Abstract
Context. Kalanchoe pinnata Lam. (Crassulaceae) is used as a traditional medicine worldwide to treat several ailments, including diabetes. However, the mechanism for the antihyperglycemic action is unknown.
Objective: The present study evaluates the antihyperglycemic and insulin secretagogue potential of Kalanchoe pinnata and assessment of the probable mechanism of action.
Materials and methods: Steam distillate of Kalanchoe pinnata leaves was subjected to solvent fractionation and antidiabetic activity was detected in dichloromethane (DCM) fraction. In the in vivo studies, rats were treated with 5 and 10 mg/kg body weight of DCM fraction for 45 days orally. Lipid profile and other biochemical parameters were estimated. The probable mechanism for insulin secretagogue action was evaluated through studies using diazoxide and nifedipine. The bioactive component from DCM fraction was studied using HPTLC, GCMS and IR.
Results and discussion: Fasting blood glucose values were reduced to 116 mg/dl from 228 mg/dl on treatment with 10 mg/kg body weight of DCM fraction, while glycated hemoglobin improved to 8.4% compared with 12.9% in diabetic controls. The insulin level and lipid profile values were close to normal values. In vitro studies demonstrated a dose-dependent insulin secretagogue action. Insulin secretion was 3.29-fold higher at 10 µg/ml as compared to the positive control. The insulin secretagogue activity was glucose independent and K+-ATP channel dependent. The bioactive component of the DCM fraction was identified to be a phenyl alkyl ether derivative.
Conclusion: The DCM fraction of Kalanchoe pinnata demonstrates excellent insulin secretagogue action and can be useful in treatment of diabetes mellitus.
Introduction
The use of traditional medicines in the treatment of diabetes is widespread. With escalation in the number of diabetics worldwide, the production of drugs for treatment of diabetes is also on the rise (WHO, 1999). Most of the currently used therapy options suffer from multiple side effects. These include weight gain and hypoglycemia for insulin, β-cell burnout for secretagogues (Efanova et al., Citation1998), lactic acidoisis for sensitizers such as metformin (Lalau & Race, Citation1999) and gastric disturbances for α-glucosidase inhibitors (Kimmel & Inzucchi, 2005). However, in the absence of better alternatives, they continue to be used. Due to these side effects of synthetic remedies, there is a growing interest in use of natural remedies in recent years (WHO, 1980). More than 400 plants worldwide have been documented for the treatment of diabetes and a majority of them await proper scientific and medical evaluation (Swanston-Flatt et al., Citation1991). Many times, the exact mechanism of action of the plant remedy used is not known. Toxicity outcomes are not duly addressed and the components responsible for the bioactivity are not identified. It is, therefore, essential to work on traditional therapies to isolate and identify the exact component, determine the mechanism of antidiabetic action, and study toxic side effects, if any.
In the present study, Kalanchoe pinnata Lam. (Crassulaceae), cultivated as a garden plant, was evaluated for its antihyperglycemic action. Kalanchoe pinnata is used as traditional medicine worldwide to treat several ailments. The major reported pharmacological activities of Kalanchoe pinnata are immunosuppressive action (Bergmann et al., Citation1994), hepatoprotective activity (Yadav & Dixit, Citation2003), and antitumor activity (Supratman et al., Citation2001). Kalanchoe pinnata is also reported to lower blood glucose (Ogbonnia et al., Citation2008; Ojewole, Citation2005). The local people of southern Maharashtra, India are known to consume the leaves of Kalanchoe pinnata as they are believed to possess antihyperglycemic activity. Hence, the objective of the present study was to evaluate the antihyperglycemic activity and toxicity effect of Kalanchoe pinnata leaf distillate fractions in steptozotocin-induced diabetic rats as an attempt to determine the probable mechanism of its insulin secretagogue action.
Materials and methods
Plant material
Kalanchoe pinnata (whole plant) was collected in the month of January from Shivaji University, Kolhapur, Maharashtra, India and identified by Prof. S. R. Yadav, Department of Botany, Shivaji University, Kolhapur, Maharashtra, India. The voucher specimen (voucher number SUK-5279) has been deposited in the Department of Botany, Shivaji University, Kolhapur, MS-India.
