Abstract
Context: Due to several limitations of existing cyanide antidotes, α-ketoglutarate (α-KG) has been proposed as a promising treatment for cyanide.
Objective: This study reports the accelerated stability and bioassay of a new oral α-KG formulation.
Materials and methods: Amber-colored PVDF bottles containing 100 ml of 10% α-KG in 70% sorbitol, preservative (sodium methyl paraben and sodium propyl paraben), sweetener (sodium saccharine), flavor (American ice-cream soda and peppermint) and color (tartrazine), at pH 7.0–8.0 were stored in stability chamber (40 ± 2 °C and 75 ± 5% humidity) for 6 months in a GMP compliant facility. Various physical (pH, color, evaporation, extractable volume and clarity), chemical (identification and quantification of active ingredient) and microbiological (total aerobic count) analyses, together with protection studies were carried periodically in mice. Acute toxicity of the formulation and bioavailability of α-KG were assessed in rats at the beginning of the experiment.
Results: No physical changes and microbiological growth were observed in the formulation. After 6 months, α-KG content in the formulation diminished by ∼24% but its protective efficacy against cyanide remained at 5.9-fold. Protection was further characterized spectrophotometrically by disappearance of α-KG spectrum in the presence of cyanide, confirming cyanohydrin formation. Oral LD50 of α-KG formulation in rats was >7.0 g/kg body weight, and did not produce any acute toxicity of clinical significance. Also, an appreciable amount of α-KG was measured in blood.
Conclusion: As per the guidelines of International Conference on Harmonization, the new α-KG formulation exhibited satisfactory stability, bioefficacy and safety as cyanide antidote.
Introduction
Cyanide is a fast-acting poison, which causes intracellular hypoxia by reversibly binding to mitochondrial cytochrome c oxidase (CCO). Information on acute cyanide poisoning from intentional and accidental exposures, causing significant morbidity and mortality, dates back to antiquity (Baskin et al., Citation2004; Gracia & Shepherd, Citation2004). Intentional exposure of humans is usually experienced during suicides, homicides, attempted genocides and military use (Eckstein, Citation2004). Inadvertent cyanide exposures usually occur during inhalation of fire smoke, occupational and household accidents, and industrial disasters (Hall et al., Citation2009). Possible threat of cyanide being used by terrorists has generated renewed interest in its diagnosis and treatment (Baud, Citation2007). Life-threatening through cyanide poisoning is treatable when quickly recognized, and immediately and aggressively counteracted with an appropriate antidote (Hamel, Citation2011). Unlike poisons for which no specific antidote is available, cyanide has numerous antidotes with diverse mechanisms of action and safety profiles (Gracia & Shepherd, Citation2004; Mégarbane et al., Citation2003). Inhalation of amyl nitrite followed by intravenous injection of sodium nitrite and sodium thiosulfate is the treatment of choice for cyanide poisoning. Other popular treatments include 4-dimethylaminophenol, dicobalt edetate and hydroxocobalamin (Bhattacharya, Citation2000). Due to several limitations of these antidotes, there is a lack of consensus in the international medical community on the risk–benefit ratio of cyanide antidotes (Cummings, Citation2004; Hall et al., Citation2009; Van Heijst et al., Citation1987). Except amyl nitrite, all the antidotes have to be administered intravenously, which is discouraged during pre-hospital mass casualty management following cyanide exposure (Lavon & Bentur, Citation2010).
Extensive research efforts have been expended to develop α-ketoglutarate (α-KG) as an effective oral treatment for cyanide poisoning (Bhattacharya, Citation2004; Bhattacharya & Tulsawani, Citation2008; Bhattacharya & Vijayaraghavan, Citation2002; Bhattacharya et al., Citation2002; Tulsawani et al., Citation2005). Cyanide detoxification by α-KG has been attributed to interaction of its carbonyl moiety with electrophilic cyanide ion to form cyanohydrin complex (Norris et al., Citation1990). Previous studies showed that oral administration of 2.0 g/kg α-KG afforded 5- to 6-fold protection against acute cyanide poisoning in rodents (Bhattacharya & Vijayaraghavan, Citation2002; Bhattacharya et al., Citation2002). Although, 2.0 g/kg α-KG was non-toxic in rodents, the dose was enormous for human consumption (Bhattacharya et al., Citation2001). During clinical trials in human volunteers, the dose of α-KG was reduced to two split doses of 10 g each followed by hydration. This improved the bioavailability and safety margin of α-KG. However, this aqueous solution of α-KG was salty in taste and caused nausea (Mittal et al., Citation2010). Now, we were interested to prepare a palatable and stable formulation of α-KG, amenable for human consumption. This manuscript addresses the preparation of a new oral α-KG formulation and its accelerated stability and bioassay for 6 months, as per the ICH (International Conference on Harmonization) guidelines (OGD, Citation2012; WHO, Citation2009). The formulation could be used for the treatment of cyanide poisoning resulting from closed-space fire, industrial accidents, chemical terrorism and ingestion of cyanide and its derivatives. Additionally, the product could be instituted as an energy-rich nutritional supplement or sports medicine.
