J Biochem Mol Biol 36:149–53
- Ellman G, Courtney KD, Andres JV, Featherstone R. (1961). A new and rapid colorimetric determination of acetylcholinesterase activity. Biochem Pharmacol 7:88–95
- Gordon MH. (1990). The Mechanism of Antioxidant Action In Vitro. London: Elsevier Applied Science
- Guillozet AL, Mesulam MM, Smiley JF, Mash DC. (1997). Butyrylcholinesterase in the life cycle of amyloid plaques. Ann Neurol 42:909–18
- Gulcin I, Emin M, Buyukokuroglu ME, Kufrevioglu OI. (2003). Metal chelating and hydrogen peroxide scavenging effects of melatonin. J Pineal Res 34:278–81
- Halliwell B, Gutteridge JMC, Aruoma OI. (1987). The deoxyribose method: A simple “test-tube” assay for determination of rate constants for reactions of hydroxyl radicals. Anal Biochem 165:215–19
- Ingkaninan K, Best D, Heijden VD, et al. (2008). High-performance liquid chromatography with on-line coupled UV, mass spectrometric and biochemical detection for identification of acetylcholinesterase inhibitors from natural products. J Chromatogr A 872:61–73
- Je JY, Park PJ, Kim EK, et al. (2009). Antioxidant activity of enzymatic extracts from the brown seaweed Undaria pinnatifida by electron spin resonance spectroscopy. LWT -- Food Sci Technol 42:874–8
- Kaliaperumal N. (2003). Products from seaweeds. SDMRI Research Publication; 3, 33–42
- Kim JA, Kong CS, Kim SK. (2010). Effect of Sargassum thunbergii on ROS mediated oxidative damage and identification of polyunsaturated fatty acid components. Food Chem Toxicol 48:1243–9
- Krishnamurthy V, Joshi HY. (1970). Checklist of Indian marine algae. Bhavnagar, India: Central Salt and Marine Chemicals Research Institute, 1–36
- Kumar KS, Ganesan K, Rao PVS. (2008). Antioxidant potential of solvent extracts of Kappaphycus alvarezii (Doty) Doty – An edible seaweed. Food Chem 107:289–95
- Markesbery WR. (1997). Oxidative stress hypothesis in Alzheimer’s disease. Free Radical Biol Med 23:134–47
- Mukherjee PK, Kumar V, Mal M, Houghton PJ. (2007). Acetylcholinesterase inhibitors from plants. Phytomedicine 14:289–300
- Oyaizu M. (1986). Studies on product of browning reaction prepared from glucose amine. Jpn J Nutr 44:307–15
- Oza RM, Zaidu A. (2003). Revised Checklist of Indian Marine Algae. Bhavnagar, India: Central Salt and Marine Chemicals Research Institute, 1–296
- Pangestuti R, Kim SK. (2011). Neuroprotective effects of marine algae. Mar Drugs 9: 803–18
- Pereira C, Agostinho P, Moreira PI, et al. (2005). Alzheimer’s disease- associated neurotoxic mechanisms and neuroprotective strategies. Curr Drug Targets CNS Neurol Disord 4:383–403
- Rhee IK, Appels N, Hofte B, et al. (2004). Isolation of the acetylcholinesterase inhibitor ungeremine from Nerine Bowdenii by preparative HPLC coupled on-line to a flow assay system. Biol Pharm Bull 27:1804–9
- Ross CA, Poirier MA. (2004). Protein aggregation and neurodegenerative diseases. Nat Med 10:S10–17
- Ryu G, Park SH, Kim ES, et al. (2003). Cholinesterase inhibitory activity of two farnesylacetone derivatives from the brown alga Sargassum sagamianum. Arch Pharmacal Res 26:796–9
- Senevirathne M, Kim SH, Siriwardhana N, et al. (2006). Antioxidant potential of Ecklonia Cava on reactive oxygen species scavenging, metal chelating, reducing power and lipid peroxidation inhibition. Int J Food Sci Technol 12:27–38
- Senthilkumar G, Madhanraj P, Panneerselvam A. (2011). Studies on the compounds and its antifungal potentiality of fungi isolated from paddy field soils of Jenbagapuram Village, Thanjavur District, and South India. Asian J Pharm Res 1:19–21
- Shimada K, Fujikawa K, Yahara K, Nakamura T. (1992). Antioxidative properties of xanthin and autooxidation of soybean oil in cyclodextrin emulsion. J Agric Food Chem 40:945–8
- Suganthy N, Pandian SK, Devi KP. (2009). Cholinesterase inhibitors from Sargassum and Gracilaria gracilis: Seaweeds inhabiting South Indian coastal areas (Hare Island, Gulf of Mannar). Nat Prod Res 23:355–69
- Villarreal-Gómez LJ, Soria-Mercado IE, Guerra-Rivas G, Ayala-Sánchez NE. (2010). Antibacterial and anticancer activity of seaweeds and bacteria associated with their surface. Revista de Biología Marina Oceanografía 45:267–75
- Wang BG, Zhang WW, Duan XJ, Li XM. (2009). In vitro antioxidative activities of extract and semi-purified fractions of the marine red alga, Rhodomela confervoides (Rhodomelaceae). Food Chem 113:1101–5
- White JB, Harmon JH. (2002). Interaction of monosulfonate tetraphenyl porphyrin, a competitive inhibitor, with acetylcholinesterase. Biosens Bioelectron 17:463–9