Extraction of fractions from steam distillate
Fresh, healthy leaves were washed, then crushed in mortar and pestle and filtered through several layers of muslin cloth. The filtrate was subjected to steam distillation at 60 °C. The distillate was subjected to sequential extraction with petroleum ether (pet ether), chloroform followed by dichloromethane (DCM). The distillate remaining after extraction was considered as aqueous fraction. Each fraction extracted was then evaporated in a vacuum rotary evaporator. The yield of pet ether, chloroform, DCM and aqueous fractions was 0.028, 0.066, 0.044 and 0.036% w/w, respectively. All the dried fractions were stored in airtight screw cap glass vials at 4 °C until further use. Each fraction was diluted with 0.05% dimethylsulfoxide (DMSO) just before use. Vehicle control was used in each in vivo and in vitro experiment.
Experimental animals
Male albino Wistar rats weighing about 200 ± 10 g were used in the experimental study. The animals were maintained under standard laboratory conditions. All the animals were allowed free access to standard rat feed (Amruth, Pune, India) and water ad libitum. All the experiments were carried out as per the guidelines of the Institutional Animal Ethical Committee (registration no. 233/CPCSEA).
Induction of diabetes
Normal male albino Wistar rats weighing 200 ± 10 g were fasted overnight and diabetes was induced by intra-peritoneal administration of freshly prepared streptozotocin (65 mg/kg body weight) in 0.1 M citrate buffer (pH 4.5) as previously reported (Kulkarni et al., Citation2012). After 14 days, animals showing stable glycemic values above 200 mg/dl were considered diabetic and selected for further experimentation.
Oral glucose tolerance test (OGTT)
A total of 42 rats (6 normal and 36 diabetic) were fasted overnight with free access to water. Initial blood glucose of each rat was measured with ACCU-CHECK. They were divided into seven groups (n = 6) as normal control, diabetic control, positive control (glibenclamide 2.5 mg/kg body weight), and the remaining four groups were fed with the respective solvent fraction (10 mg/kg body weight). Blood glucose was measured at 0, 30, 60 and 120 min.
In further experiments, four concentrations of bioactive DCM fraction (1.0, 2.5, 5.0 and 10.0 mg/kg body weight) were studied through the OGTT.
In vivo prolonged treatment
For prolonged experiments, a total of 30 male rats (6 normal and 24 diabetic) were divided into five groups as follows:
Group I: Normal control
Group II: Diabetic control
Group III: Diabetic + glibenclamide (2.5 mg/kg body weight)
Group IV: Diabetic + 5 mg DCM fraction/kg body weight
Group V: Diabetic + 10 mg DCM fraction/kg body weight
The glibenclamide or DCM fraction was fed orally to the animals twice a day while control rats were fed with vehicle (0.05% DMSO). The ED50 of the extract was calculated to be 3.75 mg/kg body weight.
Estimation of biochemical parameters
Fasting blood glucose levels of treated rats were estimated by ACCU-CHECK. Blood was collected from the tail tip. At the end of 45 days, all the rats were fasted overnight and sacrificed by cervical dislocation. Glycated hemoglobin, serum cholesterol and high-density lipoprotein cholesterol were estimated by using a biochemical kit from Crest Biosystems, total triglycerides by liquid gold kit, while serum insulin was measured by using insulin ELISA kit from CalBiotech. The amount of insulin released was quantified using an ELISA microplate reader (Multiskan EX, Thermo Scientific) at 450 nm. Liver glycogen levels were estimated by an alkali method (Stetten & Katzen, Citation1961).