Materials and methods
Animals
Male Swiss albino mice (22–25 g) and female Wistar rats (120–140 g) bred in the animal facility of Defence Research and Development Establishment (DRDE), Gwalior, were used in the study. The animals were kept in conditions consistent with requirements of institutional ethical committee on animal experimentations. They were maintained in controlled environmental conditions of ambient temperature (22 ± 2 °C) and relative humidity of 40–60%, in a 12:12 light:dark cycle. All the animals were fed standard pellet diet and water ad libitum. The animals were acclimatized for 7 days and fasted overnight prior to the experiment. The experimental protocol was approved by the Animal Ethics Committee of DRDE (No. ET-03/49/RB dated 7 July 2011).
Chemicals
Potassium cyanide (KCN) was purchased from Ferrack (Darmstaat, Germany), and α-ketoglutaric acid disodium salt (α-KG) and other chemicals were purchased from Sigma-Aldrich (St. Louis, MO).
Preparation and storage of new α-KG formulation
The α-KG formulation, on the basis of % (w/v) contained 10% α-KG (7.5–11%), 70% sorbitol (30–80%) as base, sodium methyl paraben (0.08–0.13%) and sodium propyl paraben (0.08–0.13%) as preservatives, sodium saccharine (0.02–0.06%) as sweetener, American ice-cream soda (0.04–0.1%) and peppermint (0.06–0.13%) as flavor, tartrazine (0.004–0.007%) as color and purified water (q.s.). The formulation (pH 7–8) was filled in amber-colored PVDF (polyvinylidene fluoride) bottles and placed in stability chamber (Thermo Fischer) at 40 ± 2 °C and 75 ± 5% RH (relative humidity) for 6 months in a GMP compliant facility. Various physical (pH, color, evaporation, extractable volume and clarity), chemical (identification and quantification of active ingredient) and microbiological (total aerobic count) analyses, together with protection studies were carried periodically in mice. At the start of the experiment (“0” month), acute toxicity (clinical hematology and biochemistry) of the formulation and bioavailability of α-KG were also assessed in rats.
Physical and chemical analysis
The color, clarity, pH, evaporation volume and extractable volume were measured every month by standard procedures (WHO, Citation2009). In the same aliquots, α-KG was measured by a UV-spectrophotometric method (Von Korff, Citation1969). This method involves conversion of α-KG to glutamate in the presence of nicotinamide–adenine dinucleotide (NADH) and NH4+, a reaction catalyzed by glutamate dehydrogenase (GDH). One mole NADH is oxidized for each mole of α-KG. The decrease in NADH concentration as measured by the change in absorbance at 340 nm is proportional to the amount of α-KG, which is expressed in mg/ml. In a separate study, absorbance of α-KG in 0.1 M sodium phosphate buffer (pH 7.4) was recorded at 240–400 nm. Thereafter, α-KG was mixed with cyanide in 1:1 molar ratio and the disappearance of α-KG peak was observed, which confirmed the interaction of α-KG and cyanide to produce cyanohydrin complex (Norris et al., Citation1990).
Microbiological analysis
Total aerobic count was performed by the plate count method and filtration method (Indian Pharmacopoeia, Citation2007). α-KG syrup (100 ml) was dilute with 900 ml sterile peptone water (stock sample). Further, 10-ml stock sample was diluted with 90 ml sterile peptone water (working sample). Thereafter, 1 ml working sample was aseptically transferred to two sterile Petri dishes (90 mm) containing 20 ml soybean casein digest agar medium. Temperature of the media was set at 45 °C. The plates were agitated and left for the medium to solidify. The plates were incubated at 22 ± 2 °C for 72 h and further at 32 ± 2 °C for 48–72 h. At the end of incubation period, total microbial counts were visually enumerated. The arithmetic mean of the counts was considered to calculate the number of colonies formed/unit/ml. For the microbial analysis by filtration method, 100 ml of α-KG syrup was aseptically transferred on a sterile membrane filtration unit containing sterile filter paper of 0.45 µ pore size. After filtration, the filter paper was washed with 100 ml of sterile 0.1% peptone solution. The filter paper was aseptically transferred to Petri dishes containing nutrient agar. The plates were incubated at 22 ± 2 °C for 72 h and further at 32 ± 2° for 48 h. At the end of incubation period total microbial colonies were counted.
Protective efficacy
At “0” month, the LD50 of potassium cyanide (KCN; oral) was determined in mice, in the presence or absence of simultaneous treatment (0 min) or pre-treatment (−10 min) of α-KG given at 0.15 g/kg (low dose), 0.30 g/kg (middle dose), 0.60 g/kg (high dose) and 2.0 g/kg (protective dose) body weight, following Dixon’s (Citation1965) up and down method. Here, 2.0 g/kg α-KG is referred as the protective dose because it conferred maximum protection against cyanide in experimental animals (Bhattacharya & Vijayaraghavan, Citation2002; Bhattacharya et al., Citation2002). For each LD50, five to six animals were used and the mortality was recorded up to 24 h. Both KCN and α-KG syrup was administered orally using a 16 gauge animal feeding needle (HSE-Harvard, March-Hugstetten, Germany). The protection index (PI) was calculated as the ratio of LD50 of KCN in the presence of α-KG (protected animals) and LD50 of KCN in the absence of α-KG (unprotected animals). Thereafter, every month the aliquots of α-KG syrup kept in the stability chamber were drawn for the determination of PI of α-KG (2.0 g/kg; 0 min) against KCN up to 6 months.
Toxicity assay
At “0” month, α-KG syrup was administered orally in rats. Animals were divided into four groups of six rats each as follows: (i) control, (ii) 0.15 g/kg α-KG, (iii) 0.30 g/kg α-KG and (iv) 0.60 g/kg α-KG. After 1 h, 24 h and 7 days of exposure, rats were anesthetized and blood was collected from retro orbital plexus using heparinized capillary for various biochemical assays like aspartate aminotransferase (AST; U/L), alanine aminotransferase (ALT; U/L), lactate dehydrogenase (LDH; U/L), cholesterol (mg/dl), glucose (mg/dl), creatinine (mg/dl), protein (mg/dl), urea (mg/dl) and bilirubin (mg/dl). All the assays were performed using an Ecoline diagnostic kit (Merck, Darmstadt, Germany). Further, Na+ and K+ were measured by Roche OPTI CCA blood gas analyzer (Mannheim, Germany). Various hematological indices like white blood cells, red blood cells, hemoglobin, hematocrit, mean cell volume, mean cell hemoglobin, mean cell hemoglobin concentration and platelet were also measured using automated hematological analyzer (Sysmex K-4500, Holliston, MA). Pooled urine samples after 24 h and 7 days of treatment were examined for pH, nitrite proteins, glucose, ketones, urobilinogen, bilirubin and hemoglobin using a Combur-8-Test (Boehringer Mannheim, Mannheim, Germany). Seven days after treatment, animals were sacrificed and organ-body weight index (OBI) for different organs (brain, heart, lungs, liver, kidneys and spleen) was determined as the ratio of organ weight and animal body weight × 100. In a separate study, acute (24 h) oral LD50 of the α-KG formulation was determined using five to six rats (Dixon, Citation1965).
Bioavailability studies
At “0” month, α-KG syrup was administered orally in rats. Animals were divided into five groups of six rats each as follows: (i) control, (ii) 0.15 g/kg α-KG, (iii) 0.30 g/kg α-KG, (iv) 0.60 g/kg α-KG and (v) 2.0 g/kg α-KG. Blood α-KG levels were measured at different time points by the method of Von Korff (Citation1969).
Statistics
All the values are expressed as mean ± SE of three replicates or six animals. The statistical analysis was performed using one-way ANOVA followed by the Student–Newman–Keuls multiple comparison test, using SigmaStat software (SSP Inc., Lansdowne, VA). Statistical significance was drawn at p < 0.05.
Results
enumerates the data on various physical, chemical and microbiological assays carried out during 6 months accelerated the stability analysis of α-KG formulation. Throughout the study, the color of the solutions remained yellow and clear, and the pH was between 7.7 and 7.8. Also, there was no evaporation of the formulation and the extractable volume remained constant. Quantitative estimation showed that α-KG content in the formulation progressively declined from 109.8 ± 0.50 mg/ml at “0” month to 83.6 ± 0.20 mg/ml at 6 months, amounting to ∼24% decrease. The data also indicate that there were no microbiological contaminations or growth related turbidity in the formulation during the stability analysis. Further, the ultraviolet spectrum of α-KG was obtained at 317 nm in the presence or absence of equimolar cyanide. Cyanide did not show any absorbance at 240–400 nm. However, when cyanide was added to α-KG in 1:1 ratio, the peak of α-KG spectrum was diminished, and two absorbance peaks appeared at 288.5 and 293 nm, indicating cyanohydrin formation ().