Isolation of rat pancreatic islets
The isolation of islets was carried out using a collagenase digestion method modified in our lab (Patil et al., Citation2011). In short, overnight fasted rats were sacrificed by cervical dislocation, and pancreata were removed aseptically. The pancreata were cut into small pieces and washed three-times with Hanks’ balanced salt solution (HBSS) and subjected to collagenase digestion for 20 min with continuous shaking. The dissociation medium consisted of collagenase type V (1 mg/ml) in HBSS supplemented with 10 mM 4-(2-hydroxyethyl)-1-piperazineethanesulfonic acid (HEPES), 2% BSA and 2 mg/ml soyabean trypsin inhibitor (STI). Digestion was stopped by addition of chilled HBSS and tissue digest was centrifuged at 1000 rpm for 2 min at 4 °C. Digested pellet was washed twice with HBSS. Islets were hand-picked under an inverted microscope (LABOMED TC400, Culver City, CA) and seeded in culture flasks containing RPMI 1640 (Hi-Media, Mumbai, India) supplemented with 10% fetal calf serum (Hi-Media, Mumbai, India). The flasks were incubated at 37 °C in a CO2 incubator (Thermo Scientific, Marietta, OH) gassed with 5% CO2. After a 24 h incubation, islets were again hand-picked and used for further studies.
Insulin release assay
Groups of 10 islets were placed in wells each containing 1 ml HBSS (pH 7.4) supplemented with 10 mmol/l HEPES and 2 mg/ml BSA. Cells were then incubated for 1 h with 11.8 mM glucose in the presence or absence of DCM fraction (1.25, 2.5, 5 and 10 µg/ml). Glibenclamide (10 µg/ml) was used as a positive control. To illustrate the probable mechanism of insulin secretion, isolated islets were incubated in the presence of diazoxide or nifedipine with or without the DCM fraction (10 µg/ml). After incubation, the supernatant from each well was collected and stored at −20 °C until further use. The insulin concentration in all the stored samples was determined using an ELISA kit (CalBiotech, CA) and quantified by using an ELISA microplate reader (Multiskan EX, Thermo Scientific, Shanghai, China).
Viability assessment by MTT conversion
The viability of cells after treatment with Kalanchoe pinnata DCM fraction was assessed by a MTT [3-(4,5-dimethylthiazol-2-yl)-2,5-diphenyltetrazolium bromide] assay (Latha et al., Citation2009; Mosman, Citation1983). The absorbance was measured at 570 nm on an ELISA microplate reader (Multiskan EX, Thermo Scientific). The viability of cells was expressed in percent viability (% viability) relative to untreated cells which were considered as 100% viable.
Isolation and characterization of bioactive component from DCM fraction
High performance thin layer chromatography (HPTLC) analysis
HPTLC analysis of DCM fraction was performed by using a HPTLC system (CAMAG, Switzerland). The sample (10 µL) was loaded on pre-coated HPTLC plates (Lichrospher silica gel plate, Merck, NJ), by using spray gas nitrogen and a TLC sample loading instrument (CAMAG LINOMAT 5, Switzerland). The composition of mobile phase used for HPTLC analysis was methanol:glacial acetic acid (95:5 v/v). The spots developed on TLC plates were visualized in a UV chamber and scanned at 280 nm with slit dimension 5.0 × 0.45 mm by using TLC scanner. The results were generated by using HPTLC software WinCATS 1.4.4.6337.
GC-MS analysis
GC-MS analysis of the active fraction was carried out using a GCD-1800 A model (Shimadzu, Kyoto, Japan) of GC-MS; 0.2 μl of sample was injected on a HP-5 column with a starting temperature of 100 °C and a hold time of 2 min at 150–160 °C.
FTIR analysis
An infrared (IR) spectrum of the DCM fraction was carried out using a FTIR spectrometer in the range 450–4000 cm−1 by the KBr pellet technique.
Statistical analysis
All the data obtained were expressed as mean ± SD. Statistical analysis was performed using ANOVA and unpaired Student's t-test. A value of p < 0.05 was considered as statistically significant.
Results
Antihyperglycemic activity of Kalanchoe pinnata fractions
The antihyperglycemic activity of solvent fractions of the leaf distillate was evaluated using the oral glucose tolerance test (OGTT) and results are shown in . Among the four fractions, the DCM fraction showed optimal antihyperglycemic action. The chloroform fraction also showed antihyperglycemic action but is not as effective as the DCM fraction. The remaining two fractions, viz., pet ether and aqueous fractions, were not able to reduce blood glucose levels as compared to glibenclamide control and the DCM fraction group. Hence, further studies were carried out using the DCM fraction.
Table 1. Effect of solvent fractions isolated from Kalanchoe pinnata on blood glucose levels.