Figure 1. Accelerated stability analysis of α-ketoglutarate (α-KG) formulation was carried out for a period of 6 months. At the beginning of the experiment (“0” month), absorbance of α-KG was recorded at 240–400 nm. The UV spectrum of α-KG was recorded at 317 nm, while cyanide did not show any absorbance at 240–400 nm. Thereafter, cyanide and α-KG were mixed in 1:1 ratio and the disappearance of α-KG peak was observed, which confirmed the interaction of α-KG and cyanide to produce cyanohydrin complex.
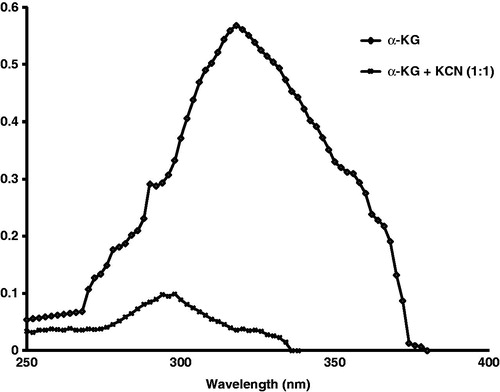
Table 1. Various physical, chemical and microbiological assays carried out during accelerated stability analysis of α-KG formulation.
At “0” month, protective efficacy of simultaneous treatment (0 min) or pre-treatment (−10 min) of α-KG given at 0.15 g/kg (low dose), 0.30 g/kg (medium dose), 0.60 g/kg (high dose) and 2.0 g/kg (protective dose) body weight, was determined against acute cyanide intoxication in mice. shows that 0.15 g/kg A-KG afforded a 2.96-fold protection against cyanide when given simultaneously or as 10-min pre-treatment. When the dose was increased to 0.30 g/kg, the protection further increased to 5.91-fold. However, further increase in the dose of α-KG (0.60 g/kg) did not yield any additional protection. On the other hand, pre-treatment of 2.0 g/kg α-KG resulted in maximum protection of 11.78-fold as against 5.91-fold when administered simultaneously. Subsequently, protective effects of 2.0 g/kg α-KG administered simultaneously (0 min) was assessed every month during 6 months of accelerated stability analysis. At “0” month, α-KG conferred 5.91-fold protection, which remained constant untill the termination of experiment after 6 months (data not shown).
Table 2. Protective efficacy of different doses of α-KG formulation against acute cyanide intoxication in mice.
Acute toxicity of the α-KG formulation was determined in rats at “0” month, and its 24 h oral LD50 was found to be 7.38 (2.98–18.29) g/kg body weight. Thereafter, different doses (0.15, 0.30 and 0.60 g/kg) of α-KG were administered in rats, and various hematological, urinary and biochemical parameters were measured 1 h, 24 h and 7-day post-exposure. All the doses of α-KG caused significant increase in blood glucose levels after 1 h, but no other significant changes were observed in the remaining parameters. Also, none of the doses of α-KG caused any significant change in animal body weight or OBI of brain, heart, lungs, liver, kidneys and spleen when observed 7-day post-exposure (data not shown). illustrates the blood α-KG levels at various time intervals after oral administration of different doses of α-KG in rats. A significant dose-dependent increase in blood α-KG levels was observed at all the time intervals and the maximum α-KG levels were observed at 30-min post-exposure, which started declining thereafter. At this point of time, blood α-KG level in 2.0 g/kg dose was almost 3- to 4-fold higher as compared to corresponding 0.15–0.60 g/kg doses of α-KG. At 60-min post-exposure also, the levels of blood α-KG after 0.30, 0.60 and 2.0 g/kg α-KG, remained significantly elevated as compared to the corresponding control.
Figure 2. Accelerated stability analysis of α-ketoglutarate (α-KG) formulation was carried out for a period of 6 months. At the beginning of the experiment (“0” month), female rats were administered α-KG (oral) formulation at 0.15 g/kg (low dose), 0.30 g/kg (medium dose), 0.60 g/kg (high dose) and 2.0 g/kg (protective dose). Thereafter, blood α-KG levels were measured at various time intervals. Values are mean ± SE of six animals. *Significantly different from corresponding control at p < 0.05.
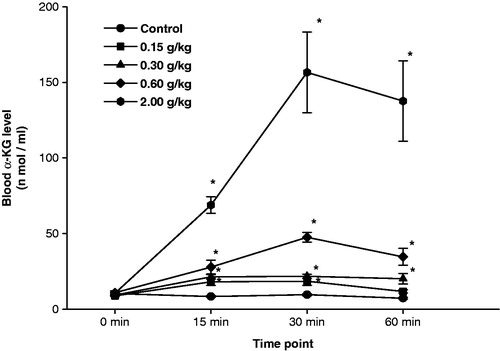
Discussion
Treatments for cyanide poisoning are many, which should be instituted immediately and aggressively for optimal results (Baud, Citation2007; Bhattacharya & Flora, Citation2009; Rotenberg, Citation2003). However, most of the treatments suffer from serious limitations (Bhattacharya, Citation2000; Van Heijst et al., Citation1987). Except amyl nitrite, all the antidotes are discouraged as self-aid or first aid during out-of-hospital mass casualty management (Lavon & Bentur, Citation2010). This necessitated the evaluation of new sulfur containing molecules as oral antidote for cyanide poisoning (Nagasawa et al., Citation2007). Alternatively, oral treatment of α-KG was also found to be effective in challenging experimental cyanide and cyanogen poisoning (Bhattacharya, Citation2004; Bhattacharya et al., Citation2009). Besides chemical detoxification of cyanide, beneficial effects of α-KG have also been harnessed as sport medicine and nutritional supplement. It is sold over the counter to treat metabolic disorders and increase stamina. It has been used as a nitrogen transporter for detoxification of excessive ammonia and other nitrogenous substances in the body (Satpute et al., Citation2012). Also, α-KG is clinically used in post-operative care as a source of glutamine, which is required for protein synthesis and rapid regeneration of cells (Cynober, Citation1991, Citation1999; Le Boucher et al., Citation1998; Pangborn et al., Citation1990). Previous animal studies revealed that, maximum antidotal potency of α-KG as cyanide antidote was achieved at 2.0 g/kg dose (Bhattacharya & Vijayaraghavan, Citation2002; Bhattacharya et al., Citation2002). However, clinical trial recommended two split doses of 10 g each of α-KG as the tolerable dose in human (Mittal et al., Citation2010). In order to make this α-KG formulation palatable and stable, the present investigation was taken up.
During our preliminary study in mice (data not shown), we observed that single dose of (i) 6.3% α-KG syrup, equivalent to 90 mg/kg α-KG for a 65–70 kg man conferred a PI of 1.48, accompanied by 18% degradation during the expected shelf life (6 months accelerated condition), (ii) 7.5–11% α-KG syrup, equivalent to 107–150 mg/kg α-KG conferred a PI of 2.96, accompanied by 17% degradation, and (iii) 14% α-KG syrup, equivalent to 200 mg/kg α-KG conferred a PI of 2.96, accompanied by 18% degradation. The data revealed that (i) although, chemical stability in all the doses was comparable, syrup containing <7.5% α-KG did not afford appreciable protection, and (ii) 7.5–11% and 14% α-KG syrup exhibited similar protection and stability. Therefore, in the absence of additional protection with 14% α-KG syrup, we narrowed down to 10% α-KG (7.5–11%) for further studies. Thereby, a significant reduction in α-KG dose could be achieved as compared to previous studies (Bhattacharya & Vijayaraghavan, Citation2002; Bhattacharya et al., Citation2002).
During our previous stability study, 40% aqueous solution of α-KG without any preservative, sweetener, flavor and color was used. The clear solution (pH 8.45–8.91) was filled in white HDPE (high-density polyethylene) bottles and stored at room temperature or refrigerator for 2 years. The α-KG solution stored at room temperature showed yellowish coloration after 6 months, accompanied by marginal decline in protective efficacy against cyanide. However, the refrigerated solution exhibited appreciable stability (Bhattacharya et al., Citation2007). During the clinical trial in human volunteers, the aqueous solution of α-KG was found to be salty in taste and unpalatable, and caused severe nausea (Mittal et al., Citation2010). The study recommended reduction in α-KG saturation, to facilitate gastric emptying and enhance bioavailability. Thereafter, we prepared a 10% α-KG syrup in sorbitol base with permitted preservatives, sweetener, flavor and color (erythrosine). However, the color was not found to be stable. Subsequently, we used tartrazine as a coloring agent, which was found to be much stable at 7–8 pH of the syrup. Also, five human subjects in the laboratory tasted the syrup and found it to be quite palatable without any allergic reaction or nausea. To prevent any photodegradation, we now filled the syrup in colored PVDF bottle. Earlier, we had carried out 2-year stability test with aqueous solution of α-KG, stored at room temperature or refrigerated conditions (Bhattacharya et al., Citation2007). As per ICH guidelines, accelerated stability study for 6 months with the new drug formulation was sufficient in lieu of long-term stability study (OGD, Citation2012). Therefore, accelerated stability tests for 6 months were taken up with the new oral α-KG formulation, which did not reveal any physical, chemical and microbiological abnormalities. Also, qualitative and quantitative measurements ascertained the presence of α-KG in the syrup, and its interaction with cyanide. However, α-KG content in the syrup decreased by 24% after 6 months, without any concomitant decrease in its protective efficacy. Aqueous solution of α-keto acids are known to be very unstable due to tautomerism. Previous NMR studies showed that even in the freshly prepared solution, chemical content of α-KG diminished by 12%. Tautomerism between keto and enol group sets in immediately in solution, which can be characterized more accurately by advanced techniques like GC-MS, LC-MS, NMR and FTIR (Bhattacharya et al., Citation2007; Von Korff, Citation1969). However, among other keto acids, α-KG is known to be more stable. Also, base-catalyzed polymerization of α-KG is not known as observed with other keto acids (Varma & Hegde, Citation2004). In spite of the tautomerism, the functional carbonyl group of α-KG is always available to neutralize the cyanide ion.