The dose-dependent effect of DCM fraction on blood glucose level was evaluated by the OGTT using four different concentrations as 1, 2.5, 5 and 10 mg/kg body weight (Supplementary Table 1). Kalanchoe pinnata DCM fraction showed very good antihyperglycemic activity in a dose-dependent manner. Even at a concentration of 1 mg/kg body weight, the DCM fraction was able to reduce the blood glucose levels as compared to the diabetic control. The antihyperglycemic activity of the fraction at 2.5 mg/kg body weight is nearly comparable to the activity of glibenclamide (at the same dose of 2.5 mg/kg body weight). Increase in the concentration of the DCM fraction further reduces the blood glucose levels in a dose dependent manner. Among four concentrations, the maximum concentration used (10 mg/kg body weight) demonstrated prominent antihyperglycemic activity. The DCM fraction showed a further reduction in the blood glucose values by another 20–30 mg/dl by 4 h; however, no further hypoglycemia or reduction in blood glucose was observed (data not shown).
Long term effect of Kalanchoe pinnata DCM fraction on biochemical parameters
The changes in blood glucose, glycated hemoglobin, serum insulin, liver glycogen and lipid profile of long term treated rats are shown in . After treatment for 45 days, it was observed that Kalanchoe pinnata DCM fraction significantly reduced the fasting blood glucose level as compared to diabetic control. At a dose of 5 mg DCM fraction/kg body weight, there was a reduction in fasting blood glucose levels. However, it was more pronounced for a dose of 10 mg/kg body weight where the blood glucose reached the normal values. There was improvement in serum insulin level and liver glycogen level and decrease in glycated Hb in treated rats as compared to the diabetic controls. The improved insulin levels in long term treated rats may be due to the insulin secretagogue action of the DCM fraction which was verified in the in vitro experiment on isolated islets.
Table 2. Effect of Kalanchoe pinnata DCM fraction on biochemical parameters.
After a 45 days prolonged treatment, the levels of triglycerides (TG), total cholesterol (TC), low-density lipoprotein (LDL) and very low-density lipoprotein (VLDL) were significantly reduced, whereas the level of high-density lipoprotein (HDL) was significantly increased in rats treated with a dose of both 5 mg and 10 mg/kg body weight. The improvement in HDL and decrease in LDL, which is very pronounced as compared to diabetic, would have important significance in prevention of cardiovascular diseases (CVD).
Insulin secretion studies on isolated pancreatic islets
Insulin secretagogue effect of DCM fraction isolated from Kalanchoe pinnata
The insulin secretagogue effect of each fraction isolated from Kalanchoe pinnata was assessed (). Among the four fractions (each at a concentration 10 µg/ml), the DCM fraction showed maximum insulin secretion (133.29 μIU) as compared to other fractions. The insulin secreted due to the action of DCM fraction was comparable to insulin secretion in presence of 10 µg/ml glibenclamide (128.34 μIU). Among the other fractions, the chloroform fraction showed moderate action (68.22 μIU), while pet ether and aqueous fraction showed very little insulin secretagogue action.
Figure 1. Effect of solvent fractions on insulin secretion from isolated rat islets. Insulin secretion induced by 11.8 mM glucose was considered as a negative control and glibenclamide (10 µg/ml) as a positive control. All the extracts were used at a concentration 10 µg/ml. Results are mean ± S.D.; n = 6. *p < 0.05 and **p < 0.001 significant from 11.8 mM glucose control.
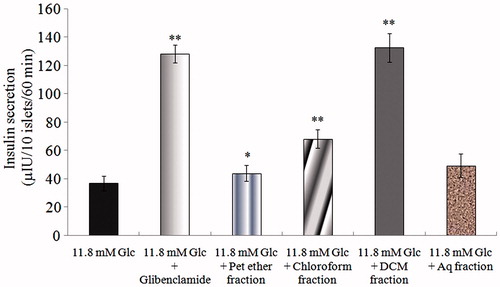
Dose-dependent insulin secretagogue effect of DCM fraction
The dose-dependent effect of DCM fraction (1.25, 2.5, 5 and 10 µg/ml) on in vitro insulin secretion is shown in Supplementary Figure 1. Glibenclamide (10 µg/ml) showed a 3.07-fold increase in insulin secretion compared with the 11.8 mM glucose control. The DCM fraction of Kalanchoe pinnata showed dose-dependent in vitro insulin secretagogue action up to a concentration of 10 µIU/10 islets/60 min. The insulin secretion at 10 µg/ml concentration (126.67 µIU) was almost equal to that of glibenclamide control (118.32 µIU). No significant increase was observed at a higher concentration than 20 µg/ml (data not shown).