In our previous study, aqueous solution of 0.125, 0.25 and 2.0 g/kg α-KG administered simultaneously (0 min) produced a PI of 1.4, 1.9 and 5.4, respectively, whereas pre-treatment (−10 min) of the same produced a PI of 1.3, 1.9 and 7.6, respectively (Bhattacharya et al., Citation2007). If we compare the dose-dependent protective efficacy of previous α-KG with the present formulation, the efficacy of the syrup was found to improve. In the present study no progressive decline in protection was observed during 6 months stability analysis, which otherwise diminished in our previous study (Bhattacharya et al., Citation2007). Several carbonyl compounds and their metabolites or nutrients including glucose, sucrose, fructose, mannitol, sorbitol, etc., have earlier been shown to antagonize experimental cyanide poisoning by mechanism similar to α-KG (Niknahad et al., Citation1994). Similar to these compounds, α-KG is also known to have antioxidant properties, which is not compromised in the aqueous solution (Andrae et al., Citation1985; Cynober, Citation1991, Citation1999; Eghbal et al., Citation2004; Varma & Hegde, Citation2004). Further, protective efficacy of α-KG is also facilitated by its integration into the malate–aspartate shuttle operative between the cytoplasm and the mitochondrial matrix, leading to ATP generation (Varma & Hegde, Citation2004). Role of α-KG as a nutritional supplement to bolster the citric acid cycle is well known (Pangborn et al., Citation1990). The GC-MS profile of α-KG showed traces of succinic acid after long storage (Bhattacharya et al., Citation2007). Succinic acid is also known to provide marginal protection against cyanide (Bhattacharya & Tulsawani, Citation2008). Although, in the present study, α-KG content by chemical analysis was found to fall by 24% under accelerated stability condition, the consistent protective efficacy of the formulation can be attributed to other mechanisms as well, in addition to its chemical interaction with cyanide. As a result, the protective efficacy of α-KG against cyanide is not exactly mirrored by its chemical degradation over a period of time.
Each bottle of 10% α-KG, if repeated after 10 min would deliver ∼0.30 g/kg α-KG for a 65–70 kg man. This dose produces a PI of 5.91, which is almost similar to that produced by 2.0 g/kg α-KG (Bhattacharya & Vijayaraghavan, Citation2002; Bhattacharya et al., Citation2002, Citation2007). In the present study, blood α-KG levels measured at 30 min after 0.15, 0.30 and 0.60 g/kg α-KG were 8.4-, 7.2- and 3.3-fold less, respectively, as compared to 2.0 g/kg α-KG. However, 13.33-, 6.66- and 3.33-fold decrease in α-KG dose, respectively, could be achieved without any concomitant decrease in protective efficacy. Previous study in rats showed that 2.0 g/kg α-KG produced a plasma Cmax of 36.9 µg/ml α-KG only, indicating that bioavailability of oral α-KG did not commensurate with the dose (Patra, Citation2005). Intestinal absorption of α-KG in pigs was reported to be very limited, possibly due to mucosal metabolism and substantial first-pass gastrointestinal metabolism (Buddington et al., Citation2004; Lambert et al., Citation2006). We also observed considerable gastric retention in animals and humans after oral administration of highly concentrated α-KG, but significant plasma α-KG levels could be achieved by ∼7-fold dilution of α-KG (Mittal et al., Citation2010). In the present study also, the PI obtained after simultaneous treatment of 0.30, 0.60 and 2.0 g/kg α-KG was identical. This indicates that at lower doses, absorption of α-KG given prophylactically or simultaneously was almost identical. Nevertheless, pre-treatment of 2.0 g/kg α-KG resulted in enhanced bioavailability and protection thereby. Sorbitol as base is known to enhance the bio-efficacy and stability of drug formulations (O’Brien Nabors, Citation2011). This possibly could also improve the stability and bioavailability of the present α-KG syrup.