The viability of the cells after the treatment is shown in Supplementary Figure 2. The viability of cells was expressed in %viability relative to 11.8 mM glucose control group cells which were considered as 100% viable. Glibenclamide treated islets showed a viability of 94.33%. The viability of islets was not highly affected by 1.25, 2.5, 5 and 10 µg/ml DCM fraction and was 95.00, 96.00, 94.33 and 88.92%, respectively.
Determination of glucose dependent or independent insulin secretagogue action
The effect of basal glucose (2.8 mM) on insulin secretion was evaluated (). At a concentration 10 µg/ml in the presence of 2.8 mM glucose, Kalanchoe pinnata DCM fraction showed a 2.80-fold increase in insulin secretion. The standard control, glibenclamide, showed a 3.17-fold increase in insulin secretion.
Determination of the mechanism for insulin secretagogue action
The effect of diazoxide (K+-ATP channel opener) on insulin secretagogue action of Kalanchoe pinnata DCM fraction is shown in . Insulin secretion in the presence of 11.8 mM glucose is 38.24 µIU. The DCM fraction (10 µg/ml) in presence of 11.8 mM glucose augments insulin secretion to 108.61 µIU. Diazoxide (300 µM) inhibited the insulin secretion of the negative control and insulin secretion dropped from 38.24 to 17.13 µIU. It can be observed that insulin secretion could not be increased by addition of the DCM fraction.
Figure 3. Probable mechanism for insulin secretagogue action of DCM fraction. Effect of 300 µM diazoxide (3A) and 20 µM nifedipine (3B) on insulin secretagogue activity of the Kalanchoe pinnata DCM fraction (10 µg/ml). Results are mean ± S.D.; n = 6. *p < 0.05 and **p < 0.001significant from 2.8 mM glucose control.
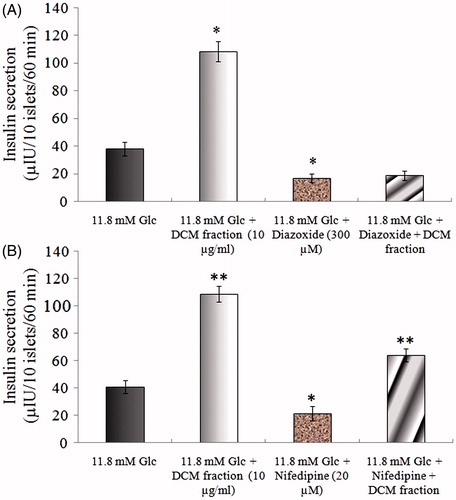
To evaluate Ca2+ dependency for insulin secretion, islets were treated with 11.8 mM glucose in the presence of 20 µM nifedipine with or without the Kalanchoe pinnata DCM fraction (). In the presence of nifedipine (20 µM) with 11.8 mM glucose, there was inhibition in insulin secretion, i.e., insulin secretion dropped from 40.75 to 21.68 µIU. The DCM fraction (10 µg/ml), however, augmented insulin secretion to 64.17 µIU even in presence of nifedipine.
Isolation and characterization of bioactive components
The bioactive DCM fraction was characterized for active chemical constituents. In HPTLC analysis, only two peaks were observed at Rf 0.74 and 0.89 (). Both the fractions were tested for bioactivity. The insulin secretagogue action was found to be present in the major fraction. This major bioactive component at Rf 0.89 was collected by using preparative HPTLC and used for further structural analysis. In GC-MS analysis only a single major peak was observed at a retention time (RT) of 15.478 (). The major ions observed from electron ionization-mass spectra of this peak included ions of m/z 150, 122, 91, 77 and 42 with base peak of 150 (). The fragmentation pattern did not match with the compounds present in a NIST library. Hence, the precise structure of the compound could not be identified. The compound showed IR absorption bands at 3054, 2987, 1598–1422, 1265 and 1033 cm−1 and 746 cm−1 suggesting the presence of aromatic and alkyl C–H stretch, aromatic C–C stretch, a phenyl alkyl ether group and out-of-plane bands in the molecule ().
Figure 4. HPTLC separation of the bioactive DCM fraction. The bioactive DCM fraction was separated by using methanol:glacial acetic acid (95:5 v/v). Only two peaks were observed at Rf 0.74 and 0.89.
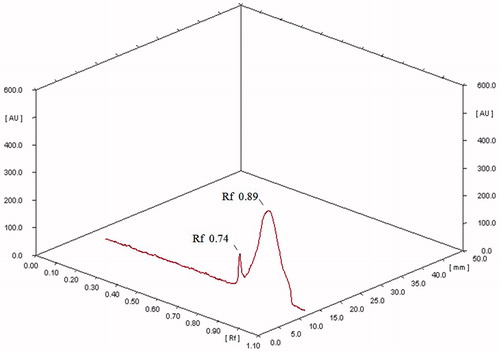
Discussion
Kalanchoe pinnata is routinely used in traditional medicines in several regions of the world for the treatment of a number of diseases. Kalanchoe pinnata has been previously reported for its antihyperglycemic effect (Ogbonnia et al., Citation2008; Ojewole, Citation2005). Another species of Kalanchoe, viz. Kalanchoe crenata (Andr.) Haw (Crassulaceae), was also shown to possess antihyperglycemic activity. Its antihyperglycemic mechanism was proposed to be due to modulation in insulin sensitivity (Kamgang et al., Citation2008).
Though traditionally used in treatment of several ailments and diseases, Kalanchoe species are reported to be cardiotoxic to cattle and sheep. The toxicity problem is more in South Africa and Australia, where these plants are found in the wild (McKenzie & Dunster, Citation1986). Kalanchoe species shows cardiotoxic effects primarily due to a group of bufadienolide compounds, including bryotoxins, bryophyllins, and bersalgenins (Geof & Volmer, Citation2004).
In the present study, instead of whole leaves extract, a DCM fraction from steam distillate was used that greatly eliminates non-volatile components of whole extract, which may have other activities. The process of distillation also eliminates cardiac glycosides present in plant (Geof & Volmer, Citation2004; Krenn & Kopp, Citation1998) with reported toxicity. The doses used in the present study were much less than reported non-toxic doses (Torres-Santos et al., Citation2003). It was observed that even at a dose of 500 mg/kg body weight given in solitary experiments did not demonstrate lethality or show any behavioral changes in the animal.
The OGTT revealed that the DCM fraction at 10 mg/kg body weight has better blood glucose lowering action than the positive control glibenclamide at 2.5 mg/kg body weight proving its efficacy as a potent insulin secretagogue. Prolonged treatment has demonstrated improved serum parameters indicating its beneficial use.
In the in vitro studies of DCM fraction on isolated islets, there was a significant and dose-dependent stimulation of insulin secretion as compared to 11.8 mM glucose control. The maximum stimulation was with 10 µg DCM fraction/ml which was almost equal to glibenclamide (10 µg/ml) proving its insulin secretagogue action. The finding that the DCM fraction stimulates a 2.80-fold increase in insulin secretion at basal glucose concentration, viz., 2.8 mM, suggested that Kalanchoe pinnata DCM fraction can stimulate insulin production in a glucose independent manner.