Data on acute toxicity of α-KG formulation did not reveal any alterations of clinical significance. Transient increase in blood glucose, 1 h after α-KG treatment could be due to sorbitol. We confirmed this phenomenon after administering an equivalent amount of sorbitol in rats (data not shown). Oral LD50 of the present formulation was found to be >5.0 g/kg body weight, which was consistent with our previous observation with aqueous α-KG solution (Bhattacharya et al., Citation2001). If the safety margin is to be calculated by dividing the LD50 (7.38 g/kg) of α-KG by its effective dose (0.30 g/kg), it works out to ∼25-folds. This indicates that the new α-KG formulation is quite safe for human consumption.
Conclusion
The present study reveals a new palatable, effective and non-toxic formulation of α-KG that could withstand accelerated stability parameters. The study revealed that, low blood α-KG levels were defied by high protective efficacy of the syrup even after 6 months of accelerated conditions. As per ICH guidelines, the formulation can be considered stable at ambient temperature for 2 years (OGD, Citation2012; WHO, Citation2009). However, our future endeavour would be to further improve the chemical stability of α-KG in the formulation.
Declaration of interest
The authors report that there are no declarations of interest.
Acknowledgements
The authors thank Prof. (Dr.) M. P. Kaushik, Director and Dr. P. V. L. Rao, Head, Bioprocess and Scale up Facility of DRDE, Gwalior, India for their support and encouragement.
References
- Andrae U, Singh J, Zeigler-Skylakakis K. (1985). Pyruvate and related alpha-ketoacids protect mammalian cells in culture against hydrogen peroxide-induced cytotoxicity. Toxicol Lett 28:93–8
- Baskin SI, Petrikovics I, Kurche JS, et al. (2004). Insights on cyanide toxicity and methods of treatment. In: Flora SJS, Romano JA, Baskin SI, Sekhar K, eds. Pharmacological Perspectives of Toxic Chemicals and their Antidotes. New Delhi: Narosa Publishing House, 105–46
- Baud FJ. (2007). Cyanide: Critical issues in diagnosis and treatment. Hum Exp Toxicol 26:191–201
- Bhattacharya R. (2000). Antidotes to cyanide poisoning: Present status. Indian J Pharmacol 32:94–101
- Bhattacharya R. (2004). α-Ketoglutarate: A promising antidote to cyanide poisoning. In: Flora SJS, Romano JA, Baskin SI, Sekhar K, eds. Pharmacological Perspectives of Toxic Chemicals and Their Antidotes. New Delhi: Narosa Publishing House, 411–30
- Bhattacharya R, Flora SJS. (2009). Cyanide toxicity and its treatment. In: Gupta RC, ed. Handbook of Toxicology of Chemical Warfare Agents. New York: Elsevier Inc, 255–70
- Bhattacharya R, Kumar D, Sugendran K, et al. (2001). Acute toxicity studies of α-ketoglutarate: A promising antidote for cyanide poisoning. J Appl Toxicol 21:495–9
- Bhattacharya R, Lakshmana Rao PV, Vijayaraghavan R. (2002). In vitro and in vivo attenuation of experimental cyanide poisoning by α-ketoglutarate. Toxicol Lett 128:185–95
- Bhattacharya R, Satpute RM, Hariharakrishnan J, et al. (2009). Acute toxicity of some synthetic cyanogens in rats and their response to oral treatment with alpha-ketoglutarate. Food Chem Toxicol 47:2314–20
- Bhattacharya R, Tulsawani RK. (2008). In vitro and in vivo evaluation of various carbonyl compounds against cyanide toxicity with particular reference to alpha-ketoglutaric acid. Drug Chem Toxicol 31:149–61
- Bhattacharya R, Tulsawani RK, Sharma M, et al. (2007). Temperature dependent stability of alpha-ketoglutarate: A promising cyanide antidote. J Cell Tissue Res 7:965–73
- Bhattacharya R, Vijayaraghavan R. (2002). Promising role of α-ketoglutarate in protecting the lethal effects of cyanide. Hum Exp Toxicol 21:297–303
- Buddington RK, Pajor A, Buddington KK, Pierzynowski S. (2004). Absorption of alpha-ketoglutarate by the gastrointestinal tract of pigs. Comp Biochem Physiol A Mol Integr Physiol 132:215–20
- Cummings TF. (2004). The treatment of cyanide poisoning. Occup Med 54:82–5