To illustrate the probable pathway for insulin stimulatory action of Kalanchoe pinnata, its effect in the absence of glucose, its dependency on K+-ATP channel and Ca2+ channel was assessed. Insulin secretagogues can stimulate insulin secretion in a number of ways. The K+-ATP channel plays a pivotal role in insulin secretion (Ashcroft & Gribble, Citation1999). Insulin secretagogues like glucose or sulfonylurea leads to insulin secretion by closure of the K+-ATP channel that leads to a series of events like depolarization of cell membrane and increase in intracellular Ca2+ concentration through voltage-dependent Ca2+ channels. The increased [Ca2+]i ultimately leads to exocytosis of insulin (Bratanova-Tochkova et al., Citation2002). The insulin stimulatory effect of glucose and sulfonylureas is abolished by diazoxide, which is known to prevent closure of the K+-ATP channel (Dunne & Petersen, Citation1991; Henquin et al., Citation1982). In the present study, diazoxide (150 µM) was found to abolish the insulin stimulatory action of Kalanchoe pinnata DCM fraction. Hence, the insulin stimulatory action of Kalanchoe pinnata DCM fraction may be due to closure of K+-ATP channels.
Increase in [Ca2+]i, whether by influx of extracellular Ca2+ or by release of Ca2+ from intracellular stores, plays an important role in insulin secretion (Henquin, Citation2004; Tengholm & Gylfe, Citation2009). To investigate whether the insulin stimulating effect of Kalanchoe pinnata was dependent on extracellular Ca2+, islets were incubated with nifedipine and 11.8 mM glucose. Nifedipine is a L-type Ca2+ channel blocker which prevents the influx of extracellular Ca2+ and hence abolishes insulin secretion (Giugliano et al., Citation1980). It was observed that nifedipine was not able to completely abolish the stimulatory effect of the Kalanchoe pinnata DCM fraction. This finding is intriguing as, if the DCM fraction can enhance the intracellular Ca+2 concentrations independently, it should be able to overcome the diazoxide block. There is a need of further detailed experimentation to understand this novel mechanism. Plant extracts such as Ocimum sanctum Linn. (Lamiaceae) leaf extract, having a similar type of insulin stimulating mechanism, have been reported (Hannan et al., Citation2006).
Conclusion
The traditional medicine system has a wealth of components with potential bioactivity in the treatment of diabetes. However, lack of understanding of the precise mechanism and identification of the bioactive constituent precludes their use in modern science. In the present study, we have been able to evaluate the antidiabetic action of Kalanchoe pinnata through in vivo and in vitro studies. The DCM fraction of the plant demonstrated glucose independent insulin secretagogue action similar to the currently used drug glibenclamide and hence needs to be administered just prior to meals as in case of glibenclamide. Hence, we report for the first time the presence of a phenyl alkyl ether derivative isolated from the Kalanchoe pinnata leaves distillate possessing potent insulin secretagogue action. This action has the potential for development of new lead molecules for the treatment of diabetes.
Declaration of interest
The authors report no declarations of interest. Dr. Swapnil Patil acknowledges University Grant Commission (UGC), New Delhi, India for the fellowship under the Special Assistance Program for Basic Scientific Research (BSR).
Supplementary Material
Download PDF (66.8 KB)References
- Ashcroft FM, Gribble FM. (1999). ATP-sensitive K+ channels and insulin secretion: Their role in health and disease. Diabetologia 42:903–19
- Bergmann BR, Costa SS, Borges MBS, et al. (1994). Immunosuppressive effect of the aqueous extract of Kalanchoe pinnata in mice. Phytotherapy Res 8:399–402
- Bratanova-Tochkova TK, Cheng H, Daniel S, et al. (2002). Triggering and augmentation mechanisms, granule pools, and biphasic insulin secretion. Diabetes 51:S83–90