- Cynober L. (1991). Ornithine α-ketoglutarate in nutritional support. Nutrition 7:313–22
- Cynober LA. (1999). The use of α-ketoglutarate salts in clinical nutrition and metabolic care. Curr Opin Clin Nutr Metab Care 2:33–7
- Dixon WJ. (1965). The up-and-down method for small samples. J Am Stat Assoc 60:967–78
- Eckstein M. (2004). Cyanide as a chemical terrorism weapon. J Emerg Med Serv 29:S22–31
- Eghbal MA, Pennefather PS, O’Brien PJ. (2004). H2S cytotoxicity mechanism involves reactive oxygen species formation and mitochondrial depolarization. Toxicology 203:69–76
- Gracia R, Shepherd G. (2004). Cyanide poisoning and its treatment. Pharmacotherapy 24:1358–65
- Hall AH, Saiers J, Baud F. (2009). Which cyanide antidote? Crit Rev Toxicol 39:541–52
- Hamel J. (2011). A review of acute cyanide poisoning with a treatment update. Crit Care Nurse 31:72–81
- Indian Pharmacopoeia (2007). Biological Methods. Vol. 1. Indian Pharmacopoeia Commission, Ministry of Health and Family Welfare, Govt. of India, Ghaziabad, India, 35--44
- Lambert BD, Filip R, Stoll B, et al. (2006). First-pass metabolism limits the intestinal absorption of enteral alpha-ketoglutarate in young pigs. J Nutr 136:2779–84
- Lavon O, Bentur Y. (2010). Does amyl nitrite have role in the management of pre-hospital mass casualty cyanide poisoning? Clin Toxicol 48:477–84.
- Le Boucher J, Eureng, Cynober LA. (1998). Ornithine α-ketoglutarate: The puzzle. Nutrition 14:870–3
- Mégarbane B, Delahaye A, Goldgran-Tolédano D, Baud FJ. (2003). Antidotal treatment of cyanide poisoning. J Chin Med Assoc 66:193–203
- Mittal G, Singh T, Kumar N, et al. (2010). Radiolabeling and dose fixation study of oral alpha-ketoglutarate as a cyanide antidote in healthy human volunteers. Clin Toxicol 48:509–15
- Nagasawa HT, Goon DJ, Crankshaw DL, et al. (2007). Novel, orally effective cyanide antidotes. J Med Chem 50:6462–4
- Niknahad H, Khan S, Sood C, O’Brien PJ. (1994). Prevention of cyanide-induced cytotoxicity by nutrients in isolated rat hepatocytes. Toxicol Appl Pharmacol 128:271–9
- Norris JC, Utley WA, Hume AS. (1990). Mechanism of antagonising cyanide induced lethality by α-ketoglutaric acid. Toxicology 62:275–83
- O’Brien Nabors L. (2011). Sorbitol and mannitol. In: Lyn O’Brien Nabors, ed. Alternative Sweeteners. 4th ed. Boca Raton: CRC Press, 343–4
- OGD (2012). Guidance for industry ANDAs: Stability testing of drug substances and products. U.S. Department of Health and Human Services, Food and Drug Administration, Center for Drug Evaluation and Research (CDER), September 2012, OGD
- Pangborn JB, Hicks JT, Chambers JR, Shahani KM. (1990). A Monograph on Alpha-ketoglutaric Acid. Lisle, IL: Bionostics Inc
- Patra D. (2005). Serum kinetics study of α-ketoglutarate in Wistar rats following single oral formulation. Internal Research Report (JRF/RES/2005/1573) of Jai Research Foundation, Valvada (India), 1–27
- Rotenberg JS. (2003). Cyanide as a weapon of terror. Pediatr Ann 32:236–40
- Satpute RM, Hariharakrishnan J, Lomash V, et al. (2012). Oxidative stress and tissue pathology caused by sub-acute ammonium acetate exposure in rats and their response to treatments of alpha-ketoglutarate and N-acetyl cysteine. Toxicol Ind Health 2012. [Epub ahead of print]. DOI: 10.1177/0748233712448117
- Tulsawani RK, Debnath M, Pant SC, et al. (2005). Effect of sub-acute oral cyanide administration in rats: Protective efficacy of α-ketoglutarate and sodium thiosulfate. Chem Biol Interact 156:1–12
- Varma SD, Hegde KR. (2004). The effect of α-ketoglutarate against selenite cataract formation. Exp Eye Res 79:913–18
- Van Heijst ANP, Douze JMC, Van Kesteren RG, et al. (1987). Therapeutic problems in cyanide poisoning. Clin Toxicol 25:383–98
- Von Korff RW. (1969). Purity and stability of pyruvate and α-ketoglutarate. Methods Enzymol 13:519–21
- WHO (2009). ICH Guidelines. Stability testing of active pharmaceutical ingredients and finished pharmaceutical products, WHO Technical Report Series, No. 953