- Dunne MJ, Petersen OH. (1991). Potassium selective ion channels in insulin-secreting cells: Physiology, pharmacology and their role in stimulus secretion coupling. Biochim Biophys Acta 1071:67–82
- Efanova IB, Zaitsev SV, Zhivotovsky B, et al. (1998). Glucose and tolbutamide induce apoptosis in pancreatic beta-cells: A process dependent on intracellular Ca2+ concentration. J Biol Chem 273:33501–7
- Geof S, Volmer PA. (2004). Kalanchoe species poisoning in pets. Vet Med 99:933–6
- Giugliano D, Torella R, Cacciapuoti F, et al. (1980). Impairment of insulin secretion in man by nifedipine. Eur J Clin Pharmacol 18:395–8
- Hannan JM, Marenah L, Ali L, et al. (2006). Ocimum sanctum leaf extracts stimulate insulin secretion from perfused pancreas, isolated islets and clonal pancreatic β-cells. J Endocrinol 189:127–36
- Henquin JC. (2004). Pathways in β-cell stimulus-secretion coupling as targets for therapeutic insulin secretagogues. Diabetes 53:S48–58
- Henquin JC, Charles S, Nenquin M, et al. (1982). Diazoxide and D600 inhibition of insulin release: Distinct mechanisms explain the specificity for different stimuli. Diabetes 31:776–83
- Kamgang R, Mboumi RY, Fondjo AF, et al. (2008). Antihyperglycaemic potential of the water-ethanol extract of Kalanchoe crenata (Crassulaceae). J Nat Med 62:34–40
- Kimmel B, Inzucchi SE. (2005). Oral agents for type 2 diabetes: An update. Clin Diabetes 23:64–76
- Krenn L, Kopp B. (1998). Bufadienolides from animal plant sources. Phytochemistry, 48:1–29
- Kulkarni CR, Joglekar MM, Patil SB, Arvindekar AU. (2012). Antihyperglycemic and antihyperlipidemic effect of Santalum album in streptozotocin induced diabetic rats. Pharm Biol 50:360–5
- Lalau JD, Race JM. (1999). Lactic acidosis in metformin therapy. Drugs 58:55–60, discussion 75–82
- Latha M, Pari L, Ramkumar KM, et al. (2009). Antidiabetic effects of scoparic acid D isolated from Scoparia dulcis in rats with streptozotocin-induced diabetes. Nat Prod Res 23:1528–40
- McKenzie RA, Dunster PJ. (1986). Hearts and flowers: Bryophyllum poisoning of cattle. Aust Vet J 63:222–7
- Mosman T. (1983). Rapid colorimetric assay for cellular growth and survival: Application to proliferation and cytotoxicity assays. J Immunol Methods 65:55–63
- Ogbonnia SO, Odimegwu JI, Enwuru VN. (2008). Evaluation of hypoglycaemic and hypolipidaemic effects of aqueous ethanolic extracts of Treculia africana Decne and Bryophyllum pinnatum Lam. and their mixture on streptozotocin (STZ)-induced diabetic rats. Afr J Biotechnol 7:2535–9
- Ojewole JAO. (2005). Antinociceptive, anti-inflammatory and antidiabetic effects of Bryophyllum pinnatum (Crassulaceae) leaf aqueous extract. J Ethnopharmacol 99:13–19
- Patil SB, Ghadyale VA, Taklikar SS, et al. (2011). Insulin secretagogue, alpha-glucosidase and antioxidant activity of some selected spices in streptozotocin-induced diabetic rats. Plant Foods Hum Nutr 66:85–90
- Stetten MR, Katzen HM. (1961). Degradation of glycogen by alkali. J Am Chem Soc 83:2912–18
- Supratman U, Fujita T, Akiyama K, et al. (2001). Anti-tumor promoting activity of bufadienolides from Kalanchoe pinnata and K. daigremontianax tubiflora. Biosci Biotechnol Biochem 65:947–9
- Swanston-Flatt SK, Flatt PR, Day C, Bailey CJ. (1991). Traditional dietary adjuncts for the treatment of diabetes mellitus. Proc Nutr Soc 50:641–51
- Tengholm A, Gylfe E. (2009). Oscillatory control of insulin secretion. Mol Cell Endocrinol 297:58–72
- Torres-Santos EC, Da-Silva SAG, Costa SS, et al. (2003). Toxicological analysis and effectiveness of oral Kalanchoe pinnata on a human case of cutaneous leishmaniasis. Phytother Res 17:801–3
- WHO Expert Committee on Diabetes Mellitus (Second Report). (1980). Geneva, World Health Organization
- World Health Organization. (1999). Definition, diagnosis and classification of diabetes mellitus and its complications: Report of a WHO consultation. Part 1: Diagnosis and classification of diabetes mellitus. Geneva, World Health Organization
- Yadav NP, Dixit VK. (2003). Hepatoprotective activity of leaves of Kalanchoe pinnata Pers. J Ethnopharmacol 86:197